Abstract
A dysfunction of endoplasmic reticulum (ER) stress response can result in various diseases, including cancer, inflammation, diabetes and neurodegenerative disorders. To investigate whether ER stress response can play an essential role in the induction and treatment of chronic constipation, alterations in the key parameters for ER stress were measured in loperamide (Lop) induced constipation Sprague Dawley (SD) rats treated with aqueous extracts of Liriope platyphylla (AEtLP), which has been shown to have a laxative effect. Symptoms of chronic constipation including alteration of stool parameters and the transverse colon's structure were successfully induced by Lop treatment. Laxative effects such as enhancement of stools parameters, recovery of the mucosa thickness, increased muscle thickness and recovery of flat luminal surface were also observed in the Lop+AEtLP treated group. Furthermore, enhancement of eukaryotic initiation factor 2 alpha (eIF2α) phosphorylation and inositol-requiring enzyme 1 beta (IRE1β) expression, key indicators for ER stress, that were observed in the Lop+vehicle treated group were significantly recovered in the Lop+AEtLP treated group, although the phosphorylation level of c-Jun N-terminal protein kinase (JNK) remained constant. Moreover, alterations in the transcription level of the marker genes X-box binding protein 1 (XBP-1) and growth arrest and DNA damage-inducible protein (GADD34) were similar to those of eIF2α and IRE1β. However, their level was slightly or completely recovered after AEtLP treatment. Overall, this study provides the first evidence that ER stress response may be tightly correlated with chronic constipation induced by Lop treatment, as well as the laxative effects of AEtLP.
The ER is a vital multifunctional organelle that is responsible for protein synthesis and folding, lipid biosynthesis and calcium storage [1]. These functions of the ER can be impacted and disturbed by intracellular alterations (calcium and redox imbalance), microenvironmental conditions (hypoxia, hypoglycemia and acidosis), high-fat and high-sugar diet, various natural compounds (thapsigargin and tunicamycin), and prescription drugs (bortezomib, celecoxib and nelfinavir) [2], resulting in ER stress. The process of protein folding in the ER lumen is known to be impaired by ER stress; accordingly, the accumulation of unfolded or misfolded proteins leads to the activation of specific cellular process known as the unfolded protein response (UPR) [3]. Furthermore, ER stress response observed in mammals consists of four major mechanisms including attenuation of protein synthesis, induction of ER chaperone genes, increased ER-associated degradation (ERAD) ability and induction of apoptosis to safely dispose of cells injured by ER stress [4].
Abnormal ER stress response can be induced by genetic mutation, aging and pathological conditions derived from various disease conditions such as diabetes, inflammation, atherosclerosis, liver disease, cancer, Alzheimer's disease and Parkinson's disease [456]. The condition of these diseases is tightly associated with interference of ER homeostasis, which gives rise to chronic activation of the UPR, contributing to the pathogenesis of many diseases [6]. Several compounds with the ability to ameliorate ER stress have been reported. Specifically, treatment with chemical chaperones [7], inducers of chaperone activity [8], benzodiazepines [9], inhibitors of eIF2α phosphatase [10], antioxidants [11], inducers of antioxidant pathways [12] and stress kinase inhibitors [13] induced several anti-ER stress effects such as increased protein folding capacity and activity of chaperones, as well as reduced oxidative stress and inhibition of pro-apoptotic signaling. However, no studies have provided scientific evidence of the correlation between ER stress response and chronic constipation, even though these responses are considered key factors in various neurodegenerative diseases.
Therefore, the present study was conducted to verify whether chronic constipation accompanies ER stress response in a Lop-induced constipation model. Moreover, we investigated the role of ER stress response on the laxative effects of AEtLP. Our results suggest the ER stress response is correlated with chronic constipation, as well as laxative effects in a constipated animal model.
AEtLP was prepared as previously described [14]. The roots of L. platyphylla were collected from plantations in the Miryang area of Korea in June of 2011 and identified by Dr. Cha Shin Woo at the Herbal Crop Research Division, National Institute of Horticultural & Herbal Science. Their voucher specimens (WPC-11-010) were deposited at the Functional Materials Bank of the PNU-Wellbeing RIS Center in Pusan National University. For preparation, the roots were dried in a hot-air drier (JSR Instruments, Uttaranchal, India) at 60℃. Next, 600 g of dry roots were reduced to powder using an electric blender, then extracted in 2 L of distilled water, after which the water extract was purified at 100℃ for 2 h using circulating extraction equipment (IKA Labortechnik, Staufen, Germany). The calories and main composition of AEtLP were measured in previous studies [1516]. Extract solutions were concentrated into dry pellets using a rotary evaporator (EYELA, Tokyo, Japan), then stored at -80℃ until use.
