Abstract
Acknowledgments
References





















Figure 1
Alterations in serum lipid profiles in rats treated with FRG. TG, triglyceride; TC, total cholesterol; LDL-C, low density lipoproteincholesterol; HDL-C, high density lipoprotein-cholesterol. Data shown are mean±S.E.M. (n=5). *Mean values were significantly different from NC group: P<0.05. #Mean values were significantly different from HFC group: P<0.05.
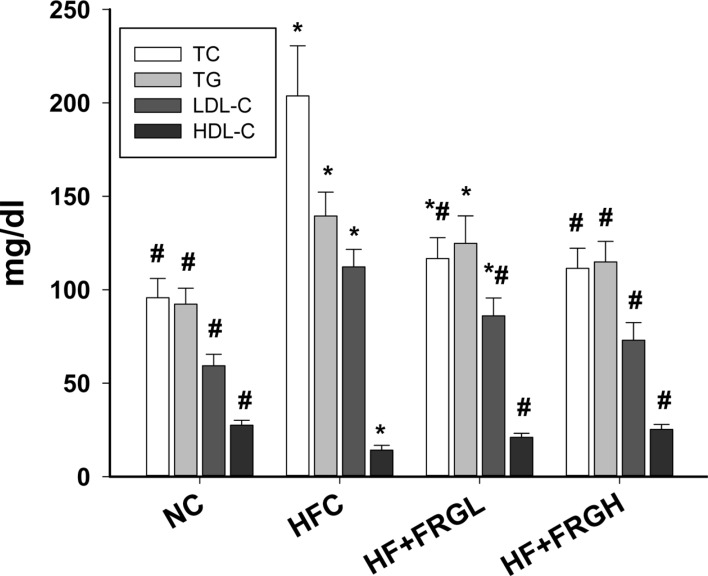
Figure 2
Alterations in hepatic malondialdehyde (MDA) level in rats treated with FRG. Data shown are mean±S.E.M. (n=5). *Mean values were significantly different from NC group: P<0.05. #Mean values were significantly different from HFC group: P<0.05.
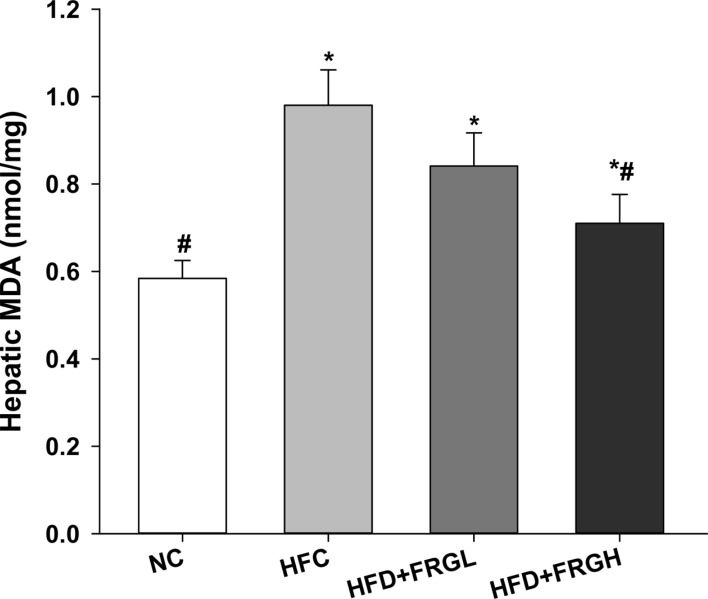
Figure 3
Histopathological examination of the rat liver tissues in hyperlipidemic rats treated with fermented red ginseng (FRG). Tissues were stained with hematoxylin and eosin. The liver section was stained using hematoxylin and eosin at 200×. NC, normal control; HFC, high fat diet control, HF+FRGL: high fat diet+FRG 0.5%, HF+FRGH: high fat diet+FRG 1.0%
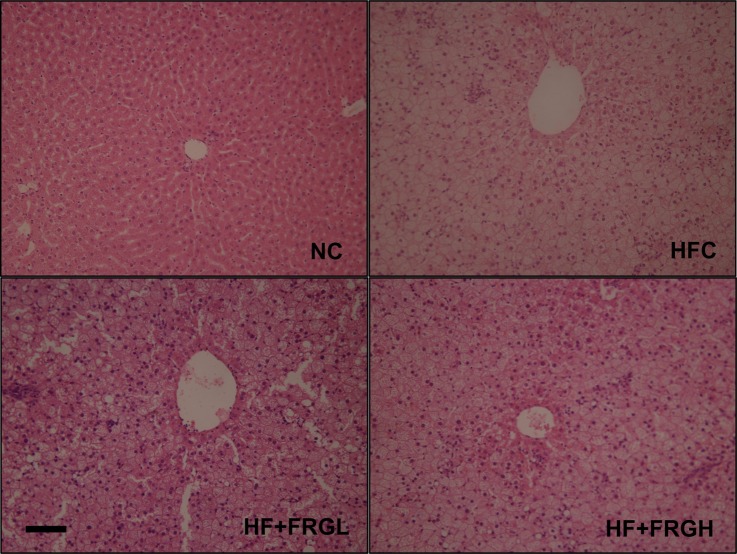
Table 1
The body weight gain and food efficiency ratio in rats treated with FRG
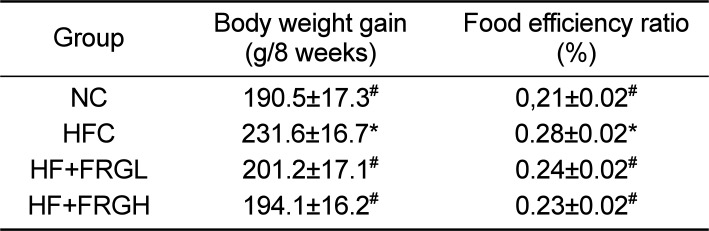
Group | Body weight gain (g/8 weeks) | Food efficiency ratio (%) |
---|---|---|
NC | 190.5±17.3# | 0,21±0.02# |
HFC | 231.6±16.7* | 0.28±0.02* |
HF+FRGL | 201.2±17.1# | 0.24±0.02# |
HF+FRGH | 194.1±16.2# | 0.23±0.02# |
