Abstract
Whereas increasing concerns about radiation exposure to nuclear disasters or side effects of anticancer radiotherapy, relatively little research for radiation damages or remedy has been done. The purpose of this study was to establish level of LD70/30 (a lethal dose for 70% of mice within 30 days) by total-body γ irradiation (TBI) in a mouse model. For this purpose, at first, 8-week-old male ICR and C57BL/6N mice from A and B companies were received high dose (10, 11, 12 Gy) TBI. After irradiation, the body weight and survival rate were monitored for 30 days consecutively. In next experiment, 5-week-old male ICR and C57BL/6N mice from B company were received same dose irradiation. Results showed that survival rate and body weight change rate in inbred C57BL/6N mice were similar between A and B company. In ICR mice, however, survival rate and body weight change rate were completely different among the companies. Significant difference of survival rate both ICR and C57BL6N mice was not observed in between 5-week-old and 8-week-old groups receiving 10 or 12 Gy TBI. Our results indicate that the strain and age of mice, and even purchasing company (especially outbred), should be matched over experimental groups in TBI experiment. Based on our results, 8-week-old male ICR mice from B company subjected to 12 Gy of TBI showed LD70/30 and suitable as a mouse model for further development of new drug using the ideal total-body irradiation model.
Ionizing radiation is a type of energy composed of electromagnetic waves, like X-rays or γ-rays, and particles, like alpha, beta, or neutron particles. Ionizing radiation has extensive effects on rapidly proliferating cell populations, such as intestinal stem cells or proliferative crypt epithelial cells [1]. Additionally, the toxicity of ionizing radiation can induce multiple organ dysfunction syndromes, such as cerebrovascular syndrome, gastrointestinal syndrome, and hematopoietic syndrome. Depending on the absorbed dose, damaged tissues or organs could lead to acute radiation syndrome and eventually death within 30 days [234].
Such radiation exposure has been observed after nuclear disasters, e.g., nuclear war or terrorism, or for medical diagnostic machines, including X-rays, nuclear medicine, and radiotherapy. In the case of large-scale nuclear disasters, such as the Fukushima and Chernobyl nuclear disasters, which generate a large number of casualties, including military personnel and civilians, plans for the medical management of radiation exposure damage are needed [5]. In addition, despite a lack of effective therapeutic agents for the side effects of cancer radiotherapy, approximately 30% of cancer patients receive radiation treatment. Side effects of radiation therapy cause not only early-phase symptoms, including skin erythema, dry or moist desquamation of the skin, mucositis, nausea, fatigue, and diarrhea, but also late-phase effects, including radiation-induced fibrosis, atrophy, vascular damage, neural damage, and a range of endocrine and growth-related effects [789]. Moreover, radiation causes genetic mutations, and these mutant cells lead to carcinogenesis [1011]. Since the percentage of radiation therapy is lower in developing countries than in developed countries, the use of radiotherapy is expected to increase and therapeutic agents are needed to address the growing concerns regarding the side effects.
Currently, in the United States, active research focuses on the development of therapeutic agents against the effects of radiation; Entolimod (CBLB502; Cleveland Biolabs, Inc., Buffalo, NY, USA) is in the most advanced stage of development (Phase II clinical) and is likely to be commercialized [12131415]. In Korea, however, few studies have attempted to develop radiation exposure treatments, and no substances have entered the product development stage.
Animal models can be used to develop therapeutic agents for irradiation and to evaluate the efficacy of radiation treatment agents [1617]. To evaluate the effects of radiation, various animal models have been used, including rodents, canines, non-human primates, minipigs, and ferrets [18192021]. In particular, mice are the most frequently used animal model owing to their anatomical, physiological, and genetic similarities to humans, in addition to other advantages, such as small body sizes and short generation times [18]. In addition, they have various benefits with respect to the cost, space, time requirement, and availability of a large number of strains; accordingly, mouse models are widely used for medical research. Therefore, the purpose of this study was to develop a mouse model of total-body γ irradiation (TBI) by determining the lethal dose of 70% of mice within 30 days (LD70/30).
