Abstract
The purpose of this study was to evaluate the effects of thiopental versus propofol on cardiopulmonary functions, when used as an induction agent prior to isoflurane anesthesia in rhesus monkeys. Eight healthy rhesus monkeys weighing 3.72 to 5.7 kg, 4-5 years old, were used in the study. Anesthesia was induced with thiopental or propofol intravenous injection, and then maintained with isoflurane in oxygen for 45 minutes. Cardiopulmonary measurements were obtained before and 5, 15, 30, 45, and 60 minutes after induction. The induction doses of thiopental and propofol were 19.41±0.54 and 9.33±1.02 mg/kg, respectively. In both groups, the values of heart rate, respiratory rate, temperature, systolic blood pressure, mean blood pressure, diastolic blood pressure, pH, and lactate were decreased, while the values of partial pressure of carbon dioxide, partial pressure of oxygen, total carbon dioxide, bicarbonate, oxygen saturation, and base excess in the extracellular fluid were increased, as compared with baseline. Systolic blood pressure was significantly lower in thiopental group compare to propofol group. Induction time was very short in both agents but not revealed a significant difference between both groups. However, recovery time was extremely faster in the propofol group. Our results demonstrated that propofol provides a minor suppression in systolic arterial blood pressure than thiopental sodium. In addition, propofol have a fast recovery effect from the anesthesia as well. Furthermore, it is suggested that thiopental sodium could also be used to induce anesthesia instead of propofol, despite slight more suppression of cardiopulmonary function compared to thiopental sodium.
Nonhuman primates (NHPs) have been used in a wide range of preclinical research studies such as organ transplantation as well as pharmacotoxicology for neo therapeutics. In experiments using the NHPs, a suitable anesthetic plan is very important for the safety of animals, as well as favorable outcome of the studies. One of anesthetic agents, ketamine has a wide margin of safety in many species, so it has been frequently and routinely used in order to facilitate safe handling of NHPs in many facilities [123]. In fact due to its convenience, ketamine is used to utilize not only for immobilization of NHPs, but for induction of anesthesia. However, this agent could be lead to adverse effects by repeated use, for example tolerance effect on heart rate and righting reflex in rhesus monkeys [4] or reduction in daily food intake in African green monkeys [5].
Barbiturate, thiopental sodium has been used as induction agent to facilitate intubation prior to inhalation anesthesia in many animals [678]. Despite its long history of use in research and animal anesthesia, thiopental is less frequently used as anesthetics for survival procedures in NHPs. Because of distinct respiratory suppression (e.g., apnea) at the dosages required to producing adequate analgesia, inability to modulate the depth of anesthesia, and a long period of recovery make it a less popular general anesthetic [910].
Non-barbiturate, propofol is noted for extremely rapid induction and recovery times, but previous studies have documented it to induce hypotension in dogs and rabbits [11121314]. On the other hand, some studies reported that propofol as induction agents did not induce important cardiovascular alterations like dramatic decrease of blood pressure in dogs [89]. Although both agents have been used to evaluate a range of cardiovascular responses for a broncho-provocation under continuous infusion in rhesus monkey, very limited information is available on cardiopulmonary effects by induction of anesthesia with these agents.
Actually, in an awake NHPs, intravenous injectable agents have crucial risks to use as an induction agent of anesthesia unless the monkey is pre-sedated with an intramuscular agent (i.e. most like ketamine). However, if the monkeys are conditioned submissively via positive reinforcement training and gently acclimated to physical restraint (e.g., monkey chair) prior to inhalational anesthesia, various injectable anesthetics could be selected.
The aim of this study was to evaluate the effects on the cardiopulmonary function, induction and recovery, physiologic homeostasis when they are induced with thiopental or propofol prior to isoflurane anesthesia in chair trained rhesus monkeys.