All values are mean SD of 7 rats for each group.
NC: normal control. HFC: high fat diet control, HF+FRGL: high fat diet+FRG 0.5%, HF+FRGH: high fat diet+FRG 1.0%
Food efficiency ratio; body weight gain/food intake.
*Mean values were significantly different from NC group: P<0.05.
#Mean values were significantly different from HFC group: P<0.05.
Table 2
The levels of serum ALT and AST in rats treated with FRG
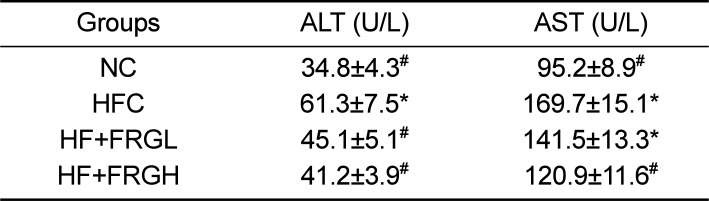
Group | ALT (U/L) | AST (U/L) |
---|---|---|
NC | 34.8±4.3# | 95.2±8.9# |
HFC | 61.3±7.5* | 169.7±15.1* |
HF+FRGL | 45.1±5.1# | 141.5±13.3* |
HF+FRGH | 41.2±3.9# | 120.9±11.6# |
All values are mean SD of 7 rats for each group.
NC: normal control. HFC: high fat diet control, HF+FRGL: high fat diet+FRG 0.5%, HF+FRGH: high fat diet+FRG 1.0%
ALT: alanine aminotransferase, AST: aspartate aminotransferase
*Mean values were significantly different from NC group: P<0.05.
#Mean values were significantly different from HFC group: P<0.05.
Table 3
The hepatic antioxidant enzyme activities in hyperlipidemic rats treated with FRG
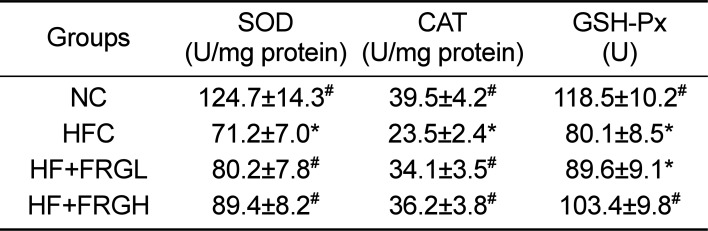
All values are mean SD of 7 rats for each group.
NC: normal control. HFC: high fat diet control, HF+FRGL: high fat diet+FRG 0.5%, HF+FRGH: high fat diet+FRG 1.0%
ALT: alanine aminotransferase, AST: aspartate aminotransferase, GLU: glucose
*Mean values were significantly different from NC group: P<0.05.
#Mean values were significantly different from HFC group: P<0.05.