Abstract
Animal models of constipation induced with drugs and diet have been widely employed to investigate therapeutic effects and the action mechanism of drugs against this disease. ICR mice were selected to produce this disease model through oral administration of loperamide (Lop), even though SD rats are commonly utilized in studies of constipation. To compare the responses of ICR mice obtained from three different sources to constipation inducers, alterations in stool number, histopathological structure, mucin secretion and opioid-receptor downstream signaling pathway were measured in Korl:ICR (Korea FDA source), A:ICR (USA source) and B:ICR (Japan source) injected with low and high concentrations of Lop (LoLop and HiLop). The number, weight and moisture content of stools decreased significantly in the Lop treated group of all ICR relative to the Vehicle treated group. Additionally, decreased mucosa layer thickness, muscle thickness, and mucin secretion were observed in the transverse colon of Lop treated ICR mice, while a similar number of goblet cells and crypt of lieberkuhn were detected in the same group. Furthermore, a similar change in the level of Gα expression and PKC phosphorylation was detected in the Lop treated group relative to the vehicle treated group, while some differences in the change pattern were observed in the B:ICR group. Therefore, these results of the present study provide strong additional evidence that Korl:ICR, A:ICR and B:ICR derived from different sources have a similar overall response to constipation induced by Lop injection, although there were a few differences in the magnitude of their responses.
Constipation is a chronic gastrointestinal disorder characterized by infrequent bowel movements, difficulty during defecation, and sensation of incomplete bowel evacuation [123]. This disease is often caused by various factors such as insufficient dietary fiber intake, inadequate fluid intake, decreased physical activity, side effects of medication, hypothyroidism, and obstruction by colorectal cancer [4]. In addition, chronic constipation is classified into one of three groups by assessment of colonic transit and anorectal function; slow transit constipation, pelvic floor dysfunction (functional defecatory disorders) and normal transit or irritable bowel syndrome [5]. However, more than half of patients with chronic constipation show normal transit (59%), while only 25% of patients show functional defecatory disorders, slow transit (13%) or a combination of these conditions (3%) [6].
To date, a wide variety of animal models for human diseases have been applied to investigate the therapeutic effects of chemical drugs or herbal medicines, as well as to study the mechanism of action of candidate drugs. The data obtained using these models have contributed greatly to our understanding of the complex motor patterns known to exist in the human colon [7]. Two methods are commonly used to produce constipation animal models, drug and diet induction. Oral administration and subcutaneous injection of Lop hydrochloride have been shown to successfully induce chronic constipation in many previous studies [89], while feeding a low-fiber diet containing 41.5% cornstarch, 24.5% milk casein, 10.0% sucrose, 10.0% dextrin, 7.0% mineral mixture, 6.0% corn oil, and 1.0% vitamin mixture for 5 weeks was induced the same condition [10].
Several strains of rodents have been utilized to conduct most experiments in studies of Lop-induced constipation, including SD rats, Wistar rats, Balb/c mice, ICR mice and ddY mice [811121314], although ICR mice have only been used in two studies [1516]. However, most studies conducted to date have used only one strain of one animal species to produce animal models for constipation and to evaluate the anti-constipation activity of drugs and extracts from herbal medicines. Moreover, no studies have provided scientific evidence for comparative analysis of the response of ICR mice derived from different sources to chemicals that cause constipation.
Therefore, in the present study, we was compared the response of ICR mice (Korl:ICR, A:ICR and B:ICR) derived from three difference sources to Lop induced constipation and evaluated the characteristics Korl:ICR mice established by the Korea FDA. These results presented herein provide the first scientific evidence of a similar response to Lop induced constipation in the transverse colon of Korl:ICR, A:ICR and B:ICR, although there were slight differences in the magnitude of these effects.
The animal protocol used in this study was reviewed and approved based on the ethical procedures for scientific care set by the Pusan National University-Institutional Animal Care and Use Committee (PNU-IACUC; Approval Number PNU-2014-0572). Six-week-old male ICR mice were obtained from three difference sources. Specifically, Korl:ICR mice were kindly provided by the Department of Laboratory Animal Resources of the National Institute of Food and Drug Safety Evaluation (NIFDS, Chungju, Korea). The other two group of ICR mice (A:ICR and B:ICR) were purchased from vendors located in the United States (Vendor A) and Japan (Vendor B). All mice were handled in the Pusan National University-Laboratory Animal Resources Center, which is accredited by the Korea Food and Drug Administration (FDA) (Accredited Unit Number-000231) and AAALAC International according to the National Institutes of Health guidelines (Accredited Unit Number; 001525). Animals were provided with ad libitum access to a standard irradiated chow diet (Samtako Inc.) consisting of moisture (12.5%), crude protein (25.43%), crude fat (6.06%), crude fiber (3.9%), crude ash (5.31%), calcium (1.14%), phosphorus (0.99%) and water. During the experiment, rats were maintained in a specific pathogen-free (SPF) state under a strict light cycle (lights on at 08:00 h and off at 20:00 h) at 23±2℃ and 50±10% relative humidity.
Constipation of ICR mice were induced based on traditional methods as described in previous studies, with some modification [89]. Briefly, 8-week-old ICR mice in each group (n=24) were assigned to either a non-constipation group (Vehicle treated group, n=8) or a constipation group (n=16). Constipation was induced by Lop injection at regular intervals. First, ICR mice were subcutaneously injected with Lop (Sigma-Aldrich, MO, USA) (4 mg/kg weight) in 0.9% sodium chloride twice a day for 4 days. After a 3 day stationary phase, two different concentrations (4 mg/kg or 8 mg/kg) of Lop were administered to two subgroups (LoLop treated subgroup and HiLop treated subgroup) in the same way for 4 days. Additionally, a vehicle treated group was injected with 0.9% sodium chloride alone (Figure 1). At 24 h after final treatment, all animals were euthanized using CO2 gas, after which tissue samples were acquired and stored in Eppendorf tubes at −70℃ until assay.
