Abstract
Inflammatory Bowel Disease (IBD) is a multifactorial disorder with many different putative influences mediating disease onset, severity, progression and diminution. Spontaneous natural IBD is classically expressed as Crohn's Disease (CD) and Ulcerative Colitis (UC) commonly found in primates; lymphoplasmocytic enteritis, eosinophilic gastritis and colitis, and ulcerative colitis with neuronal hyperplasia in dogs; and colitis in horses. Spontaneous inflammatory bowel disease has been noted in a number of rodent models which differ in genetic strain background, induced mutation, microbiota influences and immunopathogenic pathways. Histological lesions in Crohn's Disease feature noncaseating granulomatous inflammation while UC lesions typically exhibit ulceration, lamina propria inflammatory infiltrates and lack of granuloma development. Intestinal inflammation caused by CD and UC is also associated with increased incidence of intestinal neoplasia. Transgenic murine models have determined underlying etiological influences and appropriate therapeutic targets in IBD. This literature review will discuss current opinion and findings in spontaneous IBD, highlight selected transgenic rodent models of IBD and discuss their respective pathogenic mechanisms. It is very important to provide accommodation of induced putative deficits in activities of daily living and to assess discomfort and pain levels in the face of significant morbidity and/or mortality in these models. Epigenetic, environmental (microbiome, metabolome) and nutritional factors are important in IBD pathogenesis, and evaluating ways in which they influence disease expression represent potential investigative approaches with the greatest potential for new discoveries.
Inflammatory Bowel Disease (IBD) is a multifactorial disorder for which a complete etiology is not yet known, but which occurs in response to the convergence of genetic predisposition towards disease development, intestinal microbiota, external environmental factors, and host immunobiological responses [1234]. Human IBD is a naturally occurring polygenic disease expressed as abdominal pain, weight loss, diarrhea, vomiting, flatulus and ileus, ileitis, bloody stool, elevated mucous production and bowel and/or subcutaneous fistulation present in various proportions depending the area of the small and/or large bowel that is being affected [25]. IBD predominantly occurs sporadically, with familial IBD occurring in only 5-14% of the affected population [236]. IBD occurrence represents a major risk factor in development of colorectal cancer [78]. Clinical inflammatory bowel disease syndromes include Ulcerative Colitis (UC) in which limited mucosal and submucosal inflammatory processes predominantly affect the colon and adjacent areas of the rectum, and Crohn's Disease (CD) characterized by chronic intermittent relapsing discontinuous transmural inflammation of the bowel with intestinal and perianal fistulation [9]. Spontaneous natural IBD is also found in dogs and cats, horses and primates [1011]. Canine phenotypic IBD disease classifications include lymphoplasmacytic enteritis, eosinophilic gastroenteritis, eosinophilic colitis, granulomatous colitis and histiocytic ulcerative colitis and neuronal hyperplasia [1011121314]. In horses, IBD is usually expressed as weight loss and clinical colitis which exhibit variable long-term responses to corticosteroid and anthelmintic treatment [15]. Nonhuman primates frequently exhibit diarrhea, and a subset of these animals appear to exhibit chronic diarrheal disease that can be categorized within familial aggregate groups. This syndrome is a leading cause of mortality in domestic macaque colonies [16]. Finally, cattle and other ruminants once infected with Mycobacterium avium paratuberculosis will exhibit weight loss, chronic diarrhea, and other clinicopathological syndromes that are very similar to human inflammatory bowel disease, which is particularly significant because this organism is difficult to detect, can survive pasteurization and subsequently enter the human food chain [17].
While many histological disease phenotypes and lesions expressed by human and animal patients are similar, some do differ, with the result that there is no one animal model that replicates every major expression of human or animal IBD among experimental animal models. Additionally, in some instances functional phenotypes and histologic indications of inflammatory bowel disease can resemble other disease entities, which confound study and clinical diagnosis, and must be identified, treated and/or eliminated from consideration in determining disease etiology and pathogenesis [2351011121318].
