This article has been corrected. See "Erratum: Blood flow-improving activity of methyl jasmonate-treated adventitious roots of mountain ginseng" in Volume 34 on page 48.
Abstract
Ginsenosides from Panax ginseng are well known for their diverse pharmacological effects including antithrombotic activity. Since adventitious roots of mountain ginseng (ARMG) also contain various ginsenosides, blood flow-improving effects of the dried powder and extract of ARMG were investigated. Rats were orally administered with dried powder (PARMG) or ethanol extract (EARMG) of ARMG (125, 250 or 500 mg/kg) or aspirin (30 mg/kg, a reference control) for 3 weeks. Forty min after the final administration, carotid arterial thrombosis was induced by applying a 70% FeCl3-soaked filter paper outside the arterial wall for 5 min, and the blood flow was monitored with a laser Doppler probe. Both PARMG and EARMG delayed the FeCl3-induced arterial occlusion in a dose-dependent manner, doubling the occlusion time at high doses. In mechanism studies, a high concentration of EARMG inhibited platelet aggregation induced by collagen in vitro. In addition, EARMG improved the blood lipid profiles, decreasing triglyceride and cholesterol levels. Although additional action mechanisms remain to be clarified, it is suggested that ARMG containing high amount of ginsenosides such as Rg3 improves blood flow not only by inhibiting oxidative thrombosis, but also by modifying blood lipid profiles.
Today, cardiovascular diseases (CVD) due to hypertension, hyperlipidemia, and atherosclerosis are one of the largest contributors to global mortality, leading to death of 19.5 million people in 2016. According to the World Health Organization, it will be about 23.3 million deaths from CVD in 2030 [1]. As the lifestyle of modern society has changed, high-energy and fat-rich diet, stresses, crapulence, and reduced exercise are believed to cause increased cardiovascular risks by affecting lipoprotein metabolism, platelet aggregation ability, and vessel resistance. It is well established that the incidence of CVD is due to hyperlipidemia, a condition characterized by significant increases in total cholesterol (TC), triglycerides (TG), and low-density lipoproteins (LDL) as well as decrease in high-density lipoproteins (HDL) [23].
Aspirin is a widely used anti-thrombosis drug for preventing cardiovascular diseases. Low doses of aspirin have been shown to have a preventive effect on thrombus formation in animal experiments [4]. But aspirin is acidic and stimulates stomach to release gastric acid. Besides, it affects gastric wall to become weak and causes gastric hemorrhage. In addition, currently available anti-thrombotic agents such as tissue-type plasminogen activator (t-PA), streptokinase, and PA-type agents for clinical research have some adverse-effects [5]. Therefore, safe and effective anti-thrombotic agents that can inhibit thrombosis are needed to be studied. Medicinal plants are the attractive source of pharmacologically active compounds and agents.
Panax ginseng C.A. Meyer is well known as the most important botanical medicine in traditional eastern Asia for longer than 2000 years, and now one of the most extensively used herbal products in the world [67]. The root of Panax ginseng has long been studied for its pharmacological efficacies [8]. In Korea, mountain ginseng, wild Panax ginseng which lives in mountain naturally, has been considered traditionally more effective than cultivated ginseng products [9]. This plants grow wild in cool, shady forests from Korea and north eastern China to far eastern Siberia [81011]. Mountain ginseng has become extremely scarce and the ginseng supply depends almost solely on field cultivation, which is a time-consuming and labor-intensive process. However, tissue culture techniques for mountain ginseng have been established by bioreactor technology as a useful tool for large-scale production of root biomass [1213]. Tissue culture techniques allowed us to easily obtain a mass of adventitious roots.
Tissue-cultured mountain ginseng was produced to contain higher concentration of bioactive ginsenosides or polyphenols than cultivated ginseng; these compounds are believed to be beneficial to human health [14]. The major active ingredients of ginseng are the triterpene glycosides named ginsenosides, which have been studied extensively for their pharmacological effects. These saponins possess diverse pharmacological actions for treating various diseases including cerebral ischemia [15], hypertension [16], and symptoms of hyperlipidemia [17], in addition to anti-fibrotic and antioxidant activities [1819], which are associated with circulatory system or others as well. Polyphenols rich in mountain ginseng defend tissues from radical damage and have anticholesterol, intestinal regulatory, anti-cancer, and antioxidant effects [20].
Based on the reported biological effects, we suggested that the adventitious root of mountain ginseng (ARMG) would influence physiological hemostatic and pathological thrombotic processes. The present study was designed to investigate whether dried powder (PARMG) and an extract (EARMG) of ARMG have potential anti-thrombotic activity, in addition to underlying mechanisms, when it was taken orally.
The experimental materials, PARMG and EARMG treated with methyl jasmonate (50 µM) for 2 days were obtained from Dongkook Pharmaceutical Co., Ltd. (Seoul, Korea), and kept at 4℃ until use. After steaming, PARMG was extracted with 70% ethanol to obtain EARMG. PARMG, EARMG or aspirin was orally administered in a volume of 10 mL/kg sterile purified water. Aspirin, a reference material, was used as a well-known blood flow enhancer.
Seven-week-old male Sprague-Dawley (SD) rats were from Daehan-Biolink (Eumseong, Korea), and subjected to the experiment after acclimation to the laboratory environment for 1 week. The animals were housed in a room with constant environmental conditions (temperature: 22±2℃, relative humidity: 40-70%, light-dark cycle: 12 hours, brightness: 150-300 lux, ventilation: 12 times/hour), and provided with purified water and rodent pellet diet ad libitum. All the animal experiments were approved by the Institutional Animal Care and Use Committee (IACUC) of Chungbuk National University (CBNU), Korea (Approval number: CBNUA-974-16-01), and conducted according to the Standard Operation Procedures (SOP) of Laboratory Animal Research Center (LARC) of CBNU.
Blood sample was withdrawn from the male SD rats directly into anti-coagulant citrate dextrose solution containing 0.8% citric acid, 2.2% trisodium citrate, and 2% dextrose. Washed platelets were prepared as previously described [21]. In brief, platelet-rich plasma (PRP) was obtained by centrifugation at 170 g for 7 min. Platelets were sedimented by centrifugation of the PRP at 350 g and washed with tyrode buffer. The washed platelets were resuspended in tyrode buffer and adjusted to 3×108 platelets/mL [2223].