Alterations in food intake, water consumption and body weight of ICR mice derived from three different sources treated with Vehicle or Lop were measured daily at 10:00 am throughout the experimental period using an electrical balance and a measuring cylinder. All measurements were performed three times to ensure accuracy.
To collect pure stool and urine without any contamination, all ICR mice were bred in metabolic cages (Daejong Instrument Industry Co., LTD, Seoul, Korea) during the experimental period. The stool number and weight were measured as described in previous studies [917]. The stools excreted from each ICR mouse was collected at 10:00 am, after which stool samples were weighed three times per sample using an electric balance and the number of stools was counted three times. Also, the reduction rate of stool number was calculated using the following formula;
SV was represented stool number of Vehicle-treated group, while SL was indicated the stool number of Lop-treated group.
Furthermore, the stool moisture content was analyzed using the following formula;
A is indicated the weight of stools firstly collected after Lop administration, while B is represented the weight of stools after the dry at 60℃ for 12 hr.
Total proteins (30 mg) collected from the transverse colons of subset groups (Vehicle, LoLop and HiLop treated ICR mice) were separated by 4-20% sodium dodecyl sulfate-polyacrylamide gel electrophoresis (SDS-PAGE) for 3 h, after which the resolved proteins were transferred to nitrocellulose membranes for 2 h at 40 V. Each membrane was then incubated separately with primary antibody, anti-Gα (Abcame, Cambridge, UK), anti-PKC (Cell Signaling Technology Inc., Cambridge, MA, USA), anti-p-PKC (Cell Signaling Technology Inc.), or anti-actin (Sigma-Aldrich, St. Louis, MO, USA) overnight at 4℃. Next, the membranes were washed with washing buffer (137 mM NaCl, 2.7 mM KCl, 10 mM Na2HPO4, 2 mM KH2PO4, and 0.05% Tween 20) and incubated with horseradish peroxidase-conjugated goat anti-rabbit IgG (Zymed Laboratories, South San Francisco, CA, USA) at a dilution of 1:1,000 and room temperature for 2 h. Finally, the membrane blots were developed using Chemiluminescence Reagent Plus kits (Pfizer, New York, NY, USA and Pharmacia, New York, NY, USA).
Transverse colons collected from ICR mice treated with vehicle and Lop were fixed with 10% formalin for 12 h, embedded in paraffin wax, and then sectioned into 5 µm thick slices that were stained with hematoxylin & eosin (H&E, Sigma-Aldrich). Morphological features of these sections were observed by light microscopy, after which the mucosa thickness, muscle thickness, flat luminal surface thickness, number of goblet cells and number of crypt of lieberkuhn were measured using Leica Application Suite (Leica Microsystems, Switzerland). The three different sites in the transverse colon of five rats per group were evaluated in triplicate. Data represent the mean±SD from three replicates. A P value of <0.05 was considered significant after One-way ANOVA.
For mucin staining, transverse colons collected from ICR mice were fixed with 10% formalin for 48 h, embedded in paraffin wax, and then sectioned into 3 µm thick slices that were subsequently deparaffinized with xylene and rehydrated. Next, the tissue sections on the slides were rinsed with distilled water and stained with an Alcian Blue Stain kit (IHC WORLD, Woodstock, MD, USA). Finally, the morphological features in the stained colon sections were observed by light microscopy
One-way ANOVA was used to determine whether or not significant differences existed between the Korl:ICR group and the A:ICR or B:ICR group (SPSS for Windows, Release 10.10, Standard Version, Chicago, IL, USA). All values were expressed as the means±SD. A P value of <0.05 was considered significant.
To establish the induction conditions for constipation, Lop (5 mg/kg) was administered to Korl:ICR mice using two different methods, oral administration and subcutaneous injection, for 3 continuous days. However, a significant decrease of stool number and weight was not detected in any ICR groups (Table 1). We next tried a novel strategy consisting of two injection phases and one stationary phase to induce constipation. During the first 4 day, 4 mg/kg of Lop were subcutaneously injected into Korl:ICR mice, after which they were allowed to rest for 3 days. Following the resting period, Korl:ICR mice received two different concentrations of Lop, 4 mg/kg (LoLop) and 8mg/kg (HiLop), for 4 days. The number, weight and moisture content of stool decreased by 15-47%, 12-44% and 15-79% in the LoLop and HiLop treated subgroup, while feeding behavior related factors were maintained at a constant level (Table 1). Taken together, these results indicate that the interval injection of Lop can be used to induce constipation in ICR mice derived from three different sources.
We investigated whether Lop treatment could affect the feeding behavior and excretion parameters of constipated ICR mice derived from three different sources. To accomplish this, food intake, water consumption, and stool number and weight were measured in the three constipated ICR groups after Lop treatment. As shown in Table 2, three related factors, feeding behavior, body weight, and food and water consumption, did not differ significantly between the Vehicle treated subgroup and the Lop treated subgroups within each group, although water consumption was slightly lower in the HiLop treated subgroup. Among these parameters, food intake and water consumption were higher in the A:ICR group than the Korl:ICR. However, the stool number, weight and moisture content were dramatically decreased in the Lop treated subgroup relative to the Vehicle treated subgroup. Furthermore, these dose dependent decreases were commonly observed in the Korl:ICR, A:ICR and B:ICR group, although slight differences in the decrease rate of stool number were observed (Table 2, Figure 2). Specifically, the stool number of the B:ICR showed a lower decrease rate in the Lop treated subgroup and the decrease rate did not depend on the increase in Lop concentration (Table 2, Figure 2). Therefore, the above results suggest that Lop injection at regular intervals can successfully decrease some execration parameters, but does not lead to any significant alterations in feeding behavior. Furthermore, these changes were commonly observed in Korl:ICR, A:ICR and B:ICR mice.
Several histological parameters were measured in H&E stained transverse colons of Korl:ICR, A:ICR and B:ICR following treatment with Lop. Although there were a few variations in the statistical significance of each group, histopathological changes were observed in all Lop treated subgroups. The muscle thickness and mucosa layer length was significantly shorter in the Lop treated subgroup than the Vehicle treated subgroup, and these decreases depended on the increase of Lop concentration. Furthermore, the highest levels of muscle thickness and mucosa layer length were detected in the transverse colon of A:ICR (Figure 3). Therefore, the above results suggest that the histopathological structure of constipation was successfully induced by Lop treatment of Korl:ICR, A:ICR and B;ICR mice from three different sources.