The animal protocol used in this study was reviewed and approved based on the ethical procedures for scientific care by the Pusan National University-Institutional Animal Care and Use Committee (PNU-IACUC; Approval Number PNU-2012-0010). Adult SD rats were purchased from SamTacho (Osan, Korea) and handled at the Pusan National University Laboratory Animal Resources Center accredited by the Korea Food and Drug Administration (FDA) in accordance with the Laboratory Animal Act (Accredited Unit Number-000231) and AAALAC International according to the National Institutes of Health guidelines (Accredited Unit Number; 001525). All rats were provided with standard irradiated chow (Purina Mills, Seoungnam, Korea) ad libitum and were maintained in a specific pathogen-free state under a strict light cycle (lights on at 06:00 h and off at 18:00 h) at 22±2℃ and a relative humidity of 50±10%.
The model for constipation was made as previously described (14). Briefly, 8-week-old SD rats (n=21) were assigned to either a non-constipation group (n=7) or a constipation group (n=14). SD rats in the constipation group received subcutaneous injection of Lop (4 mg/kg weight) in 0.9% sodium chloride twice a day for 3 days, whereas the non-constipation group was injected with 0.9% sodium chloride alone. The constipation group was further divided into a Lop+vehicle treated group (n=7) and Lop+AEtLP treated group (n=7). The Lop+vehicle treated group received a consistent volume of water via gavage, whereas the Lop+AEtLP treated group received 15 µL/g body weight of AEtLP (1,000 mg/kg weight) one time after the induction of constipation. At 24 h after the AEtLP and vehicle treatment, all animals were sacrificed using CO2 gas and tissue samples were acquired and stored in Eppendorf tubes at -70℃ until assay.
Alterations in food intake, water intake, and body weight were measured daily at 10:00 am throughout the experimental period using an electrical balance and a measuring cylinder. All measurements were performed three times to ensure accuracy.
All SD rats in the subset group were bred in metabolic cages during the experimental period to avoid contamination. The stools and urine excreted from each SD rat were collected at 10:00 am. Stool weight was weighed three times per sample using an electric balance, whereas their water content was determined as the difference between the wet and dry weights of the stool as previously described [1718]. Changes in urine volume were measured three times per sample using a cylinder.
Transverse colons were prepared for histological analysis as previously described [14]. Briefly, transverse colons collected from SD rats were fixed with 10% formalin for 12 h, embedded in paraffin wax, and then sectioned into 5 µm thick slices that were stained with hematoxylin & eosin (H&E, Sigma-Aldrich, MO, USA). Morphological features of these sections were observed by light microscopy, after which the mucosa layer thickness and muscle thickness were measured using Leica Application Suite (Leica Microsystems, Switzerland).
To detect the transcript level of ER stress genes, frozen transverse colons were chopped with scissors and homogenized in RNAzol B solution (Tet-Test Inc., CS104). The concentration of isolated RNA was then determined by UV-spectroscopy. The expression of the genes was subsequently examined by RT-PCR using 5 µg of the total RNA from each tissue. Briefly, 500 ng of oligo-dT primer [Gibco BRL (18418-012)] was annealed with template RNA at 70℃ for 10min. The complementary DNA, which served as the template for subsequent amplification, was then synthesized by adding dATP, dCTP, dGTP and dTTP with 200 units of reverse transcriptase and 10 pmole of sense and antisense primers. Next, amplification was conducted by subjecting the samples to 28 cycles of 30 sec at 94℃, 30 sec at 62℃ and 45 sec at 72℃ in a Perkin-Elmer Thermal Cycler. In each case, negative-RT controls were included to differentiate the DNA and RNA products. RT-PCR was also performed using primers specific to β-actin to ensure the RNA integrity. The primer sequences used to evaluate XBP-1 expression were as follows: sense primer, 5'-AAACA GAGTA GCAGC ACAGA CTGC-3', antisense primer, 5'-CTTCC AGCTT GGCTG ATGAG GTCC-3'. Additionally, the primer sequences used to evaluate GADD34 expression were as follows: sense primer, 5'-TAGAG AGCAA GAAGT GGAGC ACACA GC-3', antisense primer, 5'-CGTCA TCTTC TTCTT CTGTG TCCTC-3'. The sequences of the β-actin sense and antisense primers were 5'-TGGAA TCCTG TGGCA TCCAT GAAAC-3' and 5'-TAAAA CGCAG CTCAG TAACA GTCCG-3', respectively. The level of the PCR products was quantified using a Kodak Electrophoresis Documentation and Analysis System 120 and 1% agarose gels.