Platelet aggregation was measured with an aggregometer (Chrono-Log Co., Harbertown, CA, USA) according to the turbidimetric method of Born and Cross [24]. The washed platelet suspension was pre-incubated with PARMG, EARMG or aspirin (125, 250 or 500 µg/mL) at 37℃ in the aggregometer under stirring at 1,200 rpm. After 3-min pre-incubation, platelet aggregation was induced by adding collagen (0.625 µg/mL), adenosine diphosphate (ADP, 10 µM) or thrombin (0.1 unit/mL). The extent of aggregation was expressed as percentage of the vehicle control value stimulated with collagen, thrombin or ADP.
Rats (n=8/group) were orally administered with PARMG, EARMG (125, 250 or 500 mg/kg) or aspirin (30 mg/kg) for 3 weeks. Forty min after the final administration, the animals were anesthetized by intramuscular injection of urethane (1 g/5 mL/kg), under constant maintenance of body temperature (36-37℃) using a heating pad. The right carotid artery of rats were exposed and detached away from the vagus nerve and surrounding tissues. Aortic blood flow rate was monitored with a laser Doppler flowmeter (AD Instruments, Colorado Springs, CO, USA). At the time point of 1 hour after the final administration, arterial thrombosis was induced by wrapping the artery with a Whatman No. 1 filter paper (3×5 mm) soaked with 70% FeCl3 solution near (5 mm anterior to) the flowmeter probe for 5 min. The blood flow was monitored for 40 min, and the time to occlusion was recorded. Animals were sacrificed at the time point of 40 min from the application of FeCl3, and the arteries were cut to observe the thrombus in the artery.
After 40-min monitoring of the blood flow in carotid artery applied with 70% FeCl3 to the external surface, the arteries were cut at intervals of 1 mm from the visible thrombus regions. Then, the length (in mm) and weight (in mg) of the thrombotic arteries were measured.
For microscopic examination, an 1.5-cm section of the ascending thoracic aorta was dissected from the heart. The injured artery was fixed in 4% paraformaldehyde, and embedded in paraffin. Paraffin sections were cut (4 µm in thickness), and stained with hematoxylin and eosin. The proportion of platelet plug to whole blood clot in cross-sectional area of the vessels was analyzed using an ImageJ 1.46b image analysis software.
After 16-hour overnight fasting (feed only), blood samples were collected from rats orally administrated with PARMG, EARMG or aspirin for 3 weeks. Complete blood cell counts (CBC) were measured with an automatic hematology analyzer (INTEGRA 400; Roche, Mannheim, Germany).
Blood samples were centrifuged at 3,000 g for 15 min at 4℃. Biochemical analysis was performed in sera using a blood chemistry analyzer (Hitachi-747; Hitachi Korea, Seoul, Korea) for lipids including total cholesterol (TC), low-density lipoproteins (LDL), high-density lipoproteins (HDL), and triglycerides (TG) as well as glucose.
All data are expressed as a mean±standard error (SE). Statistical significance was analyzed using SPSS package (version 18.0; SPSS Inc., Chicago, IL, USA). Differences among groups were compared with one-way ANOVA, followed by Dunnett's multiple-range test. P-value <0.05 was considered to be statistically significant.
PARMG did not inhibit all the collagen (0.625 µg/mL)-, ADP (10 µM)- or thrombin (0.1 unit/mL)-induced platelet aggregation up to 250 µg/mL (Figure 1). However, the significant inhibition on the collagen-induced platelet aggregation at a higher concentration (500 µg/mL) is unclear, since turbid PARMG interfered with the analysis of platelet aggregation. In comparison, a high concentration (500 µg/mL) of EARMG markedly inhibited the collagen-induced platelet aggregation to a half level, although it did not affect the ADP- and thrombin-induced aggregation. Similar results were attained by aspirin (500 µg/mL), near-fully inhibiting only collagen-induced platelet aggregation.
During whole 3-week administration period of PARMG, EARMG or aspirin, there were no abnormal symptoms in rats, and the rats gained body weights steadily in normal state.
Five-min application of 70% FeCl3 outside carotid arterial wall caused gradual decrease in the blood flow that near-fully ceased in 30 min (Figure 2). However, 3-week oral treatment with PARMG inhibited the thrombus formation in a dose-dependent manner, in which the blood flow was maintained longer than 40 min in the rats treated with a high dose (500 mg/kg) of PARMG, as observed in rats treated with aspirin (30 mg/kg) (Figure 2A). EARMG also delayed the arterial obstruction in a dose-dependent manner (Figure 2B). The mean occlusion time in the vehicle control group was calculated to be 18.1 min, based on the time point when the blood flow dropped to 10% (practical cessation) of initial flow rate (Figure 3). However, PARMG markedly prolonged the occlusion time to 25.8, 30.9, and 34.4 min at 125, 250, and 500mg/kg, respectively. Similar effects were achieved with EARMG, extending the occlusion time to 19.4, 31.7, and 34.7 min at 150, 250, and 500 mg/kg, respectively. Such a blood flow-improving activity was also attained by aspirin (30 mg/kg) that prolonged the occlusion time to 39.4 min.
Forty min after FeCl3 exposure, thrombi weighing 0.83 mg/mm were formed (Table 1). The thrombus weight decreased following treatment with PARMG, leading to a significant reduction to 0.72 mg/mm at 500 mg/kg. Such significant decreases in the thrombus weight were also achieved with EARMG (125 and 500 mg/kg) and aspirin (30 mg/kg).
As dissected 40 min after exposure to FeCl3, the arteries were entirely plugged with thrombi in the vehicle-treated rats (Figure 4). In the analysis of the thrombus, it was composed of 82.5% blood clot and 17.5% platelet plug (Figure 5). In the animals treated with PARMG, EARMG or aspirin, the thrombi were loose and had larger portions of permeable platelet plugs, relative to the flow-blocking blood clots. That is, aspirin (30 mg/kg) enhanced the portion of platelet plugs to 52%. By comparison, PARMG (500 mg/kg) increased the platelet plug ratio to 40%, and EARMG (500 mg/kg) also expanded to 43%.
In the blood biochemical analysis, PARMG (125-250 mg/kg) reduced blood TC, HDL, and TG levels, although there was no dose-response relationship (Table 2). EARMG significantly decreased blood glucose level at all doses tested (125-500 mg/kg), and lowered the lipids at a high dose (500 mg/kg). In comparison, aspirin (30 mg/kg) reduced TC, HDL, and TG.
This study was designed to investigate whether PARMG and EARMG have anti-thrombotic potential when it was taken orally. Furthermore, we attempted to find out which state of ARMG (dried powder or ethanol extract) is more effective for blood flow improvement via in vitro platelet aggregation assay as well as in vivo FeCl3-induced carotid arterial thrombosis model.
A high concentration (500 µg/mL) of ARMG significantly inhibited the collagen-induced platelet activation, in which EARMG was more potent than PARMG. Collagen fibers are exposed to circulating platelets following damage to the vessel wall and play an important role in hemostasis through the creation of a physical barrier at the site of vascular injuries, thereby limiting blood loss [252627]. The collagen fibers also stimulate platelet activation, recruiting additional platelets to the site of damage as well as consolidating the thrombus [27].