To characterize the ability to secrete mucin in the transverse colon of ICR mice derived from three different sources following Lop injection, the levels of mucin in tissue sections stained with Alcian blue were observed. The tissue region stained with dark blue color was concentrated in the crypt in the mucosa layer of the transvers colon of all subset groups. However, the total level of mucin decreased dramatically in the Lop treated subgroup compared to the Vehicle treated subgroup. Furthermore, three ICR groups showed similar patterns in the ability to secrete mucin following Lop injection (Figure 4). Therefore, the results of the present study suggest that suppression of the ability to secrete mucin may be successfully induced by Lop injection in the transverse colon of Korl:ICR, A:ICR and B:ICR from three different sources.
Finally, we examined whether constipation induced by Lop injection was accompanied by altered G protein signaling by measuring the expression of Gα and PKC protein in the transverse colon of subset groups. Gα expression was lower in the LoLop treated subgroup than the Vehicle treated subgroup. Following HiLop injection, this level was slightly increased relative to that of the LoLop treated group, regardless of concentration decrease. In addition, these patterns of Gα expression were commonly observed in Korl:ICR, A:ICR and B:ICR mice (Figure 5).
However, PKC phosphorylation showed the different patterns than Gα expression. Specifically, the level of PKC phosphorylation was dramatically decreased in the Lop treated subgroup relative to the Vehicle treated subgroup in a dose dependent manner, although the decrease rate varied. The lowest decrease in PKC phosphorylation was detected in the LoLop treated subgroup of the A:ICR mice (Figure 5B). Nevertheless, the results indicate that the G protein signaling pathway of Korl:ICR, A:ICR and B:ICR mice showed a similar response to Lop injection.
Animal models for constipation have been induced by various agents including Lop [8], clonidine [15], morphine [18], opioid receptor antagonist [19], clozapine [20] and carbon [2122]. Among these, Lop and carbon have been applied to produce constipation models in many studies. In addition, appropriate rodent species with high susceptibility have been selected from the many available mouse and rat models. However, ICR mice have rarely been used to produce chronic constipation models, even though they also widely used in toxicology (safety and efficacy testing), cancer research, aging, transgenesis experiments and gene mapping strategies [232425]. In this study, we investigated whether Korl:ICR, A:ICR and B:ICR mice could be utilized to investigate chronic constipation. We also applied a novel strategy in which two different concentrations (4 and 8 µg/mL) of Lop were utilized to produce an animal model for constipation and investigated differences in the responses to these chemicals among ICR mice derived from three different sources. The results presented here provide the first evidence that Korl:ICR, A:ICR and B:ICR show an overall similar response to Lop induced constipation, although some differences were detected in Gα expression and PKC phosphorylation.
Lop is well documented as a constipation inducer and commonly used to induce models of spastic constipation. Lop inhibits intestinal motility and fluid secretion, leading to reduced fecal evacuation time and intestinal luminal transit [26]. In the case of SD rats, constipation was successfully induced by subcutaneous injection of 3-5mg/kg Lop for 3-6 continuous days in most experiments [8]. However, constipation phenotypes such as decreased stool number and weight in ICR mice were observed after oral administration of 5 mg/kg Lop [1516]. In the present study, we attempted to induce constipation in ICR mice derived from three different sources using Lop injection. Following subcutaneous injection of Lop (4 mg/kg) for 4 days, mice were allowed to rest for 3 days, after which they were injected with 4 or 8 mg/kg of Lop for 4 days. This strategy successfully induced the constipation in ICR mice, although their pathological symptoms varied. Actually, the interval injection strategy has been widely applied to induce immunological responses such as vaccination and allergen immunotherapy [27].
Since being developed by the Dr. T. S. Hauschka of Fox Chase Cancer Center in 1948, the ICR mice used in this study have been extensively applied to toxicology and pharmacology studies and product safety testing because they have good reproductive performance and rapid growth [232425]. In addition, ICR mice have been used in several comparison studies to characterize the response of physiological properties to chemicals or hormones. Studies using his model have focused on the incidence of 5-azacytidine-induced exencephaly [28], lung injury by butylated hydroxytoluene [29], styreneinduced hepato/pneumotoxicity [30] and the response to growth hormone by chondrocytes [31]. However, the response to Lop has not been examined in ICR mice from different sources to date. The results presented herein provide the first evidence that a similar response can be detected in Korl:ICR, A:ICR and B:ICR provided from the Korea FDA or commercial vendors, although there were slight differences in the response rate within each analysis factor. Moreover, further studies are needed to investigate the response to other constipation inducers, including carbon and opioids.
Overall, we established the induction conditions for constipation using Lop in ICR mice as well as investigated the responses of ICR mice provided from different sources to two concentrations of Lop with constipation inducing activity. Three ICR groups, Korl:ICR, A:ICR and B:ICR, showed a similar response of stool parameters, histopathological structure and signaling pathways of opioid receptors to constipation inducers. Therefore, our results suggest that Korl:ICR mice, as well as ICR mice from other commercial vendors can be widely applied to produce animal models for chronic constipation.
Acknowledgments
We thank Jin Hyang Hwang, the animal technician, for directing the animal care and use at the Laboratory Animal Resources Center. This project was supported by a grant from BIOREIN (Laboratory Animal Bio Resources Initiative) from the Ministry of Food and Drug Safety in 2015.
References
1. Walia R, Mahajan L, Steffen R. Recent advances in chronic constipation. Curr Opin Pediatr. 2009; 21(5):661–666. PMID: 19606041.


