This article has been
cited by other articles in ScienceCentral.
Abstract
Artificial corneas have been developed as an alternative to natural donor tissue to replace damaged or diseased corneas. This study was conducted to evaluate the stability and biocompatibility of PHEMA-PMMA [poly (2-hydroxyl methacrylate)-poly (methyl methacrylate)] keratoprostheses in rabbits following penetrating keratoplasty. Sixteen male New Zealand White rabbits aged 16 weeks were divided into three groups. Group I and group II contained six rabbits each, while the control group had four rabbits. Experimental surgery was conducted under general anesthesia. The cornea was penetrated using an 8 mm diameter biopsy punch. In group I (core 5 mm & skirt 3 mm) and group II (core 6 mm & skirt 2 mm), the keratoprosthesis was placed into the recipient full thickness bed and sutured into position with double-layer continuous. In the control group, corneal transplantation using normal allogenic corneal tissue was performed with the same suture method. After four and eight weeks, keratoprosthesis devices were evaluated by histopathological analysis of gross lesions. Post-operative complications were observed, such as extrusion and infection in experimental groups. Most corneas were maintained in the defect site by double-layer continuous suture materials for 4 weeks and kept good light transmission. However, most artificial cornea were extruded before 8 weeks. Overall, combined PHEMA and PMMA appears to have sufficient advantages for production of artificial corneas because of its optical transparency, flexibility and other mechanical features. However, the stability and biocompatibility were not sufficient to enable application in humans and animals at the present time using penetrating keratoplasty. Further studies are essential to improve the stability and biocompatibility with or without other types of keratoplasty.
Go to :

Keywords: Keratoprosthesis, cornea, PHEMA-PMMA, rabbit
More than 10 million people suffer from corneal origin blindness throughout the world, making it the second most common eye affliction next to cataracts [
23422]. Corneal blindness is caused by various diseases such as ulceration, trauma, vitamin A deficiency, keratitis, dry eye syndrome, and chemical burns [
3]. In most forms of corneal blindness, the successful alternative for visual regain is corneal transplant using donor tissue [
10]. However, there are several risk factors, and corneal transplants are not always possible due to limitations in the storage and supply of corneal tissue [
15]. In veterinary medicine, the loss of corneal transparency may occur in response to various diseases such as corneal pigmentation, chronic superficial keratitis, severe stromal edema or fibrosis, keratoconjunctivitis sicca, and ulceration. Long-term medical or surgical therapies can be applied for non-treated cases despite initial treatment [
7]. In such cases, the only alternative treatment has been the surgical application of an artificial cornea (keratoprosthesis) to restore vision [
9]. The first implantation of an artificial cornea in humans was attempted in 1859 [
2]. Keratoprostheses have been made of glass, plastics, quartz or soft rubbers. Recently, carboform, hydrogels and synthetic polymers have been introduced as materials for keratoprostheses [
1115]. The poly (methyl methacrylate) (PMMA) based keratoprosthesis was the first polymer to be used for artificial corneal transplantation [
2415]. Since then, keratoprostheses have been improved significantly, although the complication and failure rates remain high. The biocompatibility between artificial cornea and surrounding cornea tissue has also been found to be poor [
4]. The most common complications associated with keratoprostheses are implant extrusion, infection, aqueous leakage, corneal melting, epithelial down growth, glaucoma and reduced visual acuity [
18,
19]. To reduce the risk of complications, it is important to improve biocompatibility and cell adhesion [
5].
Newly developed keratoprostheses based on poly (2-hydroxyethyl methacrylate) (PHEMA)-PMMA have been evaluated to minimize the risk of complications. PMMA-based materials have continued to be the most commonly used compounds for keratoprostheses since 1941. PHEMA is a non-toxic polymer of the toxic monomer HEMA, which was the first hydrogel used in a biomedical device [
1517]. PHEMA, which has been utilized as a soft contact lens material since the late 1950s, has a high water content, mechanical strength and biocompatibility [
13]. PHEMA-PMMA based artificial corneal had more strength the tensile intensity of keratoprostheses. PHEMA-PMMA keratoprostheses have a core and skirt design, in which the core allows transmission of light and the skirt allows integration with surrounding host tissue.