Many studies have reported the anti-platelet activity of ginsenosides [2829]. It is suggested that the major ginsenosides in ARMG should be effective in inhibiting collagen that induces platelet aggregation. Platelet activation is characterized by shape change, granule secretion, and ultimately, aggregation. These responses are triggered by an increase in intracellular Ca2+, which is induced by collagen [3031]. Thus, the inhibition of this increase in [Ca2+]i blocks the collagen-induced aggregation of human platelets [31]. The inhibitory effect of ARMG on the collagen-induced aggregation may be involved in the inhibition of Ca2+ influx by decreasing thromboxan A2 (TXA2) formation. At lower concentrations, many of the effects of collagen are enhanced by its production of TXA2 [32333435]. The collagen-induced increase in [Ca2+]i can be blocked by inhibiting the production of TXA2 via the pretreatment of platelets with cyclooxygenase inhibitors such as aspirin [303637]. It was reported that the antiplatelet aggregation effect of ginseng may be due to the decreased production and release of TXA2 [28]. Therefore, a part of the mechanisms involved in calcium mobilization and TXA2 release would be needed for further understanding of the processes whereby collagen induces platelet aggregation as well as PARMG and EARMG inhibit the process.
In the present study, the anti-thrombotic activities of PARMG and EARMG were evaluated in a FeCl3-induced carotid arterial thrombosis model. This model is widely used to study thrombogenesis in vivo. Since transition metals such as ferric ion facilitate oxidative radical formation, lipid peroxidation of cellular and tissue injuries as well as endothelial cell damage that leads to occlusive thrombus formation [38]. As shown in Figure 2 and Table 1, oral administration of PARMG and EARMG significantly delayed the occlusion time in a dose-dependent manner, indicating that the adventitious roots of mountain ginseng was able to inhibit thrombus formation in vivo and possibly act as platelet aggregation inhibitors like aspirin.
In the model of FeCl3-mediated rat artery thrombosis, the thrombi are composed of fibrin, activated platelets, and entrapped erythrocytes. Clinically, this type of thrombus is found in the coronary arteries after sudden death and acute myocardial infarction [3940]. Fibrin polymers and fibrin clots as the major components of thrombus are made from fibrinogen via the enzymatic action of thrombin. In the present study, the thrombi were observed to be separated into loose platelet plugs and compact blood (fibrin) clots. Notably, PARMG, EARMG as well as aspirin decreased the portion of impermeable blood clots, increasing the permeable platelet plugs. Such effects on the ratio of platelet plug/fibrin clot suggest that blood flow-improving compounds have an ability to change the structure or composition of fibrin network. As demonstrated in vitro platelet aggregation assay and in vivo thrombus analysis, ARMG may interrupt the early stage of hemostasis triggered by collagen, rather than that induced by thrombin. Thus, it is supposed that following interference with initial hemostasis, relatively-loose platelet adhesion remained, leading to decrease in the strong blood clot formation. So, this may make the blood vessel obstructs slowly. On the other hand, the interaction between fibrin structure and coagulation factors is a complex process, and it has been reported that the composition of fibrin structure affects its sensibility to enzymatic activity of thrombin [41]. Thus, it is suggested that PARMG and EARMG suppress fibrin clotting by interrupting the interaction between fibrinogen and thrombin or by decreasing the thrombin activity in the body environment.
In biochemical analysis, PARMG and EARMG improved the blood lipid profiles. In addition, EARMG remarkably decreased the glucose level. It was previously reported that there was a significant decrease in TG level in diabetic rats fed with the extracts of tissue-cultured adventitious roots of mountain ginseng [42]. Such an effect was also achieved in a human study [43], wherein ginsenosides stimulated lipid metabolism in diabetic individuals. Therefore, the beneficial efficacies of ARMG on the blood lipid and glucose levels may attract attentions of investigators and practitioners.
The major components of ginseng are saponins called ginsenosides. Ginsenosides are well known for cardioprotective, immuno-stimulatory, anti-fatigue, and hepatoprotective activities [44]. According to some studies, ginsenosides are the main components affecting the hemostatic and thrombotic processes [4546]. Among various ginsenosides, it was reported that both Rg1 and Rg2 have an anti-thrombosis activity [47]. Notably, Rg3 is the most potent ginsenoside in inhibiting platelet aggregation by decreasing TXA2 formation and granule secretion in platelets, which are mediated by Ca2+ influx and Ca2+ mobilization from intracellular stores [48].
Interestingly, the tissue-cultured ARMG contained higher amounts of Rb1, Rb2, Rc, Rd, Re, Rg1, and Rf ginsenosides when compared to field cultivated Korean ginseng. In addition, ginsenosides such as Rb3, Rg2, Rg3, Rh1, and Rh2 which were lacking in the extracts of field-cultivated Korean ginseng were abundant in ARMG [42]. Notably, it is well known that higher amounts of original ginsenosides and other novel ginsenosides are accumulated by treatment with methyl jasmonate during the tissue culture which is not contained process in Korean ginseng field cultivation [12]. It was reported that methyl jasmonate stimulates the ginsenoside biosynthetic pathway, thus enhancing the accumulation of ginsenosides up to 5-7 folds in adventitious roots [4950]. Besides, it seemed that steaming and extraction processes of ARMG led to different concentrations of ginsenosides. In contrast to the low concentration of Rg3 in PARMG (0.18 mg/g), that in EARMG was 0.43 mg/g, implying that various ginsenosieds including Rg3 can be more obtained during processes of ginseng products. In the present study, EARMG was somewhat superior to PRAMG in the anti-thrombotic activity which might be due to the increased ginsenosides including Rg3.
Taken together, ARMG, especially EARMG, improved blood flow by inhibiting platelet aggregation induced by collagen as well as thrombus formation induced by oxidative vascular injury. Although more actual mechanisms remain to be clarified, the adventitious roots of mountain ginseng would be good natural candidate without adverse effects for the prevention and improvement of cardiovascular and cerebrovascular diseases.
References
1. Alwan A, Armstrong T, Bettcher D, Branca F, Chisholm D, Ezzati M, Garfield R, MacLean D, Mathers C, Mendis S, Poznyak V, Riley L, Tang KC, Wild C. Global Status Report on Noncommunicable Diseases 2010. World Health Organization;2011.
2. Lissin LW, Cooke JP. Phytoestrogens and cardiovascular health. J Am Coll Cardiol. 2000; 35(6):1403–1410. PMID: 10807439.
3. Park IS, Lee HW, Ryuk JA, Ko BS. Effects of an aqueous extract of dangguijagyagsan on serum lipid levels and blood flow improvement in ovariectomized rats. Evid Based Complement Alternat Med. 2014; 2014:497836. PMID: 25276217.