2. McCallum IJ, Ong S, Mercer-Jones M. Chronic constipation in adults. BMJ. 2009; 338:b831. PMID: 19304766.


3. Emmanuel AV, Tack J, Quigley EM, Talley NJ. Pharmacological management of constipation. Neurogastroenterol Motil. 2009; 21:41–54. PMID: 19824937.


4. Leung WK, To KF, Man EP, Chan MW, Hui AJ, Ng SS, Lau JY, Sung JJ. Detection of hypermethylated DNA or cyclooxygenase-2 messenger RNA in fecal samples of patients with colorectal cancer or polyps. Am J Gastroenterol. 2007; 102(5):1070–1076. PMID: 17378912.


5. Bharucha AE. Constipation. Best Pract Res Clin Gastroenterol. 2007; 21(4):709–731. PMID: 17643910.


6. Nyam DC, Pemberton JH, Ilstrup DM, Rath DM. Long-term results of surgery for chronic constipation. Dis Colon Rectum. 1997; 40(3):273–279. PMID: 9118740.


7. Zarate N, Spencer NJ. Chronic constipation: lessons from animal studies. Best Pract Res Clin Gastroenterol. 2011; 25(1):59–71. PMID: 21382579.


8. Kim JE, Lee YJ, Kwak MH, Ko J, Hong JT, Hwang DY. Aqueous extracts of Liriope platyphylla induced significant laxative effects on loperamide-induced constipation of SD rats. BMC Complement Altern Med. 2013; 13:333. PMID: 24274470.