Eight female adult rhesus macaques were used in this study. The ages ranged from 4 to 5 years (mean±SD, 60.3±5.1 months) and body weights ranged from 3.72 to 5.7 kg (mean±SD, 4.44±0.7 kg). After being imported from China, a quarantine process of 1 month was conducted with the subjects in good general condition. The monkeys were maintained in single-housed cages and had daily access to food (2050 Harlan, Teklad Diets, Madison WI, USA) plus some fresh fruit and vegetables, and unlimited access to water. Their room was maintained at 24±4℃ at a relative humidity of 50±10%, with an artificial light-dark cycle of 12:12 (7:00 AM onset) and with 13-18 air changes per hour. All animals used in this study were cared for in strict accordance with the National Institutes of Health Guide for the Care and Use of Laboratory Animals.
Prior to experiment, all monkeys were trained for restraint in a chair by training program referred in our previous study [15]. On the chair, heart rate, respiration rate, core temperature, and blood pressure were measured before an anesthesia. And then blood gas analysis was performed as soon as obtained from the femoral artery. After the peripheral vein was secured, Hartmann solution (balanced electrolytes fluid) was given to the monkeys at the rate of 10mL/kg/h. Under the chair restraint, anesthetic induction of thiopental or propofol was conducted. The intubation of tracheal tube was performed immediately after the induction agent and anesthesia was maintained by 1.5% of isoflurane with oxygen supply. After 45 minutes, isoflurane was withdrawn and recovery response from anesthesia was monitored. In the thiopental treatment group (n=4), a thiopental sodium (Pentotal Sodium, JW Pharmaceutical, Korea) was slowly intravenous injected till the monkeys closed their eyes, and lost the gag reflex and pedal withdrawal response. In the propofol treatment group (n=4), propofol (PROVIVE, Claris Lifesciences Ltd., India) was also slowly intravenous injected till the monkeys showed the same response. Induction time was defined as a period of complete immobilization status following intravenous anesthetic agent injection. Recovery records were kept of arousal time at which they regained an alert appearance by raising their heads and ears, and of standing time at which they stood.
The various parameters on cardiopulmonary function were measured as follows: heart rate (HR), respiration rate (RR), core temperature, end tidal CO2 (etCO2), systolic blood pressure (SBP), diastolic blood pressure (DBP) and mean blood pressure (MBP), pH, partial pressure of CO2 (PCO2), partial pressure of O2 (PO2), base excess in extracellular fluid (Beecf), HCO3, total CO2 (TCO2), saturation of O2 (sO2), and lactate. Time intervals were before and 5, 15, 30, 45, 60 minutes after administration. All the time points were after the animals were under anesthesia with the isoflurane. Non-invasive arterial blood pressure was taken from the pediatric cuff reading. Temperature was obtained via a rectal probe. Anesthetic machine (Narkomed GS, Drager, Germany) with semi-closed type and patient monitor (Delta, Drager, Germany) were used in this study. During anesthesia, we did not use ventilator for a spontaneous respiration monitor. A small volume of blood from the femoral artery was obtained before and after anesthesia. Once the sample was obtained, blood gas was measured with an i-STAT portable electrolyte analyzer (Abbott Point of Care, Inc., USA) and a CG4+ kit. We did not use an anticoagulant such as heparin because the test was performed immediately.
The cardiopulmonary parameters were evaluated using the 2-way repeated measures ANOVA with the first factor labeled 'treatment' (thiopental vs. propofol) and second factor labeled 'time after administration'. Dunnett's test was used to compare data at each time point with baseline values within each induction regimen. Single data points (i.e., induction and recovery time) were compared with unpaired t-test. All data were reported as mean±SD.
Induction of anesthesia with thiopental or propofol was smooth and intubation was easily performed in all monkeys. No adverse effects such as vomiting or regurgitation were observed during anesthesia. Most notably, thiopental and propofol did not show critical adverse effect like a short period of apnea, as occurs after induction of anesthesia in animals. Overall, the hemodynamic, respiratory, and blood gas variables had significant within group changes, but there were no significant differences between groups, except for systolic blood pressure.
Heart rate and respiratory rate were gradually and significantly decreased in thiopental group up to 45 minutes following induction of anesthesia. Meanwhile, propofol groups showed a slight decrease of respiratory rate, as compared to the monkeys treated with thiopental (Figure 1A,B). After withdrawal from isoflurane, the decreased heart and respiratory rate were immediately restored in propofol rather than thiopental groups. In particular, the heart rate was revealed remarkable restoration in propofol group. However, there was no significant difference between the groups. Core temperature of both groups decreased significantly over a long period of time relative to baseline levels, with no significant group-wise differences (Figure 1C).