4. Peter FW, Franken RJ, Wang WZ, Anderson GL, Schuschke DA, O'Shaughnessy MM, Banis JC, Steinau HU, Barker JH. Effect of low dose aspirin on thrombus formation at arterial and venous microanastomoses and on the tissue microcirculation. Plast Reconstr Surg. 1997; 99(4):1112–1121. PMID: 9091911.


5. Mackman N. Triggers, targets and treatments for thrombosis. Nature. 2008; 451(7181):914–918. PMID: 18288180.


6. Attele AS, Wu JA, Yuan CS. Ginseng pharmacology: multiple constituents and multiple actions. Biochem Pharmacol. 1999; 58(11):1685–1693. PMID: 10571242.
7. Tachikawa E, Kudo K, Harada K, Kashimoto T, Miyate Y, Kakizaki A, Takahashi E. Effects of ginseng saponins on responses induced by various receptor stimuli. Eur J Pharmacol. 1999; 369(1):23–32. PMID: 10204677.


8. Nam KY. The comparative understanding between red ginseng and white ginsengs, processed ginsengs (Panax ginseng C.A. Meyer). J Ginseng Res. 2005; 29(1):1–18.
9. Seog H, Jung C, Kim Y, Park H. Phenolic acids and antioxidant activities of wild ginseng (Panax ginseng C.A. Meyer) leaves. Food Sci Biotechnol. 2005; 14(3):371–374.
10. Yun TK. Brief introduction of Panax ginseng C.A. Meyer. J Korean Med Sci. 2001; 16(Suppl):S3–S5. PMID: 11748372.


11. Yamaguchi H, Matsuura H, Kasai R, Tanaka O, Satake M, Kohda H, Izumi H, Nuno M, Katsuki S, Isoda S, Shoji J, Gotoh K. Analysis of saponins of wild Panax ginseng. Chem Pharm Bull (Tokyo). 1988; 36(10):4177–4181. PMID: 3245991.


12. Paek KY, Murthy HN, Hahn EJ, Zhong JJ. Large scale culture of ginseng adventitious roots for production of ginsenosides. Adv Biochem Eng Biotechnol. 2009; 113:151–176. PMID: 19373448.


13. Sivakumar G. Bioreactor technology: a novel industrial tool for high-tech production of bioactive molecules and biopharmaceuticals from plant roots. Biotechnol J. 2006; 1(12):1419–1427. PMID: 17136730.


14. Sivakumar G, Yu K, Paek K. Production of biomass and ginsenosides from adventitious roots of Panax ginseng in bioreactor cultures. Eng Life Sci. 2005; 5(4):333–342.
15. Shah ZA, Gilani RA, Sharma P, Vohora SB. Cerebroprotective effect of Korean ginseng tea against global and focal models of ischemia in rats. J Ethnopharmacol. 2005; 101(1):299–307. PMID: 15970412.


16. Han K, Shin IC, Choi KJ, Yun YP, Hong JT, Oh KW. Korea red ginseng water extract increases nitric oxide concentrations in exhaled breath. Nitric Oxide. 2005; 12(3):159–162. PMID: 15797844.