Male 7-week-old ICR and C57BL/6N mice were purchased from both A and B company in Korea. Male 4-week-old ICR and C57BL/6N mice were purchased from B company. All the mice were housed in a pathogen-free room under a constant 12-h light-dark cycle at 22±2℃ temperature and 50±10% humidity. The mice were fed standard laboratory chow (Koatech Inc, Seoul) and water ad libitum. After a week acclimatization, the mice were divided into 4 groups based on their body weight, which were control groups (n=5), 10, 11, and 12 Gy groups (n=15). The experimental protocol was approved by the Institutional Animal Care and Use Committee of Konkuk University (No. KU14089-2).
Total-body γ-irradiation (TBI) was performed on anesthetized mice with intraperitoneal injection of Zoletil/Rumpun®, using 60Co-radiation at Korea Institute of Radiological & Medical sciences. Each mouse was placed inside a well-ventilated acrylic container, receiving 10, 11, and 12 Gy radiation exposure under standard laboratory conditions. After irradiation, mice were housed in laboratory animal room at College of Veterinary Medicine of Konkuk University and monitored every alternate day for body weight and survival for 30 consecutive days. From the point at which more than 50% died in each group, the data of body weight change rate were excluded. All data were analyzed using the program GraphPad Prism (GraphPad Software, Inc., San Diego, USA). Survival analysis was determined by log-rank test and P<0.05 is considered to be statistically significant.
In the first experiment, 8-week-old male mice were used to compare the LD70/30 between outbred ICR mice obtained from companies A and B. The ICR mice were exposed to a 12-Gy dose of radiation, resulting in a characteristic radiation-induced gastrointestinal syndrome comprising weight loss and death. All irradiated groups showed dramatic reductions in body weight within 3 days (Figure 1B, 1D, 1F). Eight-week-old ICR mice from company B showed body weight recovery after 3 days of TBI in the 10-Gy group (Figure 1B). A similar body weight change in ICR mice was observed for exposure to 11 Gy (Figure 1D), and dramatic weight loss was seen in the 12-Gy group (Figure 1F). The body weight change in 8-week-old ICR mice from company A was greater than that of mice obtained from company B for all levels of radiation. Mice from company A died up to 15 days post-radiation for all radiation levels, but mice from company B died up to 28 days (Figure 1E). For 10 Gy, the mortality of radiated mice was significantly higher for those obtained from company A than company B (Figure 1A, P=0.0002). Similar results using ICR mice were found for both 11 Gy and 12 Gy (Figure 1C, P=0.0406; Figure 1E, P=0.0346). The LD70/30 level for 8-week-old ICR mice from company A was approximately 10.5 Gy, while the level for mice obtained from company B was approximately 12 Gy (Figure 1).
In the second experiment (Figure 2), 8-week-old male mice were used to compare LD70/30 between inbred C57BL/6N mice obtained from companies A and B. High-dose irradiated C57BL/6N mice showed decreased body weights within 3 days, consistent with the previous ICR experiment. The survival rate of C57BL/6N mice in the 10-Gy group for company A was 60% and was 87% for company B. The survival rate of C57BL/6N mice in the 10-Gy group was significantly different between companies A and B (Figure 2A, P=0.0314). The differences in survival rates between companies A and B for both the 11- and 12-Gy groups were not significant. In the 11-Gy groups, the survival rates of the mice obtained from companies A and B were 20 and 40%, while in the 12-Gy groups, all mice from company A died within 13 days and 93% of mice from company B died. The LD70/30 levels for 8-week-old C57BL/6N mice from both companies were approximately 11 Gy.