Overall, the changes of blood pressure followed a similar trend to heart rate, with decrease in SBP, DBP, and MBP relative to baseline after anesthetic induction. However, propofol group's monkeys showed a small decrease significantly in systolic blood pressure, as compared to the monkeys treated with thiopental (P=0.0125). Blood pressure rapidly decreased from 5 to 45 minutes in the monkeys treated with thiopental, as compared to the propofol group, but immediately restored to baseline after withdrawal from isoflurane (Figure 2A-C).
Inhalation anesthesia was maintained without support of a ventilator to evaluate respiratory rate and CO2 gas exchange. Although end tidal CO2 was not significantly different between the groups, propofol group showed a gradual decline, as compare to the thiopental group (Figure 3).
On blood gas analysis, the pH values were decreased significantly in only thiopental group relative to baseline, while PCO2, PO2 and sO2 values were increased significantly in both groups relative to baseline (Figure 4A-C,G). Total CO2, HCO3, and base excess values of the propofol group were also increased significantly relative to baseline, but not significant in thiopental group (Figure 4D-F). The levels of lactate showed a gradually decreasing pattern in both groups after anesthesia (Figure 4H). Overall, blood gas values were not significantly different between the groups.
The average induction dose of thiopental and propofol were 19.41±0.53 and 9.32±1.02 mg/kg respectively. Notably, induction time was not significant difference between the groups (Figure 5A), but recovery time had definite differences between the groups (Figure 5B, C). Induction time of the thiopental group (39.00±2.70 seconds) was not different from propofol group (45.50±10.34 seconds) (P=0.2697). Meanwhile, arousal and standing time of the propofol group (374 and 575 seconds, respectively) were also significantly faster than that of the thiopental group (1373 and 2326 seconds, respectively).
In this study, induction of anesthesia with thiopental or propofol was smooth and then well maintained with isoflurane in chair trained rhesus monkeys. In the induction stage, laryngeal reflex remained weakly only in one monkey treated with propofol but was not enough to impede intubation of tracheal tube. In particular, transient apnea following thiopental or propofol injection in other animals was not observed in all treated monkeys.
We designed that this study is to evaluate the effects of two anesthetic induction agents on cardiopulmonary and physiologic homeostasis in trained rhesus monkeys. HR, RR, Temperature, and blood pressure were observed a significant reduction relative to baseline in the necessary induction dose of thiopental and propofol. This results are consistent with those reported by other investigators [89]. Indeed, isoflurane-induced hypotension has been exploited in experimental studies in rhesus macaques [1617]. Therefore, isoflurane-related hypotension or vasodilation may influence on the cardiopulmonary function of thiopental and propofol induction in the same condition. However, blood pressure variation of propofol group under isoflurane anesthesia was minimal compared with thiopental group. Specifically, systolic arterial blood pressure was significantly lower in thiopental group. This effect likely is support that propofol as induction agent produces little change in cardiopulmonary function in rhesus monkeys.
Core temperature was significantly decreased until 60 minutes, despite of restoration of HR, RR, and BP at that time point. It was caused by exclusion of supportive care as supplemental heat. Therefore, it should be provided to ensure physiologic stability through intravenous fluids and warming care. EtCO2 values of both groups after induction were relatively minor changed and consistent during isoflurane anesthesia.
Several previous studies evaluating the cardiopulmonary effects of isoflurane in dogs did not mechanically ventilate the dog and as a consequence the dogs generally had higher PaCO2 values [1218]. Consistent with previous study, arterial carbon dioxide levels increased in non-ventilated monkeys in both groups of the current study. In particular, the higher values of PCO2, HCO3, and TCO2 in the monkeys treated with thiopental and propofol could reflect the respiratory suppression.