17. Lee E, Zhao H, Li D, Jeong C, Kim J, Kim Y. Effect of the MeOH extract of adventitious root culture of Panax ginseng on hyperlipidemic rat induced by high fat-rich diet. Korean J Pharmacogn. 2003; 34(2):179–184.
18. Ali MB, Hahn EJ, Paek K. Protective role of Panax ginseng extract on lipid peroxidation and antioxidant status in polyethylene glycol induced Spathiphyllum leaves. Biochem Eng J. 2006; 32(3):143–148.
19. Lim H, Kim Y, Lee D, Cho S, Cho M. The antifibrotic and antioxidant activities of hot water extract of adventitious root culture of Panax ginseng (ARCP). J Appl Biol Chem. 2007; 50(2):78–84.
20. Lee SO, Lee HJ, Yu MH, Im HG, Lee IS. Total polyphenol contents and antioxidant activities of methanol extracts from vegetables produced in Ulleung island. Korean J Food Sci Technol. 2005; 37(2):233–240.
21. Jin YR, Cho MR, Ryu CK, Chung JH, Yuk DY, Hong JT, Lee KS, Lee JJ, Lee MY, Lim Y, Yun YP. Antiplatelet activity of J78 (2-Chloro-3-[2'-bromo,4'-fluoro-phenyl]-amino-8-hydroxy-1,4-naphthoquinone), an antithrombotic agent, is mediated by thromboxane (TX) A2 receptor blockade with TXA2 synthase inhibition and suppression of cytosolic Ca2+ mobilization. J Pharmacol Exp Ther. 2005; 312(1):214–219. PMID: 15328379.
22. Lee JJ, Yang H, Yoo YM, Hong SS, Lee D, Lee HJ, Lee HJ, Myung CS, Choi KC, Jeung EB. Morusinol extracted from Morus alba inhibits arterial thrombosis and modulates platelet activation for the treatment of cardiovascular disease. J Atheroscler Thromb. 2012; 19(6):516–522. PMID: 22472211.
23. Lee JJ, Jin YR, Yu JY, Munkhtsetseg T, Park ES, Lim Y, Kim TJ, Pyo MY, Hong JT, Yoo HS, Kim Y, Yun YP. Antithrombotic and antiplatelet activities of fenofibrate, a lipid-lowering drug. Atherosclerosis. 2009; 206(2):375–382. PMID: 19345949.


24. Born GV, Cross MJ. The aggregation of blood platelets. J Physiol. 1963; 168:178–195. PMID: 14056485.


25. Cowan DH, Robertson AL, Shook P, Giroski P. Platelet adherence to collagen: role of plasma, ADP, and divalent cations. Br J Haematol. 1981; 47(2):257–267. PMID: 6781525.


26. Morton LF, Peachey AR, Barnes MJ. Platelet-reactive sites in collagens type I and type III. Evidence for separate adhesion and aggregatory sites. Biochem J. 1989; 258(1):157–163. PMID: 2539100.


27. Poole AW, Watson SP. Regulation of cytosolic calcium by collagen in single human platelets. Br J Pharmacol. 1995; 115(1):101–106. PMID: 7647963.


28. Jin YR, Yu JY, Lee JJ, You SH, Chung JH, Noh JY, Im JH, Han XH, Kim TJ, Shin KS, Wee JJ, Yun YP. Antithrombotic and antiplatelet activities of Korean red ginseng extract. Basic Clin Pharmacol Toxicol. 2007; 100(3):170–175. PMID: 17309520.


29. Jeon WK, Yoo BK, Kim YE, Park SO, Hahn EJ, Paek KY, Ko BS. Anti-platelet activity of tissue-cultured mountain ginseng adventitious roots in human whole blood. Food Sci Biotechnol. 2008; 17(6):1197–1202.
30. Ardlie NG, Garrett JJ, Bell LK. Collagen increases cytoplasmic free calcium in human platelets. Thromb Res. 1986; 42(2):115–124. PMID: 3715797.


31. Roberts DE, McNicol A, Bose R. Mechanism of collagen activation in human platelets. J Biol Chem. 2004; 279(19):19421–19430. PMID: 14981087.


32. Rittenhouse SE, Allen CL. Synergistic activation by collagen and 15-hydroxy-9 alpha,11 alpha-peroxidoprosta-5,13-dienoic acid (PGH2) of phosphatidylinositol metabolism and arachidonic acid release in human platelets. J Clin Invest. 1982; 70(6):1216–1224. PMID: 6816811.


33. Pollock WK, Rink TJ, Irvine RF. Liberation of [3H]arachidonic acid and changes in cytosolic free calcium in fura-2-loaded human platelets stimulated by ionomycin and collagen. Biochem J. 1986; 235(3):869–877. PMID: 3092807.


34. McNicol A, Nickolaychuk BR. Inhibition of collagen-induced platelet activation by arachidonyl trifluoromethyl ketone. Biochem Pharmacol. 1995; 50(11):1795–1802. PMID: 8615857.


35. Nakano T, Terawaki A, Arita H. Measurement of thromboxane A2-induced elevation of ionized calcium in collagen-stimulated platelets with the photoprotein, aequorin. J Biochem. 1986; 99(4):1285–1288. PMID: 3711064.
36. Feijge MA, van Pampus EC, Lacabaratz-Porret C, Hamulyak K, Levy-Toledano S, Enouf J, Heemskerk JW. Inter-individual variability in Ca2+ signalling in platelets from healthy volunteers: effects of aspirin and relationship with expression of endomembrane Ca2+-ATPases. Br J Haematol. 1998; 102(3):850–859. PMID: 9722316.
37. Shiraishi M, Ikeda M, Fujishiro T, Fukuyama K, Ito K. Characteristics of collagen-induced Ca2+ mobilization in bovine platelets. Cell Calcium. 2000; 27(1):53–60. PMID: 10726211.
38. Jang JY, Kim TS, Cai J, Kim J, Kim Y, Shin K, Kim KS, Lee SP, Kang MH, Choi EK, Rhee MH, Kim YB. Perilla oil improves blood flow through inhibition of platelet aggregation and thrombus formation. Lab Anim Res. 2014; 30(1):21–27. PMID: 24707301.