9. Wintola OA, Sunmonu TO, Afolayan AJ. The effect of Aloe ferox Mill. in the treatment of loperamide-induced constipation in Wistar rats. BMC Gastroenterol. 2010; 10:95. PMID: 20723249.


10. Kakino M, Tazawa S, Maruyama H, Tsuruma K, Araki Y, Shimazawa M, Hara H. Laxative effects of agarwood on low-fiber diet-induced constipation in rats. BMC Complement Altern Med. 2010; 10:68. PMID: 21078136.


11. Wu D, Wang X, Zhou J, Yuan J, Cui B, An R, Hu Z. Traditional Chinese formula, lubricating gut pill, improves loperamide-induced rat constipation involved in enhance of Cl− secretion across distal colonic epithelium. J Ethnopharmacol. 2010; 130(2):347–353. PMID: 20488235.


12. Kojima R, Nozawa K, Doihara H, Keto Y, Kaku H, Yokoyama T, Itou H. Effects of novel TRPA1 receptor agonist ASP7663 in models of drug-induced constipation and visceral pain. Eur J Pharmacol. 2014; 723:288–293. PMID: 24291101.


13. Choi JS, Kim JW, Cho HR, Kim KY, Lee JK, Sohn JH, Ku SK. Laxative effects of fermented rice extract in rats with loperamide-induced constipation. Exp Ther Med. 2014; 8(6):1847–1854. PMID: 25371743.


14. Chen W, Chung HH, Cheng JT. Opiate-induced constipation related to activation of small intestine opioid μ2-receptors. World J Gastroenterol. 2012; 18(12):1391–1396. PMID: 22493554.


15. Zhou M, Jia P, Chen J, Xiu A, Zhao Y, Zhan Y, Chen P, Zhang J. Laxative effects of Salecan on normal and two models of experimental constipated mice. BMC Gastroenterol. 2013; 13:52. PMID: 23514598.


16. Li C, Nie SP, Zhu KX, Xiong T, Li C, Gong J, Xie MY. Effect of Lactobacillus plantarum NCU116 on loperamide-induced constipation in mice. Int J Food Sci Nutr. 2015; 66(5):533–538. PMID: 25822005.
17. Lee HY, Kim JH, Jeung HW, Lee CU, Kim DS, Li B, Lee GH, Sung MS, Ha KC, Back HI, Kim SY, Park SH, Oh MR, Kim MG, Jeon JY, Im YJ, Hwang MH, So BO, Shin SJ, Yoo WH, Kim HR, Chae HJ, Chae SW. Effects of Ficus carica paste on loperamide-induced constipation in rats. Food Chem Toxicol. 2012; 50:895–902. PMID: 22178225.
18. Ono H, Nakamura A, Matsumoto K, Horie S, Sakaguchi G, Kanemasa T. Circular muscle contraction in the mice rectum plays a key role in morphine-induced constipation. Neurogastroenterol Motil. 2014; 26(10):1396–1407. PMID: 25041353.


19. Mori T, Shibasaki Y, Matsumoto K, Shibasaki M, Hasegawa M, Wang E, Masukawa D, Yoshizawa K, Horie S, Suzuki T. Mechanisms that underlie μ-opioid receptor agonist-induced constipation: differential involvement of μ-opioid receptor sites and responsible regions. J Pharmacol Exp Ther. 2013; 347(1):91–99. PMID: 23902939.


20. Chukhin E, Takala P, Hakko H, Raidma M, Putkonen H, Räsänen P, Terevnikov V, Stenberg JH, Eronen M, Joffe G. In a randomized placebo-controlled add-on study orlistat significantly reduced clozapine-induced constipation. Int Clin Psychopharmacol. 2013; 28(2):67–70. PMID: 23187856.


21. Zhao X, Suo HY, Qian Y, Li GJ, Liu ZH, Li J. Therapeutic effects of Lactobacillus casei Qian treatment in activated carbon-induced constipated mice. Mol Med Rep. 2015; 12(2):3191–3199. PMID: 25955533.
22. Suo H, Zhao X, Qian Y, Li G, Liu Z, Xie J, Li J. Therapeutic effect of activated carbon-induced constipation mice with Lactobacillus fermentum Suo on treatment. Int J Mol Sci. 2014; 15(12):21875–21895. PMID: 25464378.
23. Chia R, Achilli F, Festing MF, Fisher EM. The origins and uses of mouse outbred stocks. Nat Genet. 2005; 37(11):1181–1186. PMID: 16254564.


24. Cui S, Chesson C, Hope R. Genetic variation within and between strains of outbred Swiss mice. Lab Anim. 1993; 27(2):116–123. PMID: 8501892.