The induction dose of thiopental and propofol to effect for tracheal intubation were 19.41±0.54 and 9.33±1.02 mg/kg, respectively. According to previous reports, each dosages are relatively similar to reference doses in NHPs [1920]. In some study, 17.7 mg/kg of propofol as cumulative dose was used to allow endotracheal intubation neonatal rhesus monkeys [21]. Under ketamine injection, however, these dosage would be reduced to allow induction of anesthesia [11]. As mentioned before, ketamine is most commonly used for sedation or anesthesia in the many NHP facilities, and then other intravenous injectable (e.g., thiopental or propofol) agents are used to induction of anesthesia. It may not be necessary to initiate anesthesia with ketamine, if the monkeys already had trained well by chair device.
Although thiopental and propofol must be administered intravenously, it is possible in chair trained macaques which was conditioned by a safe and non-stressful way to gain vascular access. In NHPs, appropriate induction and maintenance of anesthesia is essential for long-term procedures, such as organ transplantation or experimental treatments. Especially, anesthetic induction is very important to the maintenance and recovery of anesthesia. A cumulative effect of serial or repeated anesthesia could result in changes to physiological status that may affect study data. In addition, it may have a detrimental effect on the health or wellbeing of the animal [422].
This study is the first report effects of thiopental vs. propofol as an induction agents on cardiopulmonary function in chair trained rhesus monkeys. The findings of present study demonstrated that propofol provides a minor suppression in systolic arterial blood pressure than thiopental sodium. In addition, propofol have a fast recovery effect from the anesthesia as well. Meanwhile, it is suggested that thiopental sodium could also be used to induce anesthesia instead of propofol, despite slight more suppression of cardiopulmonary function compared to thiopental sodium.
Acknowledgments
Our study was supported by a grant of the Korea health Technology R&D Project through the Korea Health Industry Development Institute, funded by the Ministry of Health & Welfare, Republic of Korea (grant number: HI13C0954).
References
1. Bennett JS, Gossett KA, McCarthy MP, Simpson ED. Effects of ketamine hydrochloride on serum biochemical and hematologic variables in rhesus monkeys (Macaca mulatta). Vet Clin Pathol. 1992; 21(1):15–18. PMID: 12671786.
2. Lee JI, Hong SH, Lee SJ, Kim YS, Kim MC. Immobilization with ketamine HCl and tiletamine-zolazepam in cynomolgus monkeys. J Vet Sci. 2003; 4(2):187–191. PMID: 14610374.


3. Davy CW, Trennery PN, Edmunds JG, Altman JF, Eichler DA. Local myotoxicity of ketamine hydrochloride in the marmoset. Lab Anim. 1987; 21(1):60–67. PMID: 3104668.


4. Settle TL, Rico PJ, Lugo-Roman LA. The effect of daily repeated sedation using ketamine or ketamine combined with medetomidine on physiology and anesthetic characteristics in rhesus macaques. J Med Primatol. 2010; 39(1):50–57. PMID: 19912466.


5. Springer DA, Baker KC. Effect of ketamine anesthesia on daily food intake in Macaca mulatta and Cercopithecus aethiops. Am J Primatol. 2007; 69(10):1080–1092. PMID: 17330308.
6. Brainard BM, Campbell VL, Drobatz KJ, Perkowski SZ. The effects of surgery and anesthesia on blood magnesium and calcium concentrations in canine and feline patients. Vet Anaesth Analg. 2007; 34(2):89–98. PMID: 17316389.


7. Chang J, Kim S, Jung J, Lee H, Chang D, Lee Y, Lee I, Yoon J, Choi M. Evaluation of the effects of thiopental, propofol, and etomidate on glomerular filtration rate measured by the use of dynamic computed tomography in dogs. Am J Vet Res. 2011; 72(1):146–151. PMID: 21194347.


8. Enouri SS, Kerr CL, McDonell WN, Dyson DH. Cardiopulmonary effects of anesthetic induction with thiopental, propofol, or a combination of ketamine hydrochloride and diazepam in dogs sedated with a combination of medetomidine and hydromorphone. Am J Vet Res. 2008; 69(5):586–595. PMID: 18447788.


9. Kojima K, Nishimura R, Mutoh T, Hong SH, Mochizuki M, Sasaki N. Effects of medetomidine-midazolam, acepromazinebutorphanol, and midazolam-butorphanol on induction dose of thiopental and propofol and on cardiopulmonary changes in dogs. Am J Vet Res. 2002; 63(12):1671–1679. PMID: 12492281.