39. Kurz KD, Main BW, Sandusky GE. Rat model of arterial thrombosis induced by ferric chloride. Thromb Res. 1990; 60(4):269–280. PMID: 2087688.


40. Lockyer S, Kambayashi J. Demonstration of flow and platelet dependency in a ferric chloride-induced model of thrombosis. J Cardiovasc Pharmacol. 1999; 33(5):718–725. PMID: 10226858.


41. Wolberg AS. Thrombin generation and fibrin clot structure. Blood Rev. 2007; 21(3):131–142. PMID: 17208341.


42. Murthy HN, Dandin VS, Lee EJ, Paek KY. Efficacy of ginseng adventitious root extract on hyperglycemia in streptozotocin-induced diabetic rats. J Ethnopharmacol. 2014; 153(3):917–921. PMID: 24709314.


43. Kim HJ, Chae IG, Lee SG, Jeong HJ, Lee EJ, Lee IS. Effects of fermented red ginseng extracts on hyperglycemia in streptozotocin-induced diabetic rats. J Ginseng Res. 2010; 34(2):104–112.


44. Wu J, Zhong JJ. Production of ginseng and its bioactive components in plant cell culture: current technological and applied aspects. J Biotechnol. 1999; 68(2-3):89–99. PMID: 10194851.


45. Matsuda H, Namba K, Fukuda S, Tani T, Kubo M. Pharmacological study on Panax ginseng C. A. Meyer. IV. Effects of red ginseng on experimental disseminated intravascular coagulation. (3). Effect of ginsenoside-Ro on the blood coagulative and fibrinolytic system. Chem Pharm Bull (Tokyo). 1986; 34(5):2100–2104. PMID: 3742652.
46. Matsuda H, Namba K, Fukuda S, Tani T, Kubo M. Pharmacological study on Panax ginseng C. A. Meyer. III. Effects of red ginseng on experimental disseminated intravascular coagulation. (2). Effects of ginsenosides on blood coagulative and fibrinolytic systems. Chem Pharm Bull (Tokyo). 1986; 34(3):1153–1157. PMID: 3731336.


47. Li CT, Wang HB, Xu BJ. A comparative study on anticoagulant activities of three Chinese herbal medicines from the genus Panax and anticoagulant activities of ginsenosides Rg1 and Rg2. Pharm Biol. 2013; 51(8):1077–1080. PMID: 23742679.
48. Lee SR, Park JH, Kim ND, Choi KJ. Inhibitory effects of ginsenoside Rg3 on platelet aggregation and its mechanism of action. Korean J Ginseng Sci. 1997; 21:132–140.
49. Yu KW, Gao W, Hahn EJ, Paek KY. Jasmonic acid improves ginsenoside accumulation in adventitious root culture of Panax ginseng C.A. Meyer. Biochem Eng J. 2002; 11(2-3):211–215.
50. Kim YS, Hahn EJ, Murthy HN, Paek KY. Adventitious root growth and ginsenoside accumulation in Panax ginseng cultures as affected by methyl jasmonate. Biotechnol Lett. 2004; 26(21):1619–1622. PMID: 15604808.