This study was conducted to evaluate the stability and biocompatibility of newly developed PHEMA-PMMA keratoprostheses with two different skirt sizes based on the penetrating method in rabbits for veterinary and human medicine.
Materials and Methods
Animals
Sixteen male New Zealand White rabbits aged 16 weeks and weighing 2.6 to 3.1 kg were used in this animal experiment. Rabbits were housed in a single cage at the laboratory animal research center of Chungbuk National University and maintained on a 12/12 hour light/dark cycle, standard laboratory diet and water. The experimental protocol was approved by the institutional animal care and use committee of the laboratory animal research center of Chungbuk National University.
PHEMA-PMMA keratoprosthesis
The PHEMA-PMMA based artificial cornea (
Figure 1) consists of two parts, a central “core” optical component and a peripheral tissue-integral “skirt”. The thickness of the artificial cornea is 0.45 mm and the diameter of the core is about 5.5 mm.
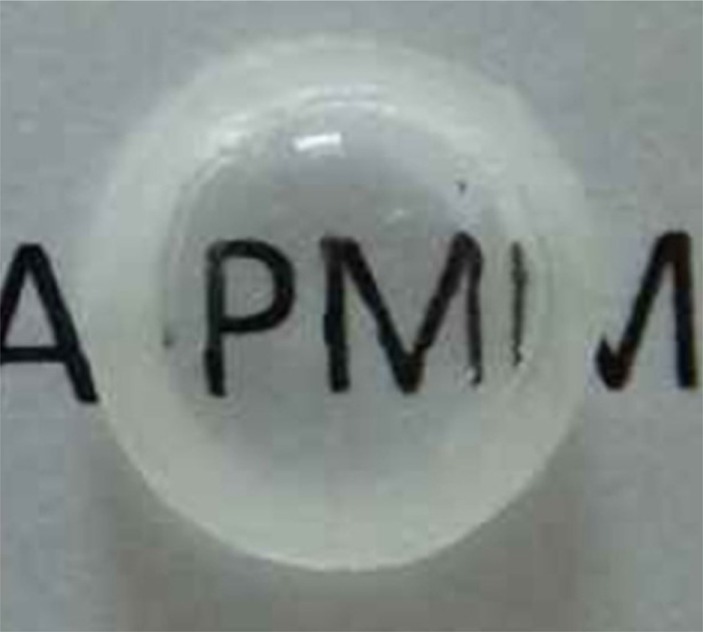 | Figure 1Gross photographs of PHEMA-PMMA artificial cornea (front view). Artificial cornea with a diameter of core is 5 mm, with 0.45 mm thick using in group I.
|
Experimental design
Sixteen rabbits were divided into three groups: group I; PHEMA-PMMA based artificial cornea with a core diameter of about 5 mm and a 3 mm skirt implanted in 6 rabbits: group II; PHEMA-PMMA based artificial cornea with a core diameter of about 6 mm and a 2 mm skirt was implanted in 6 rabbits: control group; normal allogenic corneal transplantation was performed in 4 rabbits.
All surgical procedures were performed using an operating microscope (Zeiss OPMI pico, Carl Zeiss, Goettingen, Germany). The rabbits were anesthetized using tiletamine-zolazepam (Zoletil 50, Virbac Laboratories, Carros, France) at a dose of 10 to 20 mg/kg and xylazine hydrochloride (Rompun, Bayer Korea, Seoul, Korea) at a dose of 5 to 10 mg/kg intra-muscular (IM) injection. Proparacaine hydrochloride (Alcaine 0.5%, Alcon Laboratories, Rijksweg, Puurs, Belgium) was used for topical anesthesia. After dilation of the pupil using 1% tropicamide (Ocutropic, Samil Pharma, Seoul, Korea), full-thickness corneal trephination was performed with 8 mm diameter biopsy punch and the corneal button was removed using 360 degree dissection with scissors (
Figure 2).
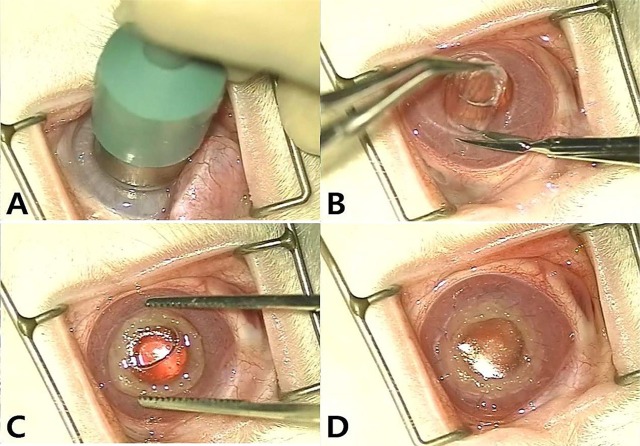 | Figure 2Creation of the recipient corneal pocket and keratoprosthesis. A. The eye is proptosed by means of gentle pressure while an 8 mm biopsy punch is used to create corneal pocket. B. Corneal button remove using 360 degree dissection with scissors. C. The devices are placed into the recipient corneal pocket. D. Double-layer continuous suture is performed using 9-0 polyglactin.
|
In the experimental groups, after complete excision of the cornea the PHEMA-PMMA based device was put into a corneal bed and artificial corneas were fixed 360 degrees to the surrounding cornea with 9-0 polyglactin (Vicryl, Ethicon, New Jersey, USA) sutures. Double-layer continuous sutures were placed between the remaining cornea and the skirt. In the control group, after the corneal pocket was created the normal allogenic cornea from the experimental group was put into a corneal bed. Double-layer continuous sutures with 9-0 polyglactin (Vicryl) were placed between the allogenic device and the corneal bed.
Following transplantation, blepharorrhaphy was performed to protect the surgical area. Antibiotic (Cefazolin; Chongkundang, Seoul, Korea; 30 mg/kg), and analgesic (Fluximine; Bomac Laboratories, Auckland, New Zealand; 1.1 mg/kg) were injected IM into each rabbit once a day for 2 weeks. Postoperatively, topical dexamethasone with polymyxin B sulfate and neomycin sulfate (Forus; Samil, Seoul, Korea) and ofloxacin (Ocuflox; Samil, Seoul, Korea) ophthalmic drops were administered once a day for 2 weeks.
Gross lesion observation and scoring
Gross lesion evaluation and scoring was performed at 4 and 8 weeks after surgery. Gross lesions were assigned a score of 1 to 5 depending on extrusion of the device and infection of the surgical area, where; 1 was extrusion and infection; 2 partial extrusion and infection; 3 partial extrusion and minimal infection; 4 no extrusion and minimal infection; and 5 no complications. Data of complete extrusion artificial cornea were removed from gross observation, therefore gross lesion scorings of each experimental group were evaluated at 4 weeks. For scoring at 8 weeks, four samples were used for observation and obtained from both group I and II, because of 5 artificial corneas were extruded completely.
Histological examination
Rabbits were sacrificed 4 weeks and 8 weeks postimplantation. The animals were anesthetized by IM injection of 20mg/kg tiletamine-zolazepam, then sacrificed. After sacrifice, the device-inserted eyes were enucleated and fixed in 10% buffered formaldehyde. Specimens were then cut along the sagittal plane and embedded in paraffin. Sections were cut into 4 µm and hematoxylin and eosin was used for staining.
Statistical analysis
The gross lesion scores were expressed as the means ±standard error and analyzed using SPSS version 20 (SPSS Inc., Chicago, IL, USA). Groups were compared by ANOVA followed by Tukey's test. P values <0.05 were considered to indicate significance.
Go to :

Results
Post-operative complications
Post-operative complications including death, extrusion of the device, infection and melting were observed in the experimental groups. There were no complications observed in the control group.
Gross lesion and scoring
In the experimental groups, most corneas were maintained in the defect site by suture materials for 4 weeks and kept good light transmission. However, all artificial corneas were partially extruded before 8 weeks. In the control group, allogenic corneas were maintained well for 8 weeks and vascularization was identified on the transplanted corneas (
Figure 3).
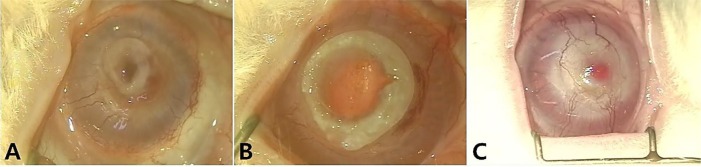 | Figure 3Gross photographs of the group I, II and control group at 8 weeks after implantation. A: Group I, partial extrusion of device is observed. B: Group II, partial extrusion of device is observed. C: Control group, There are no extrusion of implanted allogenic cornea and no stromal melting. A vascularization to the cornea was observed.
|
The gross lesion score of each group and score of each experimental period are presented in
Table 1. In group I (n=5), group II (n=4) at 4 weeks, the gross lesion score was 3.00±1.36 and 3.33±1.33, respectively. No significant differences were observed in the gross lesion scores between groups. The gross lesion score in control group (n=4) was 3.83±0.94, while score was 2.33±1.30 in experimental group at 8 weeks post-operation (n=4). The gross lesion score in experimental group at 8 weeks was significantly lower than those of experimental groups at 4 weeks and control group at 8 weeks (
P<0.05).
Table 1
Gross lesion scoring of each group and each
experimental period
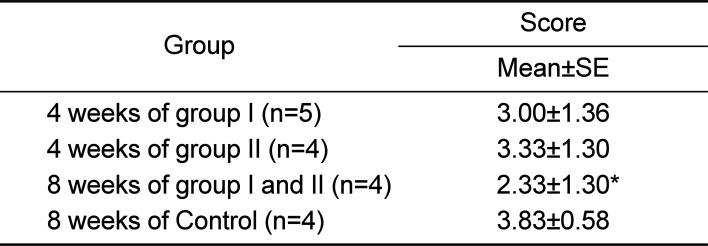
Group |
Score |
Mean±SE |
4 weeks of group I (n=5) |
3.00±1.36 |
4 weeks of group II (n=4) |
3.33±1.30 |
8 weeks of group I and II (n=4) |
2.33±1.30*
|
8 weeks of Control (n=4) |
3.83±0.58 |

Histological findings
In the experimental groups, complications such as inflammation of the surgical area, separation of the skirt and biological cornea due to artificial corneal extrusion, and partial regeneration of the corneal tissue were observed. In the control group, the epithelium, stroma and proliferation of the endothelium were found (
Figure 4).
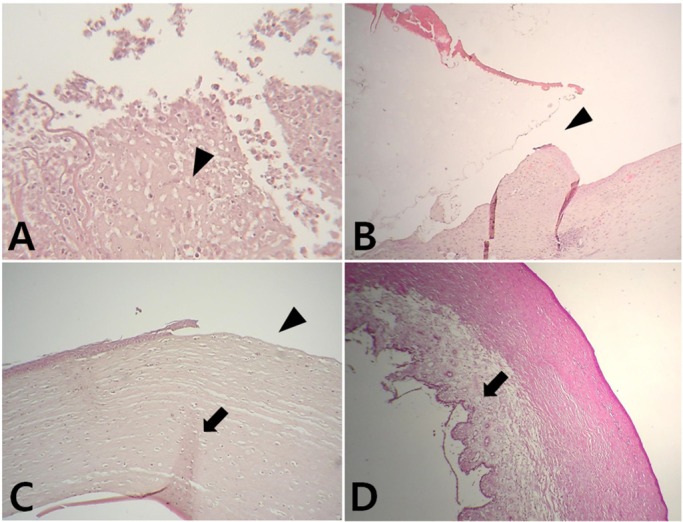 | Figure 4A: Light microscope photographs of an abnormal cornea that has post-operative infection (black arrow head). Hematoxylin and eosin staining (H&E), ×400. B: Light microscope photographs of the experimental group that has partial extrusion of the device. A separation between a skirt and corneal bed is found (black arrow head). H&E, ×100. C: Light microscope photographs of the experimental group that has complete extrusion of the device after 10 days post-operation. Regeneration of the stroma is shown (black arrow), but continuity of the epithelium is observed lost (black arrow head). H&E, ×100. D: Light microscope photographs of the control group. It shows a corneal structure as normal cornea which includes epithelium, stroma, and proliferated endothelium (black arrow). H&E, ×40.