10. Turner DM, Ilkiw JE. Cardiovascular and respiratory effects of three rapidly acting barbiturates in dogs. Am J Vet Res. 1990; 51(4):598–604. PMID: 2327623.
11. Foster A, Zeller W, Pfannkuche HJ. Effect of thiopental, saffan, and propofol anesthesia on cardiovascular parameters and bronchial smooth muscle in the rhesus monkey. Lab Anim Sci. 1996; 46(3):327–334. PMID: 8799941.
12. Fayyaz S, Kerr CL, Dyson DH, Mirakhur KK. The cardiopulmonary effects of anesthetic induction with isoflurane, ketamine-diazepam or propofol-diazepam in the hypovolemic dog. Vet Anaesth Analg. 2009; 36(2):110–123. PMID: 19239649.


13. Aeschbacher G, Webb AI. Propofol in rabbits. 2. Long-term anesthesia. Lab Anim Sci. 1993; 43(4):328–335. PMID: 8231090.
14. Quandt JE, Robinson EP, Rivers WJ, Raffe MR. Cardiorespiratory and anesthetic effects of propofol and thiopental in dogs. Am J Vet Res. 1998; 59(9):1137–1143. PMID: 9736392.
15. Lee JI, Shin JS, Lee JE, Jung WY, Lee G, Kim MS, Park CG, Kim SJ. Changes of N/L ratio and cortisol levels associated with experimental training in untrained rhesus macaques. J Med Primatol. 2013; 42(1):10–14. PMID: 23131089.


16. Enlund M, Andersson J, Hartvig P, Valtysson J, Wiklund L. Cerebral normoxia in the rhesus monkey during isoflurane- or propofol-induced hypotension and hypocapnia, despite disparate blood-flow patterns. A positron emission tomography study. Acta Anaesthesiol Scand. 1997; 41(8):1002–1010. PMID: 9311398.
17. Portier KG, Broillet A, Rioufol G, Lepage OM, Depecker M, Taborik F, Tranquart F, Contamin H. A novel minimal invasive closed chest myocardial ischaemia reperfusion model in rhesus monkeys (Macaca mulatta): improved stability of cardiorespiratory parameters. Lab Anim. 2012; 46(2):129–135. PMID: 22334875.


18. Mutoh T, Nishimura R, Kim HY, Matsunaga S, Sasaki N. Cardiopulmonary effects of sevoflurane, compared with halothane, enflurane, and isoflurane, in dogs. Am J Vet Res. 1997; 58(8):885–890. PMID: 9256976.
19. Fanton JW, Zarr SR, Ewert DL, Woods RW, Koenig SC. Cardiovascular responses to propofol and etomidate in long-term instrumented rhesus monkeys (Macaca mulatta). Comp Med. 2000; 50(3):303–308. PMID: 10894497.
20. Ator NA. Selectivity in generalization to GABAergic drugs in midazolam-trained baboons. Pharmacol Biochem Behav. 2003; 75(2):435–445. PMID: 12873636.


21. Martin LD, Dissen GA, McPike MJ, Brambrink AM. Effects of anesthesia with isoflurane, ketamine, or propofol on physiologic parameters in neonatal rhesus macaques (Macaca mulatta). J Am Assoc Lab Anim Sci. 2014; 53(3):290–300. PMID: 24827572.
22. Lugo-Roman LA, Rico PJ, Sturdivant R, Burks R, Settle TL. Effects of serial anesthesia using ketamine or ketamine/medetomidine on hematology and serum biochemistry values in rhesus macaques (Macaca mulatta). J Med Primatol. 2010; 39(1):41–49. PMID: 19878432.


Figure 1
The variation of (A) heart rate, (B) respiratory rate, (C) core temperature after induction with thiopental (closed circle) or propofol (open circle) following maintenance of isoflurane anesthesia by 45 minutes. Data are presented as the mean±SD. *P<0.05 in thiopental group and †P<0.05 in propofol for significant different from baseline.