Figure 1
Inhibition of platelet aggregation by dried powder (PARMG) or ethanol extract (EARMG) of adventitious roots of mountain ginseng or aspirin. Platelet aggregation was induced by collagen (0.625 µg/mL; A), adenosine diphosphate (ADP, 10 µM; B) or thrombin (0.1 unit/mL; C). Virgule: 125 µg/mL, gray: 250 µg/mL, black: 500 µg/mL. *Significantly different from vehicle controls (collagen, ADP or thrombin alone).
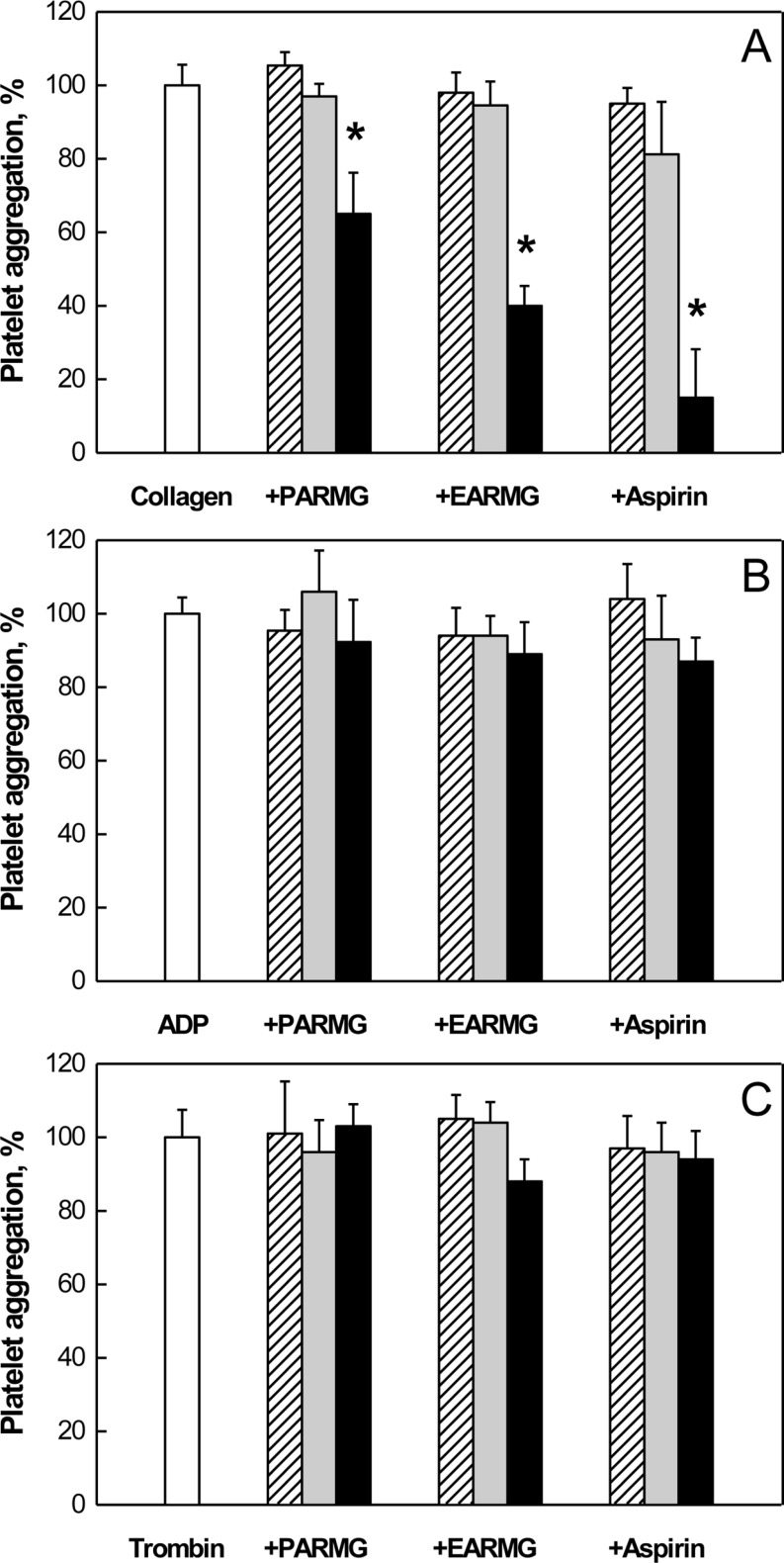
Figure 2
Time-course of carotid arterial blood flow following FeCl3 application outside the arterial wall. Dried powder (PARMG; A) or ethanol extract (EARMG; B) of adventitious roots of mountain ginseng or aspirin (A & B) were orally administered for 3 weeks prior to FeCl3 exposure. ●: vehicle (FeCl3 alone) control, ▼: low dose (125 mg/kg) of PARMG or EARMG, ■: medium dose (250 mg/kg) of PARMG or EARMG, ◆: high dose (500 mg/kg) of PARMG or EARMG, ▲: aspirin (30 mg/kg).
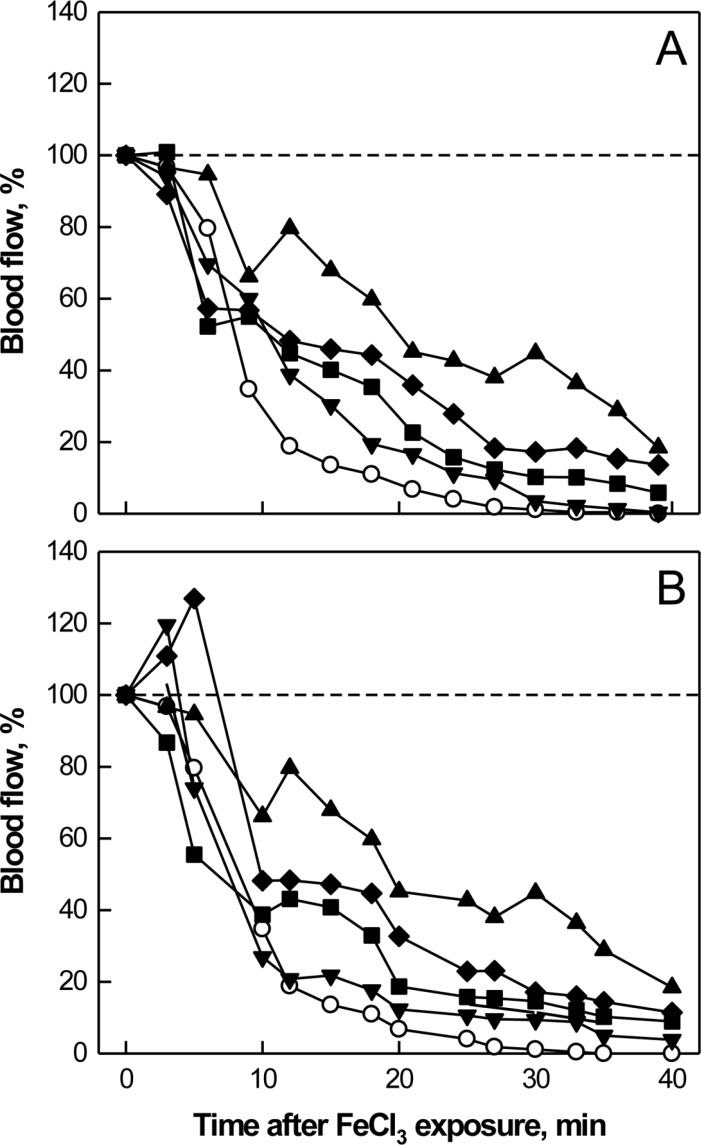
Figure 3
Time to occlusion of carotid arteries after application of FeCl3 outside the arterial wall. Dried powder (PARMG) or ethanol extract (EARMG) of adventitious roots of mountain ginseng or aspirin were orally administered for 3 weeks prior to FeCl3 exposure. *Significantly different from vehicle (FeCl3 alone) control (P<0.05).
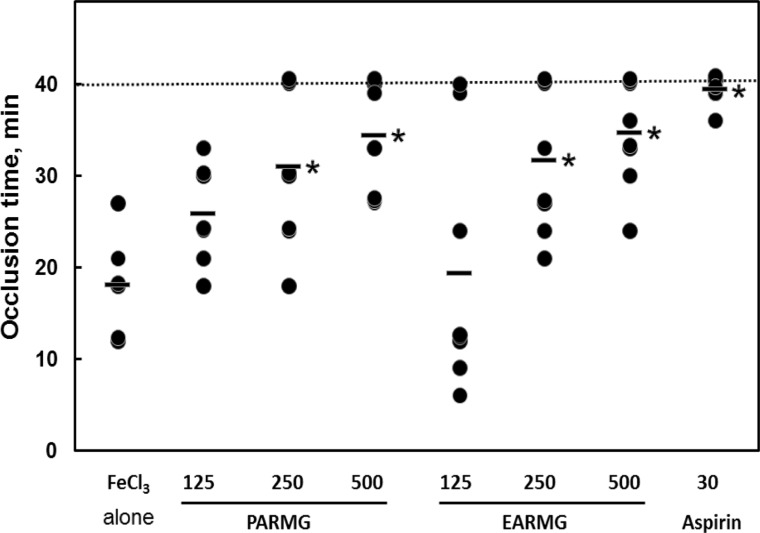
Figure 4
Representative findings of carotid arterial thrombi produced by FeCl3 application outside the arterial wall. Dried powder (PARMG) or ethanol extract (EARMG) of adventitious roots of mountain ginseng or aspirin were orally administered for 3 weeks prior to FeCl3 exposure. Gray aggregated platelet plugs (empty star) and yellow/pink blood clots (filled star) are seen in the arterial lumens exposed to FeCl3.
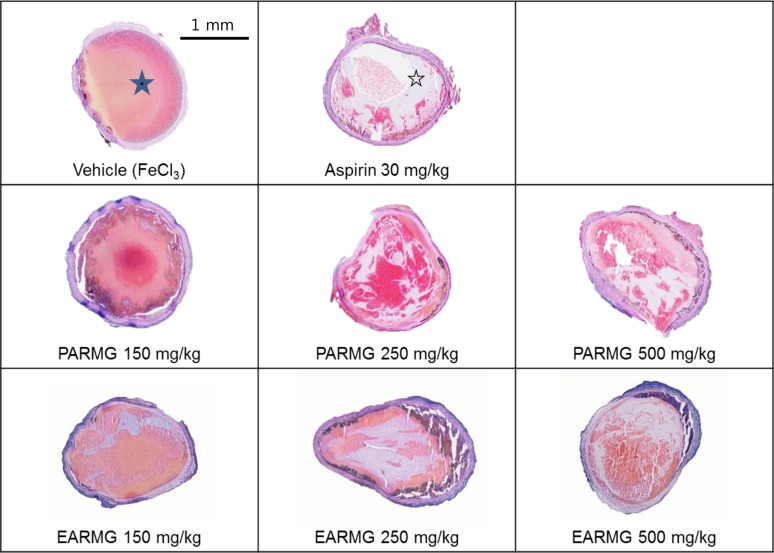
Figure 5
Platelet plug (gray) and blood clot (black) ratios in the carotid arterial thrombi produced by FeCl3 application outside the arterial wall. Dried powder (PARMG, 500 mg/kg) or ethanol extract (EARMG, 500 mg/kg) of adventitious roots of mountain ginseng or aspirin (30 mg/kg) were orally administered for 3 weeks prior to FeCl3 exposure.
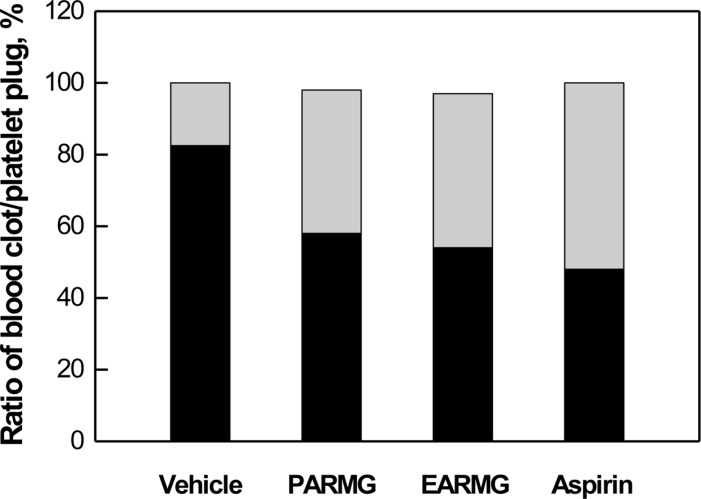
Table 1
Thrombus weight in the carotid arteries after application of FeCl3 outside the arterial wall