Histological lesions in Crohn's Disease feature noncaseating granulomatous inflammation [23518] while UC lesions typically exhibit ulceration, lamina propria inflammatory infiltrates and lack of granuloma development [23]. IBD is a diagnosis of exclusion. Patients have traditionally been diagnosed and categorized based on endoscopic histopathological lesion appearance and clinical responsiveness to medication [235131819]. There are several different systems that have been used to characterize histological lesions observed in IBD, but classical histological interpretation of mixed inflammatory cellular infiltrates can be very subjective due to their occurrence in response to non-IBD related disease etiologies, and also because a uniform set of prognostic biomarkers has not yet been fully developed in human or veterinary medicine for CD or UC diagnosis [311]. Most small animal IBD patients are initially treated with diet restrictions, use of metronidazole to change the intestinal microbiota by eliminating anaerobic bacterial species; increased fiber content and anti-inflammatory products [1220]. Human UC and CD patients are treated using first line therapies, including oral mesalamine, sulfasalazine for colitis only, Budesonide oral corticosteroid and oral proton pump inhibitors such as omeprazole. Increasingly, newly introduced tumor necrosis factor antibody and other inhibitors are improving the success of human IBD therapy [21], and have been introduced in animal models to examine underlying pathogenic mechanisms [2223]. If initial treatment measures are not effective second line immunosuppressive therapy such as high dose prednisone, azathioprine, methotrexate and immunogold preparations; and biological adjuvants such as the chimeric murine-human anti-tumor necrosis (TNF) antibodies Infliximab, Adalimumab and Certolizumab; and the Integrin antibody Natalizumab, will be added to the treatment protocol [82024].
However, many of these drug and biological adjuvant regiments do have significant side effects, and it would be advantageous to identify predictive biomarkers and new, effective therapeutic protocols to assist in managing these diseases [101121]. Identifying biomarkers associated with different pathogenic and/or progressive stages in inflammatory bowel disease in humans has been difficult in part because of great variability in the contribution of underlying mechanisms to disease incidence, and in disease onset, progression, and remission over time [21]. It is also not unusual for severely affected IBD patients to experience multiple abdominal surgeries to remove adhesions, obstructions, strictures and chronic inflammatory material debridement [20]. Experimental genetically modified transgenic animals are important model system applications in determining disease causation, identifying potential therapeutic targets, and piloting measures contributing to alleviation of pain and distress in model systems. This article will review the transgenic rodent models of IBD and shed light on their respective pathogenic mechanisms, contributions to knowledge about IBD etiological influences, and applicability for therapeutic drug intervention development and testing. Animal studies reviewed in this report were presumed to have been conducted in accordance with humane regulatory standards by respective original authors in accordance with best animal welfare practices and international publication standards.
IBD is thought to be a result of dysregulated cell signaling due to changes in bowel microbiota, diet as well as overstimulation and deficient regulation of innate and adaptive immune mechanisms within the bowel environment. The resultant spectrum of disease phenotypes have been termed "dysbiosis", representing a loss of intestinal immune homeostasis [235182526], with particular emphasis on the Th17 cell phenotypeassociated activities and dysregulation of Treg cells in the intestinal mucosa, including IL10 expression [27]. Some of the IBD animal models have relied heavily on adoptive transfer techniques to more clearly define disease activity spectra following reconstitution of selected, genetically manipulated cell populations in immunodeficient, genetically modified mouse models of inflammatory bowel disease [2728]. Only 7 to 14 % of human spontaneous disease incidence has been attributed to genetic causes. Recently, Parlato and Yeretssian noted that classified gene loci converge into common biological pathways essential for intestinal homeostasis- or inflammation-related processes, which included networks such as epithelial wound repair and barrier function, innate immune responses, autophagy, and T-cell differentiation among others [29]. Some environmental influences, in particular, commensal bacterial intestinal population characteristics, have become considered to be increasingly more important contributors to disease pathogenesis [46]. Such variability is also challenging to replicate in animal models [21]. IBD is characterized by the presence of dysregulated immunoresponses governed by the activities of CD4+ Th1, Th2, Th17, and Treg lymphocytes, resulting in sustained inflammation, mixed immune cell population recruitment, infiltration and functional activation, and marked shifts in enteric nervous system function [1130]. Traditionally, T cells have been differentiated by their abilities to produce interferon gamma (Th1) and interleukins like IL4, IL5 and IL15 (Th2) [23182531]. Th1 cells are most effective in killing intracellular pathogens such as bacteria and viruses through monocytic pathways while Th2 cells are especially effective in eliminating parasites resident in mucosal sites through activation of eosinophils, basophils and mast cells [2]. Several new T cell populations have also been identified and are thought to be important in driving disease development and progression in IBD [332]. These include subtype Th17, which appears to fill a niche activating neutrophil populations by eliminating extracellular bacterial and fungal infections as well as inflammation characterized by neutrophil activation and opsonizing IgG antibodies; the cells produce IL17, IL22, a part of the IL10 cytokine family, granulocyte colony stimulating factor (G-CSF) and granulocyte macrophage colony-stimulating factor (GM-CSF). Taken together these cells are especially important in supporting epithelial barrier integrity and microbial defense strategies [231825]. In addition, suppressor CD4+Treg subsets are active in opposition to Th17-induced inflammatory cell differentiation [2733].