In the third experiment, the difference in survival rate between 5-week-old and 8-week-old mice in both ICR and C57BL/6N strains was evaluated using mice from company B (Figure 3). No 5-week-old ICR mice that received a high radiation dose showed more than 40% mortality. All 5-week-old and 8-week-old ICR mice survived in the 10-Gy radiation group (Figure 3A). For 11 Gy of radiation, mice showed higher sensitivity to radiation in the 5-week-old ICR group (60%) than the 8-week-old ICR group (93%, Figure 3C, P=0.0333). Compared to the survival rate of 8-week old mice in the 12-Gy group, a slight, but insignificant increase was observed in the 5-week-old ICR group (Figure 3E, P=0.2143). For the C57BL/6N strain, all 5-week-old mice survived, and a slight decrease in the survival rate was detected in 8-week-old mice using 10 Gy (Figure 3B). The sensitivity to radiation exposure for 5-week-old C57BL/6N mice was higher than that of 8-week-old mice for 11 Gy (Figure 3D), but the opposite pattern was observed for 12 Gy (Figure 3F). Compared to the 8-week-old ICR and C57BL/6N mice, the differences in survival rate for 5-week-old mice for different radiation doses were not significant.
Despite increasing concern regarding radiation exposure from anti-cancer radiation therapy, nuclear war, or nuclear accidents, which can lead to skin diseases, genetic mutations, or even death, few studies have examined the effects and therapeutic agents for radiation exposure [922]. The development of treatment agents for radiation exposure provides a market for new treatment agents, but also minimizes military damage by establishing a system for protection against nuclear attacks. Establishing an animal model to evaluate the efficacy of radiation exposure treatment agents and to predict side effects is essential. To establish a mouse model of TBI based on the LD70/30, body weight changes and survival rates of ICR and C57BL/6N mice were observed.
In the first experiment, to compare the LD70/30 of mice obtained from manufacturing companies A and B, ICR mice were exposed to high doses of radiation and changes in their body weight and survival were monitored for 30 days. Changes in body weight for ICR mice from company A were greater than the changes observed for mice from company B. In addition, the survival rate was significantly lower for mice from company A than company B, indicating differences in susceptibility to radiation among mouse manufacturing companies. The LD70/30 levels in 8-week-old ICR mice was about 10.5 Gy from company A and about 12 Gy for mice from company B.
Sensitivity to a high dose of radiation was compared between C57BL/6N mice obtained from the two companies. Compared to ICR mice, the overall mortality rates of C57BL/6N mice for every radiation level were higher, except for 10-Gy irradiation for mice from company A. Only 10-Gy irradiated C57BL/6N mice exhibited a significant difference in survival rate between the companies. In the outbred ICR groups, differences in survival rates for all levels of radiation between companies were significant [23]. The irradiation level that represents LD70/30 for TBI in C57BL/6N mice from companies A and B was approximately 11 Gy.
To examine age-related differences in radiation sensitivity, 5-week-old mice were used in the third experiment. In 5-week-old ICR mice exposed to all radiation levels, the survival rate was greater than 60%. In contrast to the similar survival rates among the 5-week-old ICR groups, 8-week-old ICR mice exhibited dose-dependent survival rates. Significant differences were observed between the 11-Gy 5-week-old and 8-week-old groups; however, for 10 and 12 Gy, there were no significant differences between mouse ages. Similar to the results obtained for the ICR groups, no significant differences were found among the C57BL/6N groups.
In conclusion, differences in the sensitivity to radiation exposure according to mouse age were not significant, but we found differences in sensitivity with respect to the mouse manufacturing company, especially for outbred ICR mouse. Therefore, 8-week-old ICR mice from company B for a radiation level of 12 Gy was chosen as a mouse model based on the LD70/30 for TBI, rather than C57BL/6N, which exhibited high mortality. The mouse model established in our research could be used to develop therapeutic agents for radiation exposure or to evaluate the efficacy of treatment agents.
Acknowledgments
This paper was supported by the Civil-Military Technology Cooperation Program in 2014.
References
1. Zhang C, Ni J, Li BL, Gao F, Liu H, Liu W, Huang YJ, Cai JM. CpG-Oligodeoxynucleotide Treatment Protects against Ionizing Radiation-Induced Intestine Injury. PLoS One. 2013; 8(6):e66586. PMID: 23805241.