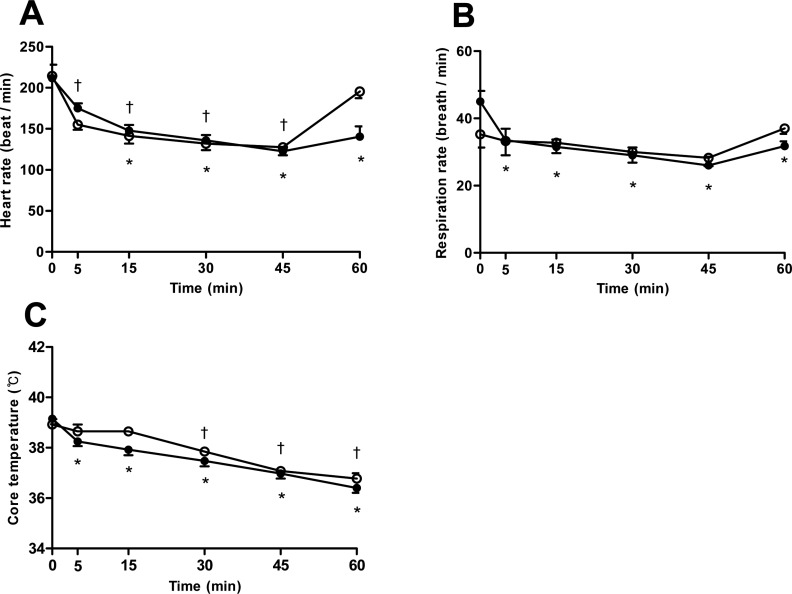
Figure 2
The variation of (A) systolic, (B) diastolic, and (C) mean blood pressure after induction with thiopental (closed circle) or propofol (open circle) following maintenance of isoflurane anesthesia by 45 minutes. Data are presented as the mean±SD. *P<0.05 in thiopental group and †P<0.05 in propofol group for significant different from baseline.
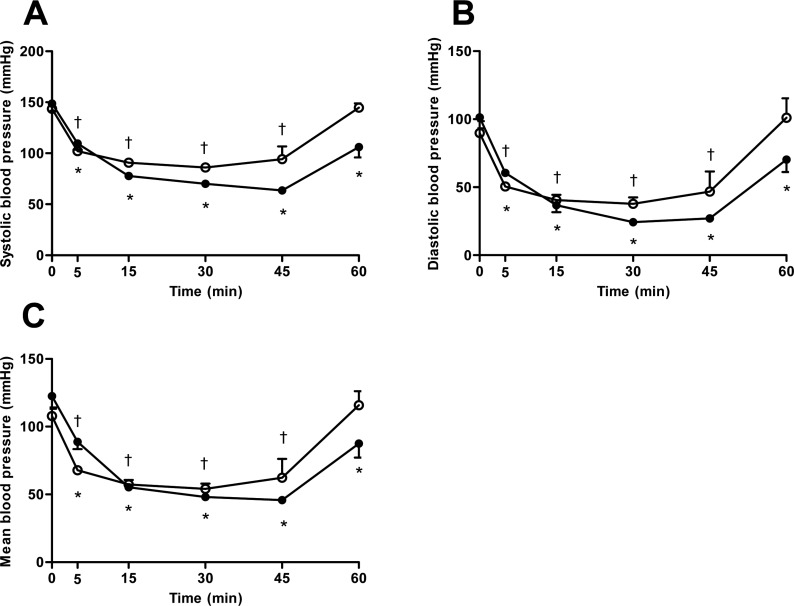
Figure 3
The variation of end tidal carbon dioxide (etCO2) after induction with thiopental (closed circle) or propofol (open circle) following maintenance of isoflurane anesthesia by 45 minutes. Data are presented as the mean±SD.