25. Lehoczky JA, Cai WW, Douglas JA, Moran JL, Beier DR, Innis JW. Description and genetic mapping of Polypodia: an X-linked dominant mouse mutant with ectopic caudal limbs and other malformations. Mamm Genome. 2006; 17(9):903–913. PMID: 16964440.


26. Holzer P. Opioid receptors in the gastrointestinal tract. Regul Pept. 2009; 155(1-3):11–17. PMID: 19345246.


27. Castiglione F, Mantile F, De Berardinis P, Prisco A. How the interval between prime and boost injection affects the immune response in a computational model of the immune system. Comput Math Methods Med. 2012; 2012:842329. PMID: 22997539.


28. Matsuda M. Comparison of the incidence of 5-azacytidine-induced exencephaly between MT/HokIdr and Slc:ICR mice. Teratology. 1990; 41(2):147–154. PMID: 1690922.


29. Kehrer JP, DiGiovanni J. Comparison of lung injury induced in 4 strains of mice by butylated hydroxytoluene. Toxicol Lett. 1990; 52(1):55–61. PMID: 2356571.


30. Carlson GP. Comparison of mouse strains for susceptibility to styrene-induced hepatotoxicity and pneumotoxicity. J Toxicol Environ Health. 1997; 51(2):177–187. PMID: 9176557.