When activated these cells are able to augment, coordinate and integrate adaptive immunity through their actions on diverse cell sets for pathogen eradication [3]. It is through effector cells (Th1: monocyte/macrophage lineages; Th2: eosinophils, basophils and mast cells; and Th17: neutrophils) and elaborated soluble factors that are either pro-inflammatory or anti-inflammatory in character [2351821]. Additional polymorphic loci variants for genes that have been associated with elevated risk disease as well as other not yet identified candidate genes may also influence disease expression onset, development and progression. These unknown polymorphic variants may be modified through the accumulative effect of multigenerational single nucleotide polymorphisms [2], or, perhaps through modification of a significant as yet not described polymorphic gene variant by additional factors [2] resulting in gradation of disease expression.
Recent genome wide association studies involving large case cohort human subjects trials have identified over 100 loci that are associated with enhanced risk of developing CD, UC, or both [2334], including those governing innate genes (definitive associations of nucleotide-binding oligomerization domain proteins (NOD proteins) [NOD2 in the IBD1 16q12 linkage region for CD only] [1335]; modified immune function related to guanosine-5'-triphosphate hydrolyase (GTPase) M protein and autophagy-related protein 16-L1 (ATG16L1) autophagy gene expression, janus kinase 2 (JAK2) activation; and IL12B/p40) gene expression [25]. The strongest CD gene associations are with IL23R and NOD2 [2], and an intact IL23R is required in mouse IBD models for intestinal inflammation phenotypic expression [2].
On the other hand, NOD proteins are important cytosolic regulators of apoptopsis and pathogen response and resistance that contain leucine rich repeat segments. They express genetic polymorphisms in regions that have been associated with inflammatory disease or increased susceptibility to infection [303536]. The NOD proteins NOD 1 and NOD 2 are important in sensing the presence of bacterial pathogens by identifying peptidoglycan wall peptide ligands gamma-D-glutamylmeso-diaminopimelic acid and muramyl dipeptide components that are associated with bacterial walls, and work with other proteins, specifically the Toll-like receptors (TLRs), in mediating responses to bacterial invasion [36]. Once NOD proteins detect peptidoglycan group patterns in bacterial cells they are activated through the leucine rich repeat segment, recruit and bind receptor-interacting serine/threonine kinase (RICK) through the caspase-recruitment domain interactions (CARD) [3037]. RICK activation then stimulates polyubiquitulanation of IkkB [30]. The resultant phosphorylation event activates nuclear factor kappa B (NF-κB), mitogen-activated protein kinases (MAPKs) such as JUN-amino terminal kinase (JNLK), JAK, extracellular signal-regulated kinases (Erk) and p38 MAPK while also stimulates pro-inflammatory cytokine expression [303537]. All of the transcription factors and cytokines involved in this pathway are important mediators of inflammatory disease and immune pathogenesis, and through the CARD system, apoptosis and cysteine-dependent-aspartate directed protease (caspase) protein activation [303537]. NOD1 is less active in this functional pathway, and has been primarily associated with demonstrating elevated levels in response to bacterial infection such as Helicobacter pylori gastroenteritis [30].
Major NOD 2 mutations R702W, G908R and L1007fsinsC are associated with impaired responses to the presence of bacterial pathogens (and in some cases putative commensal bacteria) that are persistently found in the lamina propria [36]. The protein is expressed in lamina propria macrophages, monocytes and paneth cells of the mucosal epithelium, thus regulating production of antimicrobial alpha-defensins [36] in the mucosa. The resultant overstimulatory reaction causes CD; alternatively, some have suggested that NOD deficiency/loss of function models are associated with T cell activation with subsequent cytokine and chemokine release [36]. These reactions may also be associated with other immune-mediated or influenced diseases such as pediatric sepsis, allergy and atopy, psoriasis, sacroilitis, and graft vs host disease [3036]. In two syndromes, Blau syndrome and Early-onset sarcoidosis, these mutations occur in the NOD region and not in the LR domain. They are associated with granulomatous pathologic disease, and appear to operate through gain of function mutants, demonstrated through increased NF-κB activation in the absence of muramyl dipeptide stimulation and in response to excessive NOD signaling [30]. Once activated NOD 2 interacts with CARD12, which negatively regulates RICK-mediated proteins and stimulating NF-κB translocation into the nucleus, and release of proinflammatory cytokines. Conversely, individuals with CARD15 mutations show very strong immune reactions to peptidoglycan peptides, elaborate high levels of IL12 and IL23, and can easily support development of Th1 mediated clinical colitis [30]. Interestingly, the CARD proteins are instrumental in activating caspase proteins that are important mediators of apoptosis, inflammation, necrosis and cell death [37], and can recognize intracellular double stranded RNA which is commonly found in viral genomes ranging from the Orthomyxoviridae viruses such as Influenza virus, to Rhabdoviridae family viruses such as Rabies virus [37]. Both NