Abstract
Inflammatory bowel disease is a chronic inflammatory disorder occurring in the gastrointestinal track. However, the efficacy of current therapeutic strategies has been limited and accompanied by side effects. In order to eliminate the limitations, herbal medicines have recently been developed for treatment of IBD. Peuraria Lobata (Peuraria L.) is one of the traditional herbal medicines that have anti-inflammatory effects. Bioavailability of Peuraria L., which is rich in isoflavones, is lower than that of their fermented forms. In this study, we generated fermented Peuraria L. extracts (fPue) and investigated the role of fPue in inflammation and intestinal barrier function in vitro and in vivo. As the mice or intestinal epithelial cells were treated with DSS/fPue, mRNA expression of pro-inflammatory cytokines was reduced and the architecture and expression of tight junction proteins were recovered, compared to the DSS-treated group. In summary, fPue treatment resulted in amelioration of DSS-induced inflammation in the colon, and the disrupted intestinal barrier was recovered as the expression and architecture of tight junction proteins were retrieved. These results suggest that use of fPue could be a new therapeutic strategy for treatment of IBD.
Inflammatory bowel disease (IBD), including ulcerative colitis (UC) and Crohn's disease (CD), are autoimmune diseases, characterized by chronic inflammation in the gastrointestinal (GI) tract, and recent reports indicated that the incidence and prevalence of IBD have increased worldwide [1]. Studies on pathogenesis of IBD have reported various factors that cause both types of IBD, including genetic susceptibility, environmental factors, infectious agents, and abnormality of immune system function [2]. IBD is accompanied by other diseases such as psychiatric disorders [34] and colon cancer development [56].
In the pathogenesis of IBD, the expression and secretion of pro-inflammatory cytokines, such as tumor necrosis factor-α (TNFα) and interleukin-1β (IL-1β), are increased and the expression of cytokines is regulated by nuclear factor-kappa B (NF-κB) [7]. In addition, dysfunction of the gastrointestinal barrier is a major step in the pathogenesis of IBD [8910]. The major components forming the gastrointestinal barrier are tight junction (TJ) proteins in the apical junction complex, which regulate paracellular permeability. It has been reported that the structure and function of TJ proteins are disrupted in IBD patients [11], which leads to intestinal bacterial translocalization into mesenteric lymph nodes [12].
There are several therapeutic strategies for remission of IBD, including use of anti-inflammatory medicines and immunomodulators, however, their clinical efficacy has been limited and accompanied by side effects, including fluid retention, insomnia and vomiting [13]. The overall remission rate of IBD patients with therapies is only approximately 50% [1415]. Among the therapeutic strategies, targeting the adoptive immune system, has been the most common for treatment of IBD, such as monoclonal antibodies against TNFα [1316]. However, treatment with the agents is successful only in less than one third of IBD patients and a proportion of patients experience loss of response to the agents [141718]. Therefore, development of new therapeutic strategies for treatment of IBD is needed.
Complementary and alternative medicines (CAMs) have recently been developed for treatment of IBD and, the use of herbal medicines is the most common for treatment of IBD [1920]. Herbal medicines have a number of beneficial effects, including anti-bacterial, anti-oxidant, and anti-inflammatory effects [19]. Pueraria L., a common Chinese herbal medicine, also has various beneficial effects, including anti-diarrhetic and antiemetic effects, as well as an anti-oxidant effect [2122]. Pueraria L. is rich in isoflavones, including peurarin, daidzein and genistein [21]. Isoflavones are known for their anti-inflammatory effect and anti-oxidant effect [23]. However, bioavailability of isoflavones is lower than that of their fermented forms, isoflavone aglycones [24].
In this study, for development of more effective herbal medicine for treatment of IBD, we generated the fermented Pueraria L. extract (fPue) and compared its anti-inflammatory effect with that of Pueraria L. extract (Pue). In addition, in vivo experiments were performed on a dextran sulfate sodium (DSS)-induced colitis model, which mimics human ulcerative colitis [25].
For generation of fPue, Pueraria L. was purchased from Omniherb Inc.; 400 g of that was crushed and boiled in 4 L of water at 121℃ for 30 minutes and the aqueous extract was filtered using Whatman filter paper 2 (GE Healthcare, Little Chalfont, United Kingdom). Dextrose (2%) and yeast extract (1%) were autoclaved and added to the Pue extract with 1% Lactobacillus brevis, aD110T, and the Pue extract was incubated at 30℃ for 5 days. Following incubation, the Pue extract was centrifuged at 12,000 rpm for 15 minutes, and the supernatant was filtered. The filtered extract was concentrated at 40℃ and 80 rpm, using a rotary evaporator (Eyela, Irvine, CA).
Specific pathogen-free female ICR mice (5 weeks old) were purchased from Orient Inc., Gyeonggi-do, Korea and the mice were acclimatized for 1 week prior to the experiment. All mice were maintained under 12 hours light/dark cycles at 22±5℃ and 60±5% humidity and provided with food (Orient Inc.) and water ad libitum. The information about components and calorie of the food is supported by Orient Inc. All experiments with animals were approved by the Institutional Animal Care and Use Committee at Lee Gil Ya Cancer and Diabetes Institute (IACUC No., LCDI-2014-0010).
Raw264.7 and Caco-2 cell lines were purchased from Korea Cell Line Bank, Seoul, Korea. The cell lines were cultured in 10% FBS-supplemented DMEM (WelGene, Daegu, Korea) with streptomycin (100 µg/mL)/penicillin (100 units/mL) at 37℃ in a humidified chamber with 5% CO2. Raw264.7 and Caco-2 cells were treated with Pue or fPue for western blot, RT-PCR and immunofluorescence analysis. For detection of NF-κB signaling by western blot, Raw264.7 cells were treated with Pue or fPue (0, 25, 100, 400 µg/mL) for 4 hours, followed by treatment with lipopolysccharide (LPS) for 1 hour. Total protein was isolated and western blot was performed. For determination of transcriptional level of cytokines in Raw264.7 cells, the cells were treated with Pue or fPue (0, 25, 100, 400 µg/mL) for 4 hours, followed by treatment with LPS for 3 hours. Total RNA was isolated using TriZol (Invitrogen, Waltham, MA) and RT-PCR was performed. Caco-2 cells were seeded in 12-well plates and cultured with or without a cover slip for immunofluorescence assay or western blot, respectively. The cells were incubated until they become 100% confluent, followed by incubation for an additional 7 days. The cells were treated with fPue (0, 25, 100, or 400 µg/mL) for 48 hours, followed by treatment with 2% DSS for 24 hours. Then, immunofluorescence assay and membrane fractionation for western blot were performed.
The mice were divided into 3 groups (7 mice in each group): (1) no treatment group; (2) 5% DSS-treated group; (3) 5% DSS with fPue (100 mg/kg)-treated group. DSS (MP Biomedical, Burlingame, CA) and fPue were dissolved in distilled water. Five percent DSS was administered to the mice in the drinking water for 7 days and normal distilled water was then supplied for 4 days for recovery (Figure 2A). During the recovery period, the mice were treated with fPue, or distilled water daily by gavage (Figure 2A). At the end of the recovery period, disease activity index (DAI) score was determined as described in the previous report [26] and necropsy was then performed.
The mice were sacrificed at the end of the recovery period. Following excision of the whole colon from anus to cecum, the length was measured. Approximately 0.5 cm of the distal colon was excised and frozen in liquid nitrogen for RT-PCR and histological analysis, and the remaining colon was Swiss-rolled and fixed in 10% formalin. The tissue samples were then paraffin-embedded and sectioned at 5 µm-thickness. The sections were stained with hematoxylin and eosin to evaluate the severity of colitis, according to three parameters described in the previous report [26]. The severity of colitis was scored blindly by 2 researchers.
Twenty micrograms of each protein sample was used for western blot analysis. The primary antibodies are as followed: anti-rabbit ZO-1, anti-mouse Occludin, 1:1,000, anti-mouse Claudin-1 (Invitrogen, Waltham, MA); anti-rabbit p-P65 (Cell Signaling, Danvers, MA), anti-rabbit P65, anti-goat Lamin B (Santa Cruz, CA) and anti-mouse GAPDH (Merck Millipore, Billerica, MA).
Using 3 µg of RNA, cDNA was synthesized using the PrimeScript RT reagent Kit (TaKaRa, Shiga, Japan) as described in the manufacturer's instructions. RT-PCR was performed, using the primers listed on Supplementary Table 1 (Table S1).
The 5 µm-thick frozen colon tissue and cells on the slides were fixed in an acetone-methanol (1:1) mixture at -20℃. The slides were incubated with primary antibodies (anti-rabbit ZO-1, anti-mouse Occludin, anti-mouse Claudin-1; Invitrogen, Waltham, MA) overnight at 4℃ and secondary antibodies (anti-rabbit IgG FITC, Santa Cruz; Alexa Fluor 488 goat anti-mouse IgG; Invitrogen) for 2 hours at room temperature. After mounting the slides with VectaShield Mounting Medium with DAPI (Vector Laboratories, Burlingame, CA), the slides were sealed with nail polish and stored at -20℃ until observation, using a confocal microscope (Nikon Instruments Inc., Melville, NY).
Numerical data for all graphs were expressed as mean±standard deviation. Statistical changes in all data were determined using one-way analysis of variance (ANOVA). Scheffe was used for the post hoc test. The significance level was limited to 5% (P<0.05). SPSS 22.0 was used as a tool for statistical analysis.
To determine whether fPue can enhance anti-inflammatory effect, compared to Pue, the activity of P65, a subunit of NF-κB, was examined in murine macrophage cell line Raw264.7. The cells were treated with Pue or fPue, followed by activation by LPS, which induces phosphorylation of P65 in Raw264.7 cells. Results of western blot showed that treatment with fPue significantly inhibited phosphorylation of P65 while Pue slightly inhibited that of P65 (Figure 1A). Activation of NF-κB leads to upregulated transcription of pro-inflammatory cytokines, including IL-1β, TNFα, IL-6, and IL-12. Treatment of LPS resulted in increased transcriptional levels of IL-1β, TNFα, IL-6 and IL-12 (Figure 1B). On the other hand, when pre-treated with fPue, the expression levels of the cytokines were decreased while treatment with Pue did not affect them (Figure 1B). These results suggest that fPue would improve the anti-inflammatory effect.
Because fPue exerted an anti-inflammatory effect in Raw264.7 cell line (Figure 1), we examined whether fPue could improve DSS-induced colitis. DSS-induced colitis is characterized by disruption of epithelial structure and infiltration of inflammatory cells and loss of goblet cells [27]. To evaluate the effect of fPue on DSS-induced colitis, DAI was assessed by fecal analysis, to examine how much water and blood the fecal pellet contains and histological analysis was performed by H&E staining on the paraffin block sections to assess the severity of colitis. In the control group, DAI score was nearly 0 and that of the DSS-treated group showed a significant increase (Figure 2B), indicating that colitis was induced by DSS. On the other hand, DAI score of the DSS+fPue-treated group was significantly decreased, suggesting that fPue treatment improved the colonic function (Figure 2B). Histological analysis demonstrated that DSS treatment induced severe inflammation in mucosa, edema in submucosa and epithelial cell loss while they were significantly lower in the DSS+fPue-treated group, compared with the DSS+Vehicle-treated group (Figure 2C, 2D). In addition, alcian blue staining showed that while goblet cells were lost in the DSS-treated group, they were restored by fPue treatment (Figure 2E). These results suggest that treatment with fPue resulted in amelioration of colonic inflammation and promoted recovery of epithelial structure in the colonic mucosa, following recovery from diarrhea.
In a DSS-induced colitis model, inflammatory cells, mainly macrophages, are infiltrated into submucosa and lamina propria of the inflamed colon [1628]. To determine whether the number of infiltrated macrophages is decreased by treatment with fPue, immunostaining was performed for F4/80, a marker for macrophages, on frozen sections of colon tissue. Immunofluorescence analysis revealed that while the number of F4/80-positive cells present in mucosa and submucosa of the inflamed colon was significantly increased, that of F4/80-positive cells was markedly reduced in the colon of the DSS+fPue-treated group (Figure 3A, B), indicating that macrophage infiltration was reduced by fPue treatment. In addition, decrease in transcriptional expression of pro-inflammatory mediators, IL-1β, TNFα and IL-6 was observed by performing RT-PCR (Figure 3C).
As previously mentioned, epithelial dysfunction is a major aspect in the pathogenesis of IBD. To determine whether the epithelial barrier, disrupted in DSS-induced colitis, is recovered by treatment with fPue, the expression levels and the arrangement of ZO-1, Claudin1 and Occludin, were examined by immunofluorescence assay, using cryosections of colon tissue. As shown in Figure 4A, all three TJ proteins were expressed along with the inner lining of the columnar epithelium of normal colon tissue. While the expression levels of TJ proteins were decreased and their architecture was disrupted in DSS-induced colitis, they were recovered as DSS-induced colitis was ameliorated by fPue treatment (Figure 4A). In addition, to investigate whether the physiological function of the epithelial barrier was recovered, bacterial translocalization was examined by blood agar assay, using cells from mesenteric lymph nodes. As shown in Figure 4B and C, bacterial translocalization was induced as the epithelial barrier was disrupted by DSS treatment. On the other hand, in the DSS+fPue-treated group a decreased number of bacteria was present in the lymph node (Figure 4B, C), indicating that bacterial translocalization was reduced as disrupted tight proteins were rescued by fPue treatment.
To determine whether DSS-induced disruption of TJ would be recovered by fPue treatment, an in vitro experiment was performed using colonic epithelial cell line Caco-2. Caco-2 cells form a monolayer and TJ between the cells, and the TJ is disrupted by DSS treatment [29]. Consistent with the previous study, treatment of Caco-2 cells with DSS resulted in a decrease in protein expression of Occludin, which was detected by western blot, while that of ZO-1 did not change (Figure 5A). Although ZO-1 expression level was not affected by DSS, its architecture was disrupted as well as that of Occludin (Figure 5B). However, fPue treatment prevented disruption of the architecture of ZO-1 and Occludin (Figure 5B). These data suggest that fPue could have a protective effect directly on intestinal TJ.
Previous studies have reported that traditional herbal medicines are the most commonly used CAM worldwide for treatment of IBD because of their efficacy and safety [1920]. However, because not all herbal medicines are safe and they are incomplete for treatment of IBD, improvement of the safety and efficacy is necessary. The fermentation process may be a useful tool for elimination of toxicity of herbal medicines and to improve their bioavailability [24]. Even though Pueraria L. is used traditionally in treatment of diarrhea, to the best of our knowledge, in vivo experiments, using fermented form of Pue on a DSS-induced acute colitis model have not been reported. Therefore, we aimed to investigate the differences in anti-inflammatory effect between Pue and fPue in vitro and to examine the therapeutic effect of fPue in vivo, using a DSS-induced acute colitis model in mice.
NF-κB signaling pathway is the major pathway that regulates expression of pro-inflammatory cytokines such as IL-1β, TNFα, IL-6 and IL-12, which are involved in development of IBD [16]. Therefore, inhibition of pro-inflammatory cytokines may lead to remission of IBD. In Figure 1, as Raw264.7 cells were treated with fPue, followed by LPS, phosphorylation of NF-κB and the expression of pro-inflammatory cytokines were reduced, compared to cells treated with LPS only. However, the treatment with Pue had a limited anti-inflammatory effect (Figure 1), suggesting that fermented form of Pue, rather than Pue itself, has an anti-inflammatory effect.
One of the major aspects in pathogenesis of IBD is infiltration of inflammatory cells, mainly macrophages [1628], which leads to production of pro-inflammatory cytokines. In our results, histopathological analysis showed that the number of infiltrated inflammatory cells was diminished in mucosa and submucosa of colon in the DSS+fPue-treated group, compared with the DSS+Vehicle-treated group (Figure 2, 3). Consistent with in vitro data, the transcription of pro-inflammatory cytokines was suppressed by fPue treatment (Figure 3C). This reduction of cytokines may have been resulted from a reduced number of macrophages infiltrated into colonic epithelium. In vitro data shown in Figure 1 also suggest that fPue could have a suppressive effect on production of pro-inflammatory cytokines in macrophages as it inhibits NF-κB activation. However, it remains unclear how fPue reduced macrophage infiltration and its cytokine production.
Another aspect of IBD is dysfunction of gastrointestinal barrier by destruction of TJ in colonic mucosa [8910]. The gastrointestinal barrier is responsible for uptake of nutrients, ions, and water, and for preventing translocation of pathogens across tissue [11]. Our in vivo experiment demonstrated that as acute colitis was induced by DSS administration in mice, the structure of the TJ complex was disrupted (Figure 4A). In contrast, the disrupted TJ complex was restored by fPue treatment (Figure 4A). In addition, while bacterial translocalization was induced due to disrupted TJ complex, bacteria were not translocalized into mesenteric lymph nodes as the TJ complex was recovered by treatment with fPue (Figure 4B, C), indicating that the function of intestinal barrier was recovered. Interestingly, treatment with fPue inhibited collapse of TJ proteins (Figure 5), suggesting that fPue may has a direct effect on recovery of the intestinal barrier function in acute colitis by rescuing TJ protein distribution.
In conclusion, in vitro experiment with Raw264.7 suggested that fPue has a more beneficial effect on inflammatory activity than the extract itself. The anti-inflammatory effect of fPue was also demonstrated in an in vivo experiment. fPue ameliorated DSS-induced acute colitis and recovered gastrointestinal barrier function. In addition, there was no side effect in the use of fPue, such as weight loss or liver injury (data not shown). However, investigation to determine whether there are additional beneficial effects of fPue is needed. In this study, we suggest that the fPue has a therapeutic potential for treatment of IBD without undesirable effects.
Acknowledgments
This work was conducted under the industrial infrastructure program (No. N0000888) for fundamental technologies, which is funded by the Ministry of Trade, Industry, and Energy (MOTIE, Korea), and also supported by High Value-added Food Technology Development Program 114006-04, Ministry of Agriculture, Food and Rural Affairs.
References
1. Molodecky NA, Soon IS, Rabi DM, Ghali WA, Ferris M, Chernoff G, Benchimol EI, Panaccione R, Ghosh S, Barkema HW, Kaplan GG. Increasing incidence and prevalence of the inflammatory bowel diseases with time, based on systematic review. Gastroenterology. 2012; 142(1):46–54.e42. PMID: 22001864.


2. Baumgart DC, Carding SR. Inflammatory bowel disease: cause and immunobiology. Lancet. 2007; 369(9573):1627–1640. PMID: 17499605.


3. Walker JR, Ediger JP, Graff LA, Greenfeld JM, Clara I, Lix L, Rawsthorne P, Miller N, Rogala L, McPhail CM, Bernstein CN. The Manitoba IBD cohort study: a population-based study of the prevalence of lifetime and 12-month anxiety and mood disorders. Am J Gastroenterol. 2008; 103(8):1989–1997. PMID: 18796096.


4. Goodhand JR, Wahed M, Mawdsley JE, Farmer AD, Aziz Q, Rampton DS. Mood disorders in inflammatory bowel disease: relation to diagnosis, disease activity, perceived stress, and other factors. Inflamm Bowel Dis. 2012; 18(12):2301–2309. PMID: 22359369.


5. Itzkowitz SH, Yio X. Inflammation and cancer IV. Colorectal cancer in inflammatory bowel disease: the role of inflammation. Am J Physiol Gastrointest Liver Physiol. 2004; 287(1):G7–G17. PMID: 15194558.


6. Jess T, Rungoe C, Peyrin-Biroulet L. Risk of colorectal cancer in patients with ulcerative colitis: a meta-analysis of population-based cohort studies. Clin Gastroenterol Hepatol. 2012; 10(6):639–645. PMID: 22289873.


7. Reinecker HC, Steffen M, Witthoeft T, Pflueger I, Schreiber S, MacDermott RP, Raedler A. Enhanced secretion of tumour necrosis factor-alpha, IL-6, and IL-1 beta by isolated lamina propria mononuclear cells from patients with ulcerative colitis and Crohn's disease. Clin Exp Immunol. 1993; 94(1):174–181. PMID: 8403503.
8. Fries W, Renda MC, Lo Presti MA, Raso A, Orlando A, Oliva L, Giofre MR, Maggio A, Mattaliano A, Macaluso A, Cottone M. Intestinal permeability and genetic determinants in patients, first-degree relatives, and controls in a high-incidence area of Crohn's disease in Southern Italy. Am J Gastroenterol. 2005; 100(12):2730–2736. PMID: 16393227.


9. Reuter BK, Pizarro TT. Mechanisms of tight junction dysregulation in the SAMP1/YitFc model of Crohn's disease-like ileitis. Ann N Y Acad Sci. 2009; 1165:301–307. PMID: 19538320.


10. Zeissig S, Burgel N, Gunzel D, Richter J, Mankertz J, Wahnschaffe U, Kroesen AJ, Zeitz M, Fromm M, Schulzke JD. Changes in expression and distribution of claudin 2, 5 and 8 lead to discontinuous tight junctions and barrier dysfunction in active Crohn's disease. Gut. 2007; 56(1):61–72. PMID: 16822808.


11. Bruewer M, Samarin S, Nusrat A. Inflammatory bowel disease and the apical junctional complex. Ann N Y Acad Sci. 2006; 1072:242–252. PMID: 17057204.


12. Zareie M, Johnson-Henry K, Jury J, Yang PC, Ngan BY, McKay DM, Soderholm JD, Perdue MH, Sherman PM. Probiotics prevent bacterial translocation and improve intestinal barrier function in rats following chronic psychological stress. Gut. 2006; 55(11):1553–1560. PMID: 16638791.


13. Fakhoury M, Negrulj R, Mooranian A, Al-Salami H. Inflammatory bowel disease: clinical aspects and treatments. J Inflamm Res. 2014; 7:113–120. PMID: 25075198.


14. Peyrin-Biroulet L, Lemann M. Review article: remission rates achievable by current therapies for inflammatory bowel disease. Aliment Pharmacol Ther. 2011; 33(8):870–879. PMID: 21323689.


15. Clark M, Colombel JF, Feagan BC, Fedorak RN, Hanauer SB, Kamm MA, Mayer L, Regueiro C, Rutgeerts P, Sandborn WJ, Sands BE, Schreiber S, Targan S, Travis S, Vermeire S. American gastroenterological association consensus development conference on the use of biologics in the treatment of inflammatory bowel disease, June 21-23, 2006. Gastroenterology. 2007; 133(1):312–339. PMID: 17631151.


16. Neurath MF. Cytokines in inflammatory bowel disease. Nat Rev Immunol. 2014; 14(5):329–342. PMID: 24751956.


17. Gisbert JP, Panes J. Loss of response and requirement of infliximab dose intensification in Crohn's disease: a review. Am J Gastroenterol. 2009; 104(3):760–767. PMID: 19174781.


18. Billioud V, Sandborn WJ, Peyrin-Biroulet L. Loss of response and need for adalimumab dose intensification in Crohn's disease: a systematic review. Am J Gastroenterol. 2011; 106(4):674–684. PMID: 21407178.


19. Langmead L, Rampton DS. Review article: complementary and alternative therapies for inflammatory bowel disease. Aliment Pharmacol Ther. 2006; 23(3):341–349. PMID: 16422993.


20. Jackson LN, Zhou Y, Qiu S, Wang Q, Evers BM. Alternative medicine products as a novel treatment strategy for inflammatory bowel disease. Am J Chin Med. 2008; 36(5):953–965. PMID: 19051360.


21. Jun M, Hong J, Jeong WS, Ho CT. Suppression of arachidonic acid metabolism and nitric oxide formation by kudzu isoflavones in murine macrophages. Mol Nutr Food Res. 2005; 49(12):1154–1159. PMID: 16254887.


22. Bebrevska L, Foubert K, Hermans N, Chatterjee S, Van Marck E, De Meyer G, Vlietinck A, Pieters L, Apers S. In vivo antioxidative activity of a quantified Pueraria lobata root extract. J Ethnopharmacol. 2010; 127(1):112–117. PMID: 19799984.


23. Jiang RW, Lau KM, Lam HM, Yam WS, Leung LK, Choi KL, Waye MM, Mak TC, Woo KS, Fung KP. A comparative study on aqueous root extracts of Pueraria thomsonii and Pueraria lobata by antioxidant assay and HPLC fingerprint analysis. J Ethnopharmacol. 2005; 96(1-2):133–138. PMID: 15588661.


24. Izumi T, Piskula MK, Osawa S, Obata A, Tobe K, Saito M, Kataoka S, Kubota Y, Kikuchi M. Soy isoflavone aglycones are absorbed faster and in higher amounts than their glucosides in humans. J Nutr. 2000; 130(7):1695–1699. PMID: 10867038.


25. Boismenu R, Chen Y. Insights from mouse models of colitis. J Leukoc Biol. 2000; 67(3):267–278. PMID: 10733087.


26. Nishiyama Y, Kataoka T, Yamato K, Taguchi T, Yamaoka K. Suppression of dextran sulfate sodium-induced colitis in mice by radon inhalation. Mediators Inflamm. 2012; 2012:239617. PMID: 23365486.


27. Stillie R, Stadnyk AW. Role of TNF receptors, TNFR1 and TNFR2, in dextran sodium sulfate-induced colitis. Inflamm Bowel Dis. 2009; 15(10):1515–1525. PMID: 19479745.


28. Stevceva L, Pavli P, Husband AJ, Doe WF. The inflammatory infiltrate in the acute stage of the dextran sulphate sodium induced colitis: B cell response differs depending on the percentage of DSS used to induce it. BMC Clin Pathol. 2001; 1(1):3. PMID: 11580872.


29. Samak G, Chaudhry KK, Gangwar R, Narayanan D, Jaggar JH, Rao R. Calcium/Ask1/MKK7/JNK2/c-Src signalling cascade mediates disruption of intestinal epithelial tight junctions by dextran sulfate sodium. Biochem J. 2015; 465(3):503–515. PMID: 25377781.


Figure 1
Anti-inflammatory effect of Peuraria L. extract and its fermented form. A. Western blot analysis for phosphorylation of NF-κB in Raw264.7 cells after treatment of Pue or fPue with LPS. B. RT-PCR for transcriptional expression levels of pro-inflammatory cytokines in Raw264.7 cells after treatment of Pue or fPue with LPS. Pue, Pueraria Lobata aqueous extract; fPue, fermented form of Pueraria Lobata aqueous extract. Intensity of each band in western blot and RT-PCR results was quantified by using ImageJ and normalized to GAPDH (A) and β-actin (B).
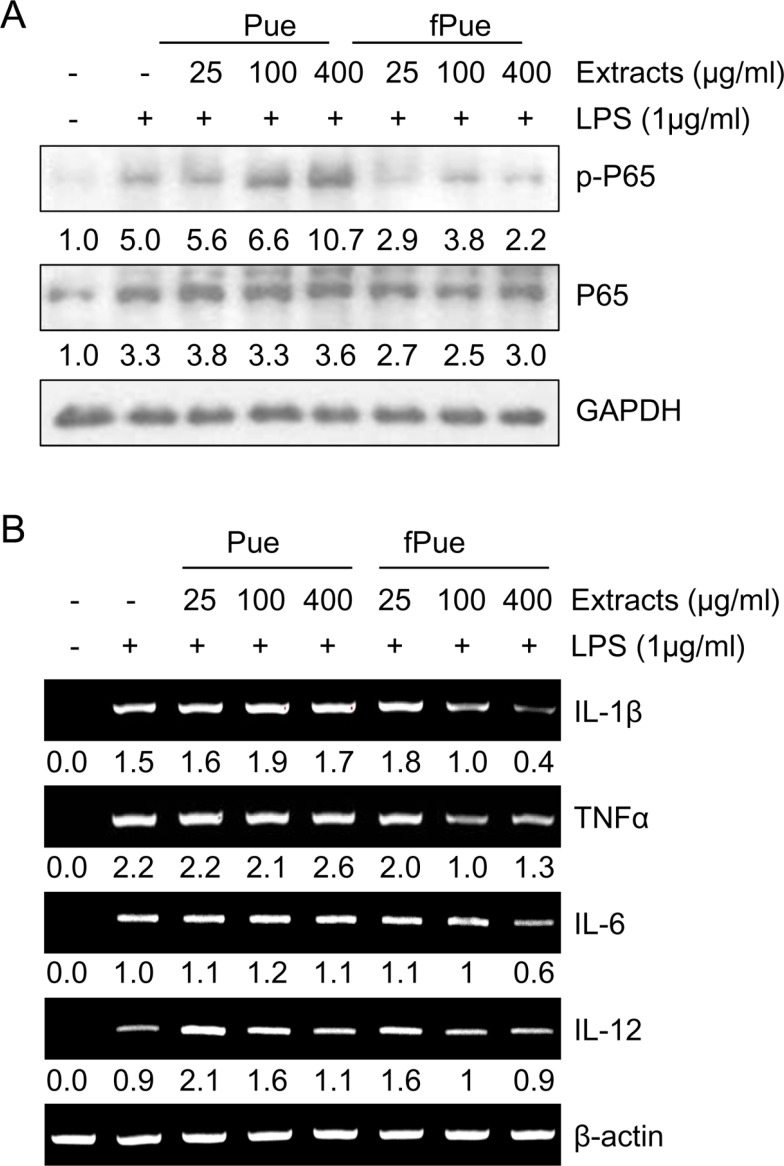
Figure 2
Effect of fPue on DSS-induced colitis in mice. A. Schedule for administration of 5% DSS and fPue. B. Assessment of disease activity index (DAI) by fecal analysis. C. Histological evaluation of DSS-induced colitis according to degree of epithelial cell loss, crypt damage, and infiltration of inflammatory cells. D. Histological figures of colon stained by H&E. (a, mucosa; b, submucosa; c, muscularis; d, crypt) E. Histological figures to observe goblet cell loss by Alcian Blue staining. Numerical data were expressed as mean±standard deviation (n=7, *P<0.05, **P<0.01, ***P<0.001). DSS, dextran sulfate sodium; fPue, fermented form of Pueraria Lobata aqueous extract; H&E, hematoxylene and eosin.

Figure 3
Infiltration of macrophages in colonic mucosa and submucosa. A. Immunofluorescence assay for F4/80, using frozen colon section (bar=50 µm). B. Quantification of number of F4/80-possitive cells per field. C. Transcriptional expression levels of pro-inflammatory cytokines, using colon tissue. Numerical data were expressed as mean ± standard deviation (n=7, *P<0.05, **P<0.01, ***P<0.001). DSS, dextran sulfate sodium; fPue, fermented form of Pueraria Lobata aqueous extract; HPF, high power field.
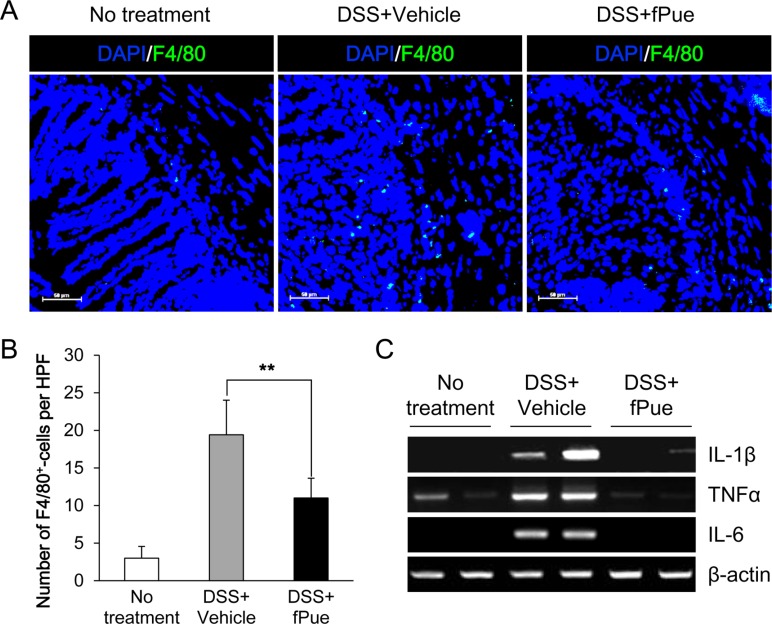
Figure 4
Effect of fPue on recovery of colonic epithelial barrier. A. Immunofluorescence assay for tight junction proteins, ZO-1, Claudin-1, and Occludin, on frozen colon tissue. B. Blood agar assay, using cells isolated from mesenteric lymph nodes. C. Representative figures for blood agar assay. Numerical data were expressed as mean ± standard deviation (n=7, *P<0.05, **P<0.01, ***P<0.001). DSS, dextran sulfate sodium; fPue, fermented form of Pueraria Lobata aqueous extract.

Figure 5
Evaluating a direct effect of fPue on recovery of tight junction proteins, using Caco-2 cells. A. Western blot analysis for expression of tight junction proteins, ZO-1 and Occludin after treatment of fPue with 2% DSS. B. Immunofluorescence assay for ZO-1 and Occludin on Caco-2 cells. DSS, dextran sulfate sodium; fPue, fermented form of Pueraria Lobata aqueous extract.
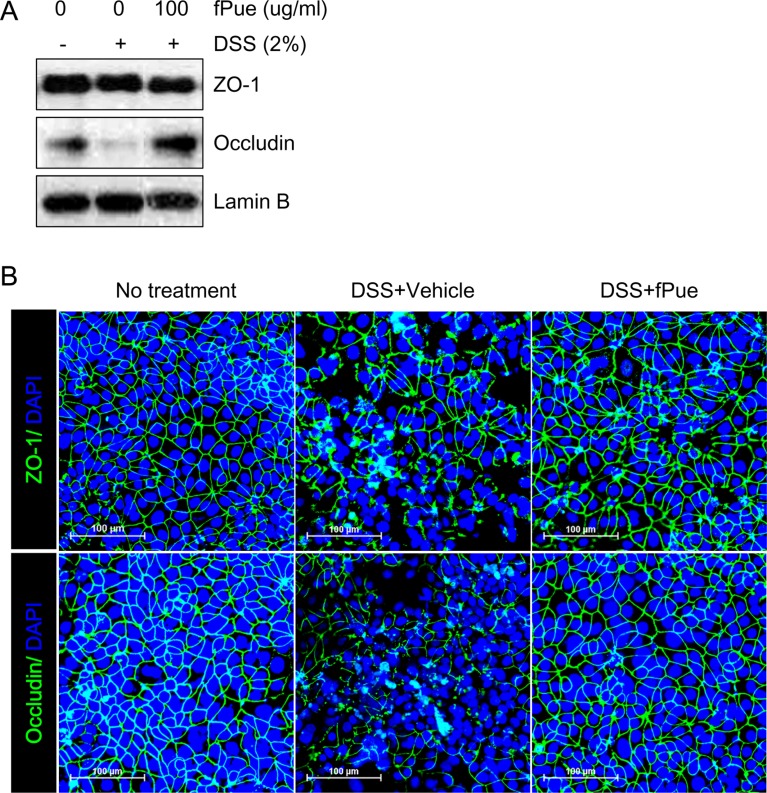