31. Livne E, Laufer D, Blumenfeld I. Comparison of in vitro response to growth hormone by chondrocytes from mandibular condyle cartilage of young and old mice. Calcif Tissue Int. 1997; 61(1):62–67. PMID: 9192516.
Figure 1
Strategy for induction of chronic constipation using Lop. After the first injection of Lop (4 mg/kg) for 4 days, ICR mice were allowed to rest for 3 days. Two different concentrations (4 and 8 mg/kg) of Lop were then subcutaneously injected into three groups of ICR mice to produce constipated mice.
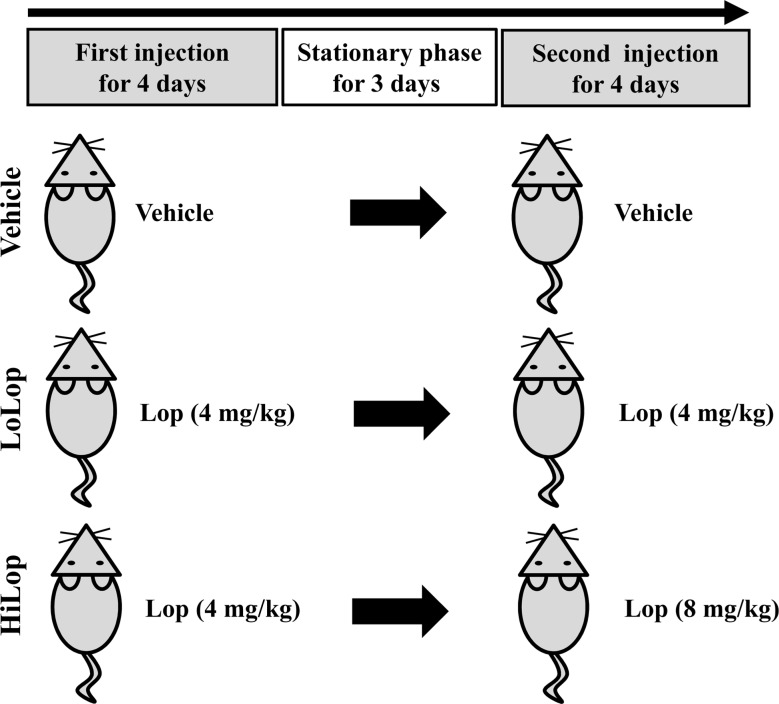
Figure 2
Alteration of the number and morphology of stools in three ICR groups after Lop injection. (A) The number of stools were observed during two Lop injection phases and one stationary phase in Vehicle, LoLop and HiLop treated mice. Numbers were counted three times and data represent the means±SD of three replicates. *, P<0.05 compared to the Korl:ICR group. (B) Following the final treatment, total stools were collected from metabolic cages and their morphology was observed.
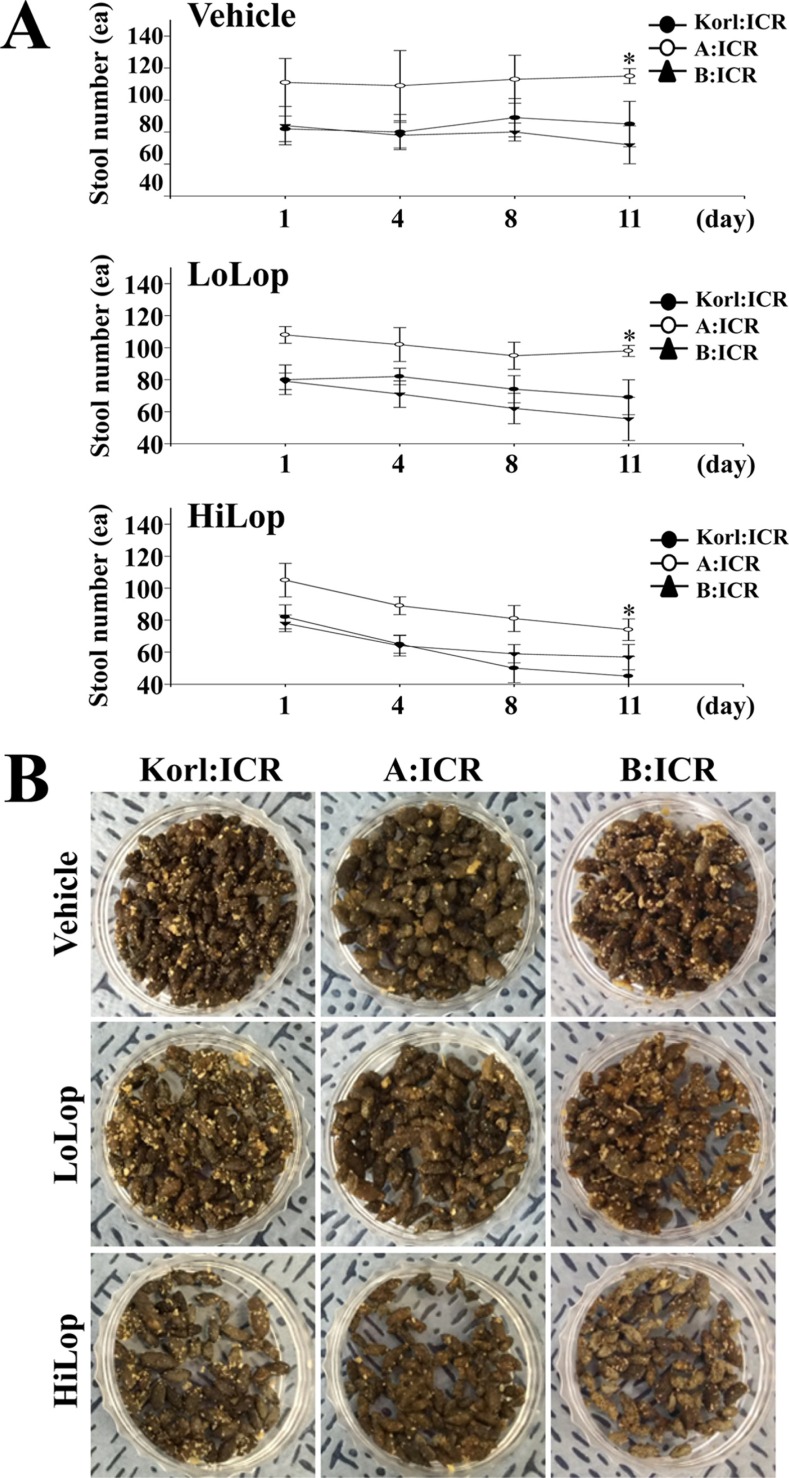
Figure 3
Alteration of histopathological structure in Lop-induced constipated ICR mice. H&E stained sections of transverse colons rats from the Vehicle treated group, LoLop treated group or HiLop treated group were observed at 200× magnification using a light microscope. The length of the mucosa layer and muscle thickness in each tissue were measured using Leica Application Suite. Data represent the means±SD of three replicates. *, P<0.05 compared to the Korl:ICR group.
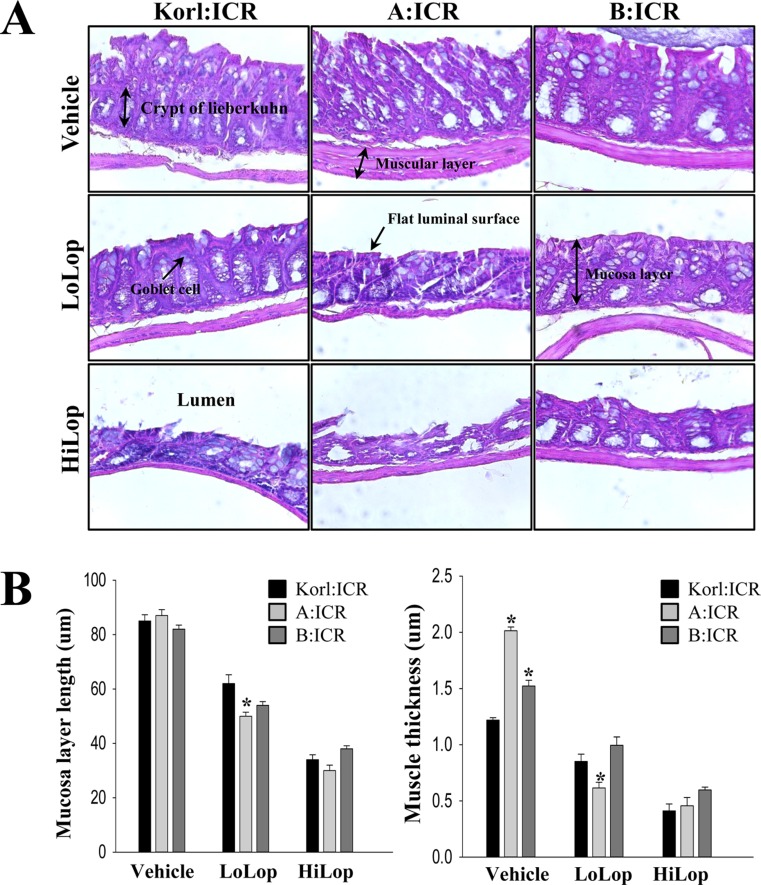
Figure 4
Mucin secretion in the transverse colon of Lop-induced constipated ICR mice. Mucin secreted from crypt layer cells was stained with alcian blue at pH 2.5 and their morphology were observed at 200× magnification. Five to six rats per group were assayed in triplicate by alcian blue staining.