To analyze ER stress marker proteins, total proteins collected from the transverse colons of No, Lop+ vehicle and Lop+AEtLP treated rats were separated by 4-20% sodium dodecyl sulfate-polyacrylamide gel electrophoresis (SDS-PAGE) for 3 h, after which the resolved proteins were transferred to nitrocellulose membranes for 2 h at 40 V. Each membrane was subsequently incubated separately overnight at 4℃ with the following primary antibodies: eIF2α (Cell Signaling Technology Inc., Cambridge, MA, USA), p-eIF2α (Cell Signaling Technology Inc.), IRE1β (Santa Cruz Biotechnology, Santa Cruz, CA, USA), JNK (Cell Signaling Technology Inc.), p-JNK (Cell Signaling Technology Inc.) and anti-GAPDH (Cell Signaling Technology Inc.). Next, the membranes were washed with washing buffer (137 mM NaCl, 2.7 mM KCl, 10 mM Na2HPO4, 2 mM KH2PO4, and 0.05% Tween 20) and incubated with horseradish peroxidase-conjugated goat anti-rabbit IgG (Zymed Laboratories, South San Francisco, CA, USA) at a dilution of 1:1,000 and room temperature for 2 h. Finally, the membrane blots were developed using Chemiluminescence Reagent Plus kits (Pfizer, New York, NY, USA and Pharmacia, New York, NY, USA).
One-way ANOVA was used to identify significant differences between the No and Lop treated groups (SPSS for Windows, Release 10.10, Standard Version, Chicago, IL, USA). Additionally, differences between the Lop+vehicle treated group and the Lop+AEtLP treated group were evaluated by a post hoc test (SPSS for Windows, Release 10.10, Standard Version) of the variance and significance levels. All values were expressed as the means±SD. A p value of <0.05 was considered significant.
We first investigated the effects of AEtLP treatment on the body weight and feeding behaviors of Lop-induced constipation rats. Body weight and water consumption did not differ significantly among experimental groups. However, SD rats with constipation ate significantly less food (50.7%) than the non-constipation group, while there were no differences between the Lop+vehicle and Lop+AEtLP treated groups (Table 1). Overall, these results show that AEtLP treatment did not induce any significant alteration in body weight, food intake or water consumption.
To investigate the laxative effects of AEtLP on stool and urine excretion, alterations in stool parameters and urine volume were measured in No, Lop+vehicle and Lop+AEtLP treated SD rats. The number, weight and water content of stools was lower in the Lop+vehicle treated group than the No treated group. However, their level was completely recovered in the Lop+AEtLP treated group relative to the Lop+vehicle treated group (Table 1). A similar pattern was observed upon urine volume analysis. Specifically, the decrease in urine volume in the Lop+vehicle treated group was effectively recovered in the Lop+AEtLP treated group (Table 1). Taken together, the present results indicate that AEtLP treatment could improve Lop-induced constipation in SD rats through enhancement of stool and urine excretion.
To determine if improvement of constipation after AEtLP treatment was accompanied by alterations in the histological structure of the transverse colon, the mucosa layer and muscle thickness were measured in transverse colons of rats in the subset groups following H&E staining. The average thickness of the mucosa layer in the Lop+vehicle treated group was shorter than in the No treated group. Following Lop and AEtLP cotreatment, this level recovered by more than 65% when compared with the Lop+vehicle treated group (Figure 1). In addition, a similar pattern was detected in muscle thickness. In the Lop+vehicle treated group, muscle thickness was dramatically reduced when compared with that in the No treated group, while significant recovery of this level was observed in AEtLP treated group (Figure 1). These results show that AEtLP treatment can alleviate the histopathological structure of chronic constipation through recovery of the mucosa layer and muscle thickness in the transverse colon of constipated SD rats.
We next investigated two issues related to ER stress, whether the chronic constipation of SD rats induced by Lop treatment can induce alterations in ER stress response, and whether the laxative effects of AEtLP are correlated with ER stress. To achieve this, the expression level of some key proteins in the ER-stress downstream signaling pathway was measured. The phosphorylation of eIF2α and expression of IRE1β was 668 and 1,206% higher in the Lop+Vehicle treated group than the No treated group. Moreover, the level of XBP-1 and GADD34 transcripts increased by 152 and 253%, respectively, in the Lop+vehicle treated group compared with the No treated group (Figures 2, 3). The present results indicate that ER stress can be successfully induced by the chronic constipation induced by Lop treatment.
Furthermore, expression of the above marker proteins was dramatically recovered in the Lop+AEtLP treated group, although the rate of recovery differed among groups. The greatest decrease was observed in the phosphorylation of eIF2α in the Lop+AEtLP treated group, and this level in the Lop+AEtLP treated group recovered to that in the No treated group (Figures 2, 3). Therefore, these results showed that the laxative effects of AEtLP were closely correlated with suppression of ER stress in the Lop induced constipation model.
Constipation is a chronic gastrointestinal disorder caused by various factors including insufficient dietary fiber intake, inadequate fluid intake, decreased physical activity, side effects of medication, hypothyroidism, and obstruction caused by colorectal cancer [19]. Recently, many studies have focused on investigation of the basic causes, development of novel therapeutic drugs and identification of molecular mechanisms responsible for constipation [202123]. In an effort to investigate the molecular mechanisms associated with this disease, we investigated the role of Lop as an ER stressor, as well as the correlation between ER stress response and the laxative effect of L. platyphylla. The results indicate that ER stress response can play an essential role in Lop-induced constipation and the laxative effects of AEtLP in this constipation model.
Lop has been shown to improve diarrhea through inhibition of gastrointestinal motility in a morphine-like, naloxone-sensitive manner [2425]. Lop also inhibits Ca2+-induced contractions of ileal smooth muscle and acetylcholine release from cholinergic nerve terminals as a Ca2+ channel-blocker [2627]. Therefore, this chemical has great potential for use as an ER stressor because Ca2+ metabolism disruptors including A23187 and thapsigargin can be considered ER stressors [4]. However, no studies have been conducted to confirm this. Therefore, we investigated changes in key marker proteins representing ER stress in the transverse colons obtained from a Lop-induced constipation model to determine if Lop acts as an ER stressor. Our results suggest that Lop can be used to evoke ER stress in animals for experimental purposes.
Moreover, some natural products able to reduce ER stress have been considered candidates for the treatment of several diseases. For example, dilinoleoyl-phosphateidyl-ethanolamine (DLPE) from Hericium erinaceum was found to reduce ER stress dependent cell death in the mouse neuroblastoma cell line, Neuro2a [28], while Saliva sahendica suppressed ER stress induced by H2O2 via attenuation of intracellular calcium levels and decreased calpain activation [29]. In addition, ER stress response was inhibited by treatment with purple rice (Oryza sativa L.) bran extract and its constituents in tunicamycin-treated retinal ganglion cells in a study of neurodegenerative disease, as well as by Ixeris dentata in palmitate treated HepG2 hepatocytes in an investigation of liver disease [3031]. However, natural products have had the opposite effect when applied to treat cancer. Tanshinone IIA isolated from Radix Salviae miltiorrhizae suppressed cancer cell growth by stimulating ER stress such as the activation of and increase in GADD expression that occur in prostate cancer [32]. In this study, we attempted to verify the effects of L. platyphylla on the suppression of ER stress using a Lop-induced constipation model. As shown in Figures 2 and 3, the marker proteins indicating ER stress decreased significantly after AEtLP treatment. These results were very similar to those in previous studies of natural products able to suppress ER stress. Therefore, our findings provide important scientific evidence that the ER stress response can be considered a mechanism for the laxative effects of AEtLP.
Taken together, we investigated the correlation between the laxative effect of L. platyphylla and ER stress response in Lop-induced constipation SD rats. The level of several marker proteins and transcripts indicating ER stress response was increased in Lop treated constipation rats, but dramatically recovered in Lop+AEtLP treated rats. The results presented herein are the first to suggest that the laxative effects of AEtLP are closely correlated with attenuation of increasing ER stress induced by Lop treatment.
Acknowledgments
We thank Jin Hyang Hwang, the animal technician, for directing the Animal Facility and Care at the Laboratory Animal Resources Center. This research was supported by the Basic Science Research Program through the National Research Foundation of Korea (NRF) funded by the Ministry of Education (2014R1A1A2058360).
References
1. Alberts B, Johnson A, Lewis J, Raff M, Roberts K, Walter P. Molecular biology of the cell. 4th edn. New York: Garland Science;2002.
2. Schonthal AH. Endoplasmic reticulum stress: its role in disease and novel prospects for therapy. Scientifica (Cairo). 2012; 2012:857516. PMID: 24278747.
3. Ron D, Walter P. Signal integration in the endoplasmic reticulum unfolded protein response. Nat Rev Mol Cell Biol. 2007; 8(7):519–529. PMID: 17565364.


5. Forman MS, Lee VM, Trojanowski JQ. 'Unfolding' pathways in neurodegenerative disease. Trends Neurosci. 2003; 26(8):407–410. PMID: 12900170.


6. Ozcan L, Tabas I. Role of endoplasmic reticulum stress in metabolic disease and other disorders. Annu Rev Med. 2012; 63:317–328. PMID: 22248326.


7. Ozcan U, Yilmaz E, Ozcan L, Furuhashi M, Vaillancourt E, Smith RO, Görgün CZ, Hotamisligil GS. Chemical chaperones reduce ER stress and restore glucose homeostasis in a mouse model of type 2 diabetes. Science. 2006; 313(5790):1137–1140. PMID: 16931765.


8. Zhang Z, Tong N, Gong Y, Qiu Q, Yin L, Lv X, Wu X. Valproate protects the retina from endoplasmic reticulum stress-induced apoptosis after ischemia-reperfusion injury. Neurosci Lett. 2011; 504(2):88–92. PMID: 21939735.


9. Kim I, Shu CW, Xu W, Shiau CW, Grant D, Vasile S, Cosford ND, Reed JC. Chemical biology investigation of cell death pathways activated by endoplasmic reticulum stress reveals cytoprotective modulators of ASK1. J Biol Chem. 2009; 284(3):1593–1603. PMID: 19004820.


10. Boyce M, Bryant KF, Jousse C, Long K, Harding HP, Scheuner D, Kaufman RJ, Ma D, Coen DM, Ron D, Yuan J. A selective inhibitor of eIF2alpha dephosphorylation protects cells from ER stress. Science. 2005; 307(5711):935–939. PMID: 15705855.
11. Appierto V, Tiberio P, Villani MG, Cavadini E, Formelli F. PLAB induction in fenretinide-induced apoptosis of ovarian cancer cells occurs via a ROS-dependent mechanism involving ER stress and JNK activation. Carcinogenesis. 2009; 30(5):824–831. PMID: 19325135.


12. Satoh T, Okamoto SI, Cui J, Watanabe Y, Furuta K, Suzuki M, Tohyama K, Lipton SA. Activation of the Keap1/Nrf2 pathway for neuroprotection by electrophilic [correction of electrophillic] phase II inducers. Proc Natl Acad Sci U S A. 2006; 103(3):768–773. PMID: 16407140.
13. Wang XZ, Ron D. Stress-induced phosphorylation and activation of the transcription factor CHOP (GADD153) by p38 MAP kinase. Science. 1996; 272(5266):1347–1349. PMID: 8650547.


14. Kim JE, Lee YJ, Kwak MH, Ko J, Hong JT, Hwang DY. Aqueous extracts of Liriope platyphylla induced significant laxative effects on loperamide-induced constipation of SD rats. BMC Complement Altern Med. 2013; 13:333. PMID: 24274470.


15. Kim HJ, Kim J, Kim SJ, Lee SH, Park YS, Park BK, Kim BS, Kim SK, Cho SD, Jung JW, Nam JS, Choi CS, Jung JY. Anti-inflammatory effect of quercetin on picryl chloride-induced contact dermatitis in BALB/c mice. Lab Anim Res. 2010; 26(1):7–13.


16. Kim JE, Hwang IS, Choi SI, Lee HR, Lee YJ, Goo JS, Lee HS, Son HJ, Jang MJ, Lee SH, Kang BC, Hwang DY. Aqueous extract of Liriope platyphylla, a traditional Chinese medicine, significantly inhibits abdominal fat accumulation and improves glucose regulation in OLETF type II diabetes model rats. Lab Anim Res. 2012; 28(3):181–191. PMID: 23091518.
17. Wintola OA, Sunmonu TO, Afolayan AJ. The effect of Aloe ferox Mill. in the treatment of loperamide-induced constipation in Wistar rats. BMC Gastroenterol. 2010; 10:95. PMID: 20723249.


18. Lee HY, Kim JH, Jeung HW, Lee CU, Kim DS, Li B, Lee GH, Sung MS, Ha KC, Back HI, Kim SY, Park SH, Oh MR, Kim MG, Jeon JY, Im YJ, Hwang MH, So BO, Shin SJ, Yoo WH, Kim HR, Chae HJ, Chae SW. Effects of Ficus carica paste on loperamide-induced constipation in rats. Food Chem Toxicol. 2012; 50(3-4):895–902. PMID: 22178225.
19. Leung FW. Etiologic factors of chronic constipation: review of the scientific evidence. Dig Dis Sci. 2007; 52(2):313–316. PMID: 17219073.
20. Kim JE, Lee YJ, Kwak MH, Jun G, Koh EK, Song SH, Seong JE, Kim JW, Kim KB, Kim S, Hwang DY. Metabolomics approach to serum biomarker for loperamide-induced constipation in SD rats. Lab Anim Res. 2014; 30(1):35–43. PMID: 24707303.


21. Carrington EV, Evers J, Grossi U, Dinning PG, Scott SM, O'Connell PR, Jones JF, Knowles CH. A systematic review of sacral nerve stimulation mechanisms in the treatment of fecal incontinence and constipation. Neurogastroenterol Motil. 2014; 26(9):1222–1237. PMID: 25167953.


22. Gyawali B, Hayashi N, Tsukuura H, Honda K, Shimokata T, Ando Y. Opioid-induced constipation. Scand J Gastroenterol. 2015; 50(11):1331–1338. PMID: 26061717.


23. Li Y, Yu Z, Xu B. Mechanism progress on enteric nervous system of acupuncture for slow transit constipation. Zhongguo Zhen Jiu. 2015; 35(3):309–312. PMID: 26062213.
24. Awouters F, Niemegeers CJ, Janssen PA. Pharmacology of antidiarrheal drugs. Annu Rev Pharmacol Toxicol. 1983; 23:279–301. PMID: 6307123.


25. Church J, Fletcher EJ, Abdel-Hamid K, MacDonald JF. Loperamide blocks high-voltage-activated calcium channels and N-methyl-D-aspartate-evoked responses in rat and mouse cultured hippocampal pyramidal neurons. Mol Pharmacol. 1994; 45(4):747–757. PMID: 8183255.
26. Reynolds IJ, Gould RJ, Snyder SH. Loperamide: blockade of calcium channels as a mechanism for antidiarrheal effects. J Pharmacol Exp Ther. 1984; 231(3):628–632. PMID: 6502516.
27. Burleigh DE. Opioid and non-opioid actions of loperamide on cholinergic nerve function in human isolated colon. Eur J Pharmacol. 1988; 152(1-2):39–46. PMID: 2850201.


28. Nagai K, Chiba A, Nishino T, Kubota T, Kawagishi H. Dilinoleoyl-phosphatidylethanolamine from Hericium erinaceum protects against ER stress-dependent Neuro2a cell death via protein kinase C pathway. J Nutr Biochem. 2006; 17(8):525–530. PMID: 16426828.


29. Shaerzadeh F, Alamdary SZ, Esmaeili MA, Sarvestani NN, Khodagholi F. Neuroprotective effect of Salvia sahendica is mediated by restoration of mitochondrial function and inhibition of endoplasmic reticulum stress. Neurochem Res. 2011; 36(12):2216–2226. PMID: 21769643.
30. Tanaka J, Nakanishi T, Shimoda H, Nakamura S, Tsuruma K, Shimazawa M, Matsuda H, Yoshikawa M, Hara H. Purple rice extract and its constituents suppress endoplasmic reticulum stress-induced retinal damage in vitro and in vivo. Life Sci. 2013; 92(1):17–25. PMID: 23123597.
31. Lee J, Hong SW, Park SE, Rhee EJ, Park CY, Oh KW, Park SW, Lee WY. Exendin-4 attenuates endoplasmic reticulum stress through a SIRT1-dependent mechanism. Cell Stress Chaperones. 2014; 19(5):649–656. PMID: 24446069.


32. Chiu SC, Huang SY, Chen SP, Su CC, Chiu TL, Pang CY. Tanshinone IIA inhibits human prostate cancer cells growth by induction of endoplasmic reticulum stress in vitro and in vivo. Prostate Cancer Prostatic Dis. 2013; 16(4):315–322. PMID: 24042854.
Figure 1
Alteration of the histological structure in transverse colons of Loperamide (Lop)-induced constipated rats. Hematoxylin and eosin (H&E)-stained sections of transverse colons from the No treated group (a and d), Lop+vehicle treated group (b and e) or Lop+Liriope platyphylla (AEtLP) treated group (c and f) were observed at two different magnifications (100× and 400×) using a light microscope. (B) The thickness of the mucosa layer and muscle thickness are presented as graphs. Data represent the mean±SD from three replicates. *P<0.05 compared to the No treated group. #P<0.05 compared to the Lop+vehicle treated group.
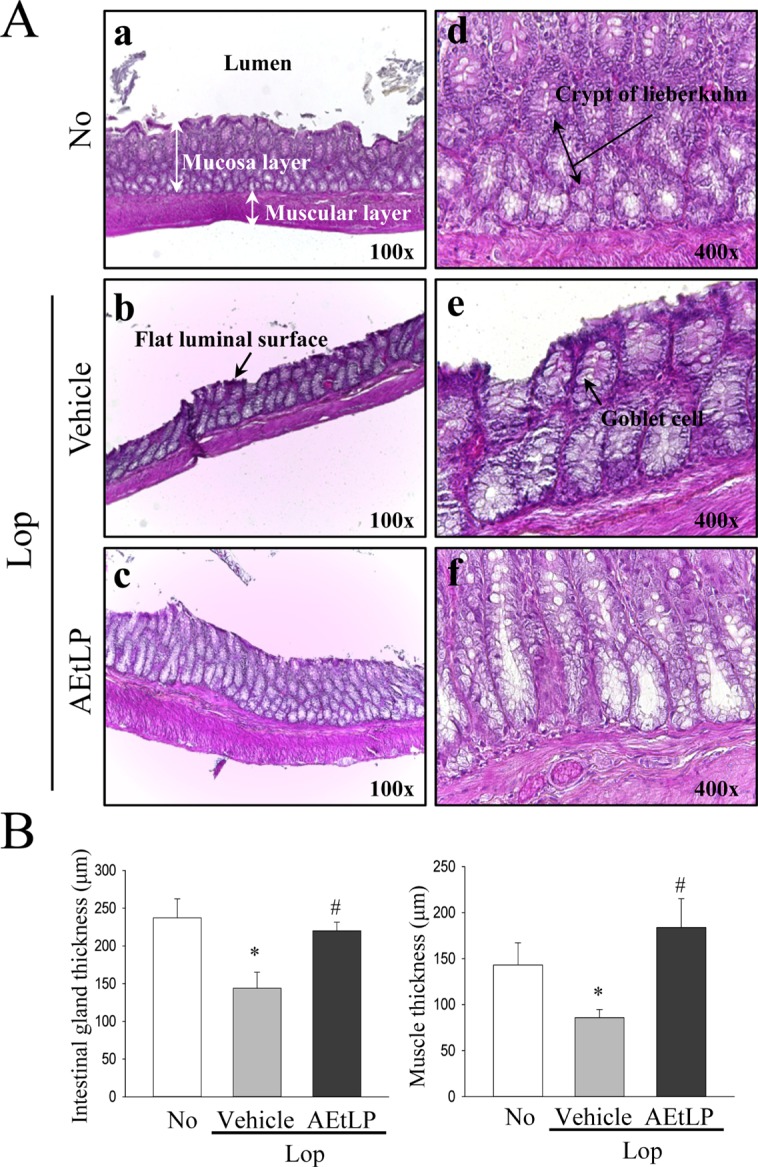
Figure 2
Expression of endoplasmic reticulum (ER) stress marker proteins. The expression levels of eukaryotic initiation factor 2 alpha (eIF2α), p-eIF2α and inositol-requiring enzyme 1 beta (IRE1β) in total proteins were detected with specific antibodies. The glyceraldehyde 3-phosphate dehydrogenase (GAPDH) level is also shown as an endogenous control. The band intensity of these proteins was determined using an imaging densitometer and the relative levels of each protein were calculated based on the intensity of GAPDH protein. Data represent the means±SD from three replicates. *P<0.05 compared to the No treated group. #P<0.05 compared to the Lop+vehicle treated group.
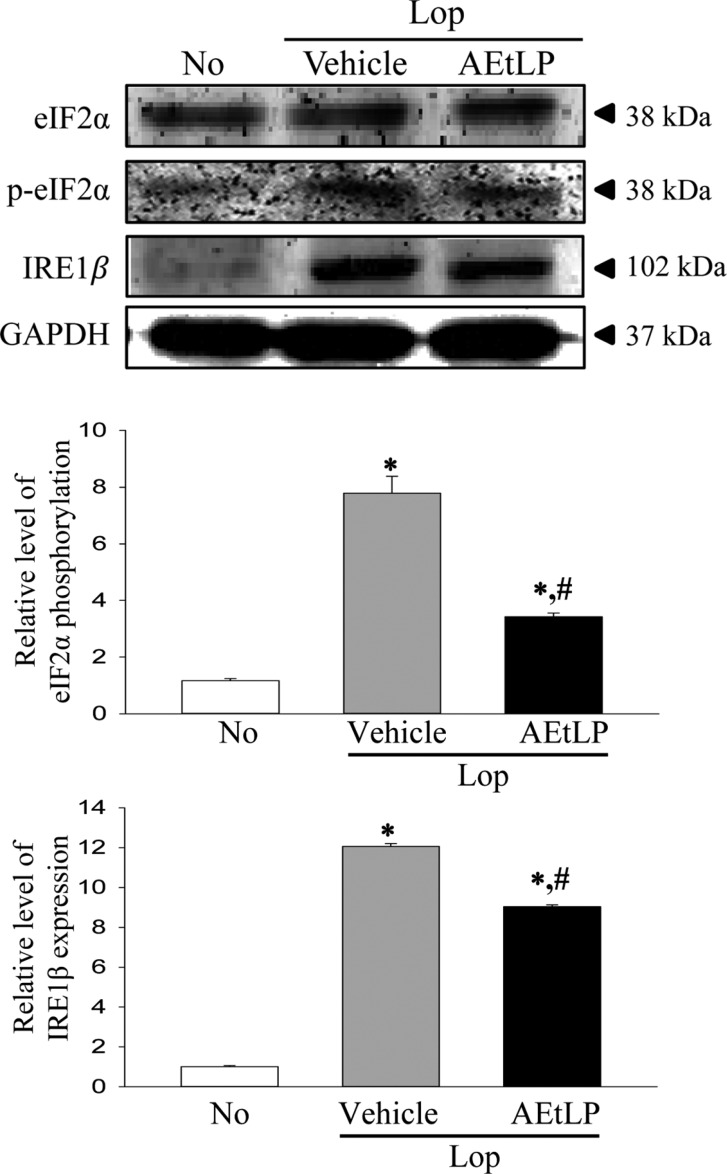
Figure 3
Transcript levels of ER stress marker genes. The levels of X-box binding protein 1 (XBP-1) and growth arrest and DNA damage-inducible protein (GADD34) transcripts in total mRNA of transverse colons were measured by RT-PCR using specific primers. After the intensity of each band was determined using an imaging densitometer, the relative levels of XBP-1 and GADD34 transcripts were calculated based on the intensity of actin transcripts. Five to six rats per group were assayed in triplicate by RT-PCR assays. Data represent the means±SD of three replicates. *P<0.05 compared to the No treated group. #P<0.05 compared to the Lop+Vehicle treated group.
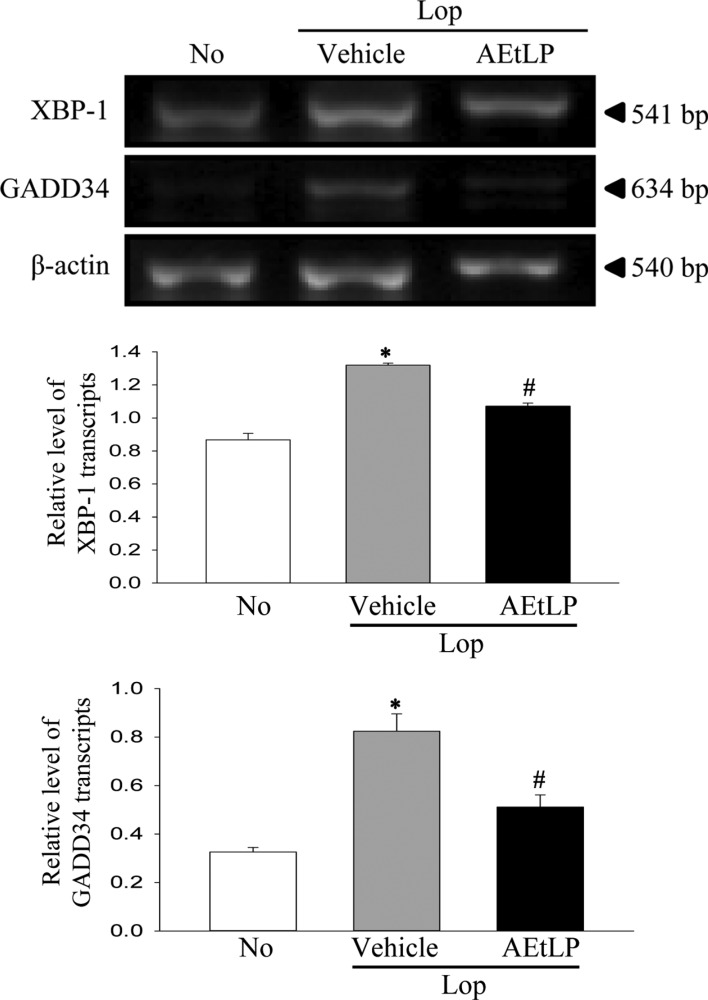
Table 1
Measurement of body weight, feeding behavior, stools and urine secretion in Lop-induced constipated SD rats
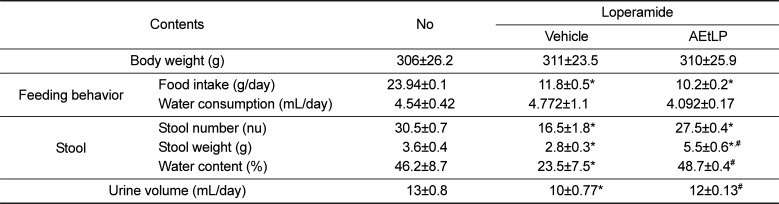