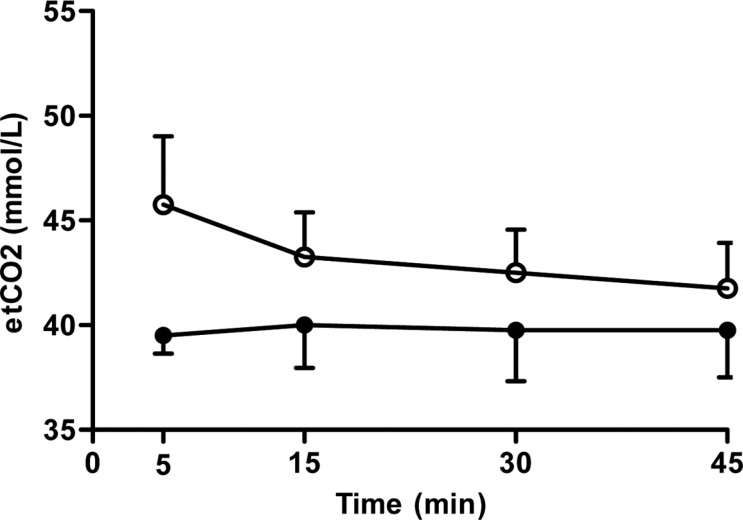
Figure 4
The variation of (A) pH, (B) partial pressure of carbon dioxide (PCO2), (C) partial pressure of oxygen (PO2), (D) base excess in the extracellular fluid (Beecf), (E) bicarbonate (HCO3), (F) total carbon dioxide (TCO2), (G) oxygen saturation (sO2) and (H) lactate after induction with thiopental (closed circle) or propofol (open circle) following maintenance of isoflurane anesthesia by 45 minutes. Data are presented as the mean±SD. *P<0.05 in thiopental group and †P<0.05 in propofol group for significant different from baseline.
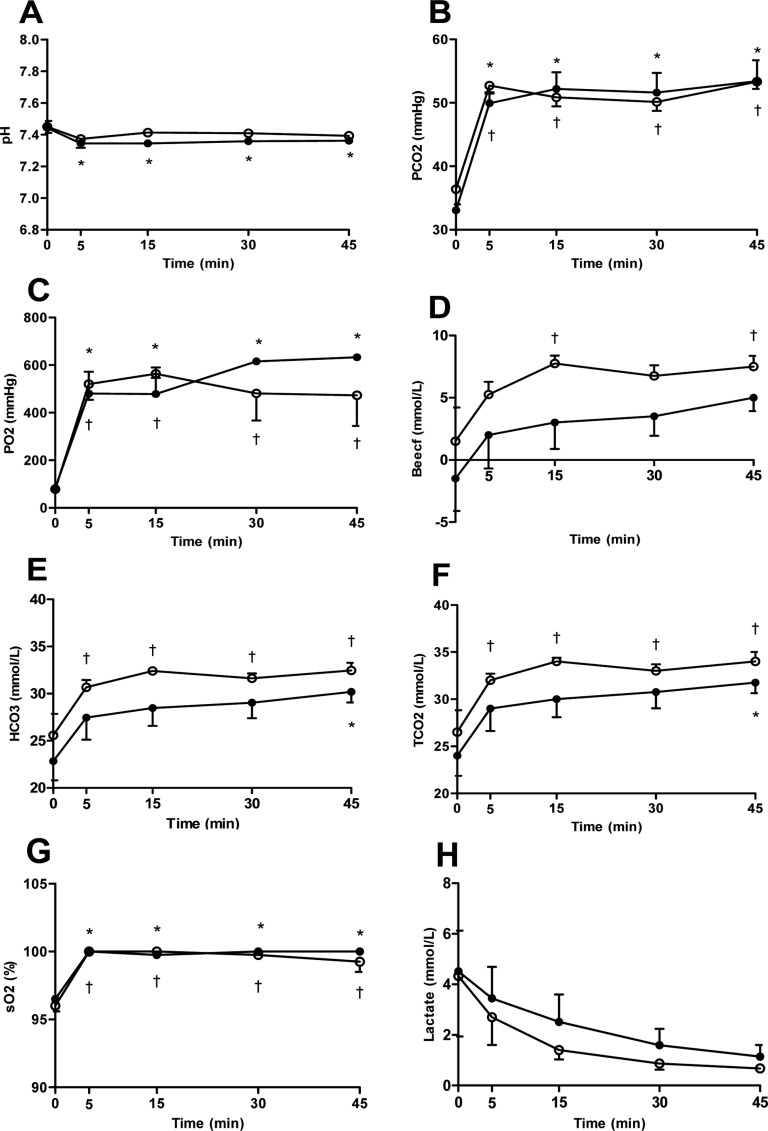