Figure 5
Alteration of the level of Gα expression and PKC phosphorylation. The Gα expression and PKC phosphorylation within opioid-receptor downstream signaling pathway was measured by Western blotting using HRP-labeled anti-rabbit IgG antibody. After the intensity of each band was determined using an imaging densitometer, the relative levels of protein were calculated based on the intensity of actin protein. Data represent the means±SD of three replicates. *, P<0.05 compared to the Korl:ICR group.
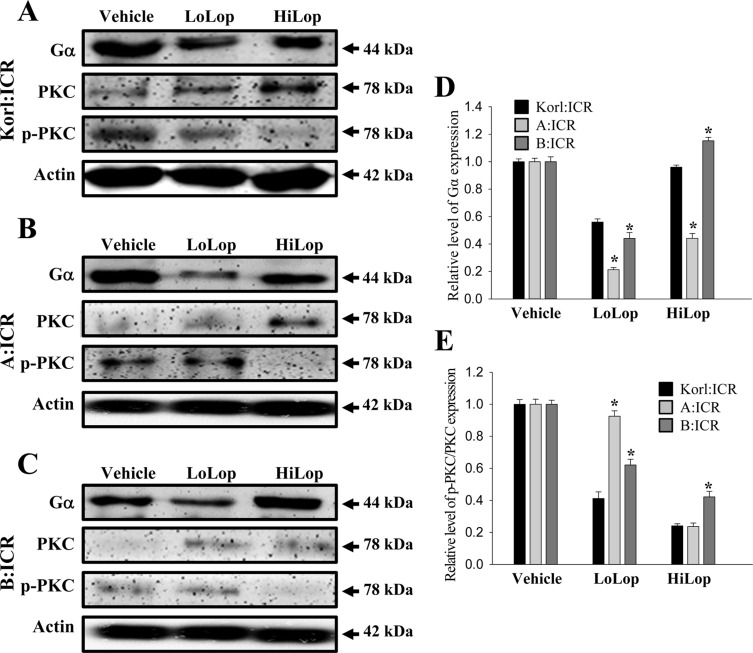
Table 1
Alteration on the excretion parameters after Lop administration using two different methods

Table 2
Measurement of body weight, feeding behavior, stool and urine secretion in Lop-induced constipated ICR mice
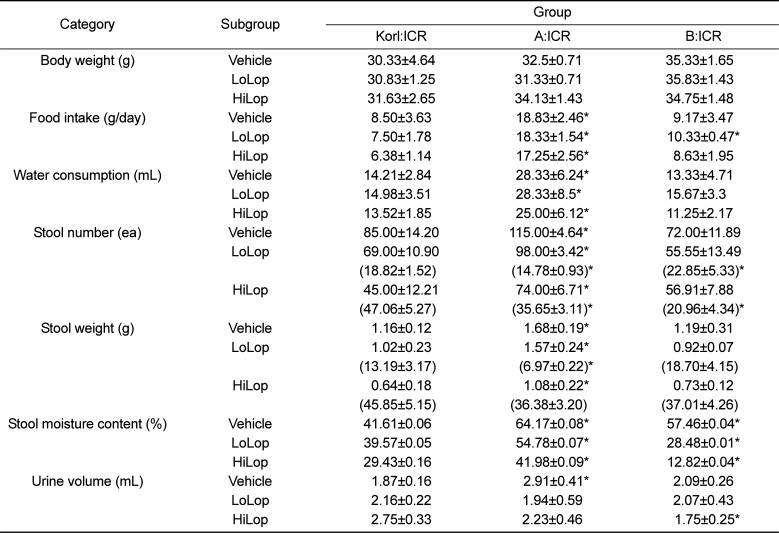