|
Go to :

Discussion
Loss of vision can be the final result of chronic corneal disease and ulceration in both humans and animals. However, these problems cannot always be prevented or treated by general medical and surgical therapies [
23]. In such cases, allogenic corneal transplantation (keratoplasty) may be an option to regain vision. However, transplantation has disadvantages including a high-risk of surgical failure, shortage of donor corneas, difficulties in storage, and potential for disease transmission [
14]. Moreover, a previous study found that only 20% of grafts remained clear for 5 years [
21]. Because of these problems, researchers have designed and encouraged the development of artificial corneas (keratoprosthesis) to replace the keratoplasty. The ideal keratoprosthesis would utilize an epithelized material that could be implanted as in keratoplasty [
6]. While various keratoprosthesis materials and techniques have been developed, many complications such as extrusion, melting and infection have been reported. To reduce the risk of complications, keratoprosthesis should be stable and biocompatible.
PMMA has been the primary material used for keratoprosthesis since the early 1950s. This material has transparency, high mechanical strength, and is easy to process [
21]. PHEMA is widely used in biomaterials, including soft contact lenses, because of its flexibility and high water content. Moreover, this material makes the composite core-and-skirt keratoprosthesis possible [
6].
The single interrupted suture and double-layer continuous suture methods are commonly used in artificial cornea transplant or keratoplasty [
121620]. Extrusion of the device was observed frequently in the single interrupted suture method than in the double-layer continuous suture in our pre-experimental study (data not shown). Based on these results, the double-layer continuous suture method should be used for all procedures. However, single continuous suturing has become the preferred method because of its greater safety compared with the interrupted suturing. According to previous reports, multiple interrupted sutures can disrupt the even distribution of corneal tension [
20].
Overall, three of the animals (3/16, 18%) died after implantation. Additionally, post-operative inflammation was observed in one rabbit from group I (1/16, 6%). Extrusions of artificial cornea were found in most of the animals in the experimental group. Most corneas were well maintained in the recipient site by suture materials for 4 weeks; however, all artificial corneas were extruded before 8 weeks. There was no extrusion of allogenic cornea in the control group. Overall, the results of this study indicate that the biocompatibility of the PHEMA-PMMA keratoprosthesis is not sufficient to enable combination with the host tissue during penetrating keratoplasty. A gross lesion score was applied according to the degree of inflammation and extrusion of the device, which are the most important considerations in post keratoplasty evaluation. When compared with the gross lesion scores of each group, there were no significant differences between the experimental groups. However, the gross lesion scores were significantly lower at 8 weeks after artificial cornea implantation than control. These findings were likely because of the extrusion of the most devices at 8 weeks post-operation. Unexpected cornea healing was also found in three rabbits in the experimental groups. It is assumed for cornea to be healed followed by the inner layer of device before the extrusion of them for 8 weeks.
To evaluate histological changes, we used hematoxylin and eosin stain. Post-operative infection, complete extrusion of the artificial cornea and partial regeneration of the corneal tissue after device extrusion were observed upon histological evaluation. No cell adhesions from the host tissue to the skirt of the artificial cornea were observed. These findings indicate that the devices could not be attached to the recipient host tissue. There was no difference in skirt size of the devices because separation of the core and skirt was not confirmed in this study.
Combined PHEMA and PMMA appears to have sufficient advantages for production of artificial corneas because of its optical transparency, flexibility and other mechanical features. However, the stability and bio-compatibility were not sufficient to enable application in humans and animals at the present time using penetrating keratoplasty. Further studies are essential to improve the stability and biocompatibility with or without other types of keratoplasty; however, PHEMA-PMMA based keratoprostheses will likely be used commonly in the future.
Go to :