2. Herodin F, Drouet M. Cytokine-based treatment of accidentally irradiated victims and new approaches. Exp Hematol. 2005; 33(10):1071–1080. PMID: 16219528.
3. Koenig KL, Goans RE, Hatchett RJ, Mettler FA Jr, Schumacher TA, Noji EK, Jarrett DG. Medical treatment of radiological casualties: current concepts. Ann Emerg Med. 2005; 45(6):643–652. PMID: 15940101.


4. Plett PA, Sampson CH, Chua HL, Joshi M, Booth C, Gough A, Johnson CS, Katz BP, Farese AM, Parker J, MacVittie TJ, Orschell CM. Establishing a murine model of the hematopoietic syndrome of the acute radiation syndrome. Health Phys. 2012; 103(4):343–355. PMID: 22929467.


5. Dorr HD, Meineke V. Appropriate radiation accident medical management: necessity of extensive preparatory planning. Radiat Environ Biophys. 2006; 45(4):237–244. PMID: 17047978.


6. Bentzen SM. Preventing or reducing late side effects of radiation therapy: radiobiology meets molecular pathology. Nat Rev Cancer. 2006; 6(9):702–713. PMID: 16929324.


7. Hwang JH, Chang HJ, Shim YH, Park WH, Park W, Huh SJ, Yang JH. Effects of supervised exercise therapy in patients receiving radiotherapy for breast cancer. Yonsei Med J. 2008; 49(3):443–450. PMID: 18581595.


8. Senkus-Konefka E, Jassem J. Complications of breast-cancer radiotherapy. Clin Oncol (R Coll Radiol). 2006; 18(3):229–235. PMID: 16605054.


9. Jereczek-Fossa BA, Marsiglia HR, Orecchia R. Radiotherapy-related fatigue. Crit Rev Oncol Hematol. 2002; 41(3):317–325. PMID: 11880207.


10. Williams D. Radiation carcinogenesis: lessons from Chernobyl. Oncogene. 2008; 27:S9–S18. PMID: 19956182.


11. Morgan WF, Day JP, Kaplan MI, McGhee EM, Limoli CL. Genomic instability induced by ionizing radiation. Radiat Res. 1996; 146(3):247–258. PMID: 8752302.


12. Burdelya LG, Krivokrysenko VI, Tallant TC, Strom E, Gleiberman AS, Gupta D, Kurnasov OV, Fort FL, Osterman AL, Didonato JA, Feinstein E, Gudkov AV. An agonist of toll-like receptor 5 has radioprotective activity in mouse and primate models. Science. 2008; 320(5873):226–230. PMID: 18403709.


13. Krivokrysenko VI, Shakhov AN, Singh VK, Bone F, Kononov Y, Shyshynova I, Cheney A, Maitra RK, Purmal A, Whitnall MH, Gudkov AV, Feinstein E. Identification of granulocyte colony-stimulating factor and interleukin-6 as candidate biomarkers of CBLB502 efficacy as a medical radiation countermeasure. J Pharmacol Exp Ther. 2012; 343(2):497–508. PMID: 22837010.


14. Wang ZD, Qiao YL, Tian XF, Zhang XQ, Zhou SX, Liu HX, Chen Y. Toll-like receptor 5 agonism protects mice from radiation pneumonitis and pulmonary fibrosis. Asian Pac J Cancer Prev. 2012; 13(9):4763–4767. PMID: 23167416.


15. Burdelya LG, Gleiberman AS, Toshkov I, Aygun-Sunar S, Bapardekar M, Manderscheid-Kern P, Bellnier D, Krivokrysenko VI, Feinstein E, Gudkov AV. Toll-like receptor 5 agonist protects mice from dermatitis and oral mucositis caused by local radiation: implications for head-and-neck cancer radiotherapy. Int J Radiat Oncol Biol Phys. 2012; 83(1):228–234. PMID: 22000579.


16. Rice AS, Cimino-Brown D, Eisenach JC, Kontinen VK, Lacroix-Fralish ML, Machin I. Preclinical Pain Consortium. Mogil JS, Stohr T. Animal models and the prediction of efficacy in clinical trials of analgesic drugs: a critical appraisal and call for uniform reporting standards. Pain. 2008; 139(2):243–247. PMID: 18814968.


17. Talmadge JE, Singh RK, Fidler IJ, Raz A. Murine models to evaluate novel and conventional therapeutic strategies for cancer. Am J Pathol. 2007; 170(3):793–804. PMID: 17322365.


18. Singh VK, Newman VL, Berg AN, MacVittie TJ. Animal models for acute radiation syndrome drug discovery. Expert Opin Drug Discov. 2015; 10(5):497–517. PMID: 25819367.


19. Krigsfeld GS, Savage AR, Billings PC, Lin L, Kennedy AR. Evidence for radiation-induced disseminated intravascular coagulation as a major cause of radiation-induced death in ferrets. Int J Radiat Oncol Biol Phys. 2014; 88(4):940–946. PMID: 24495588.


20. Shim S, Jang WS, Lee SJ, Jin S, Kim J, Lee SS, Bang HY, Jeon BS, Park S. Development of a new minipig model to study radiation-induced gastrointestinal syndrome and its application in clinical research. Radiat Res. 2014; 181(4):387–395. PMID: 24786169.


21. Sanzari JK, Wan XS, Krigsfeld GS, King GL, Miller A, Mick R, Gridley DS, Wroe AJ, Rightnar S, Dolney D, Kennedy AR. Effects of solar particle event proton radiation on parameters related to ferret emesis. Radiat Res. 2013; 180(2):166–176. PMID: 23883319.


22. Meineke V, van Beuningen D, Sohns T, Fliedner TM. Medical management principles for radiation accidents. Mil Med. 2003; 168(3):219–222. PMID: 12685687.


23. Iwakawa M, Noda S, Ohta T, Ohira C, Lee R, Goto M, Wakabayashi M, Matsui Y, Harada Y, Imai T. Different radiation susceptibility among five strains of mice detected by a skin reaction. J Radiat Res. 2003; 44(1):7–13. PMID: 12841593.


Figure 1
8-week-old ICR mice from A and B companies were irradiated using different dose radiation (10, 11, and 12 Gy). Survival rate and body weight changes of radiated mice were monitored for 30 days. P values were calculated using a log-rank test (Mantel-Cox test).
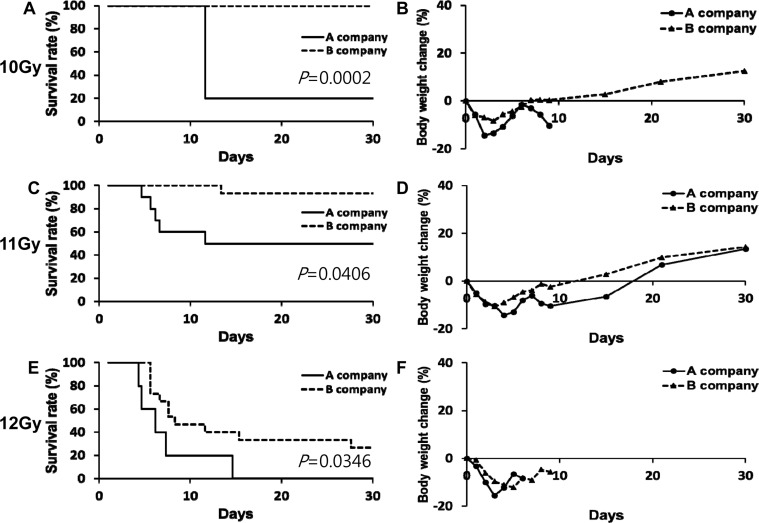
Figure 2
8-week-old C57BL/6N mice from A and B companies were irradiated using different dose radiation (10, 11, and 12 Gy). Survival rate (A, C, and E) and body weight changes (B, D, and F) of radiated mice were monitored for 30 days. P values were calculated using a log-rank test (Mantel-Cox test).
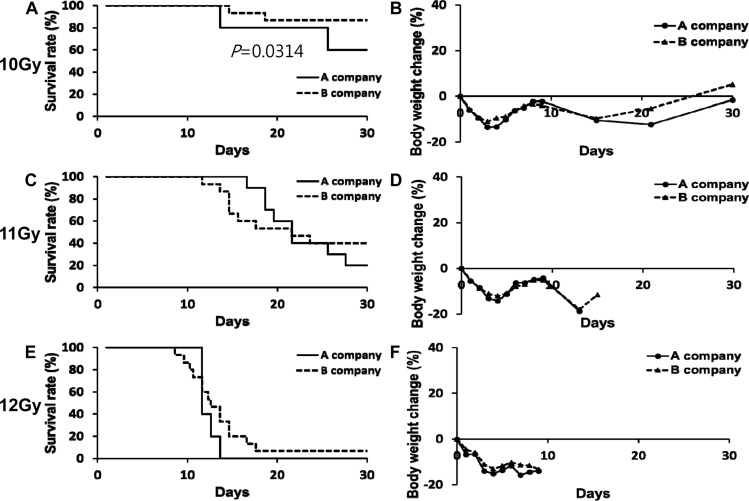