Treatment | Dose (mg/kg) | Thrombus (mg/mm) |
---|---|---|
FeCl3 alone | 0 | 0.83±0.03 |
PARMG | 125 | 0.78±0.03 |
250 | 0.78±0.01 | |
500 | 0.72±0.03* | |
EARMG | 125 | 0.73±0.04* |
250 | 0.81±0.07 | |
500 | 0.76±0.01* | |
Aspirin | 30 | 0.76±0.03 |
Table 2
Blood biochemistry of the rats with carotid arterial thrombosis induced by FeCl3 application outside the arterial wall
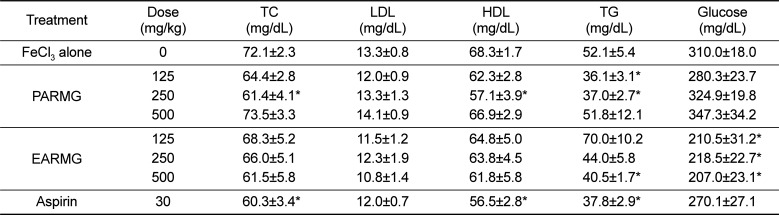
Dried powder (PARMG) or ethanol extract (EARMG) of adventitious roots of mountain ginseng or aspirin were orally administered for 3 weeks prior to FeCl3 exposure. TC, total cholesterol; LDL, low-density lipoproteins; HDL, high-density lipoproteins; TG, triglycerides. *Significantly different from vehicle (FeCl3 alone) control (P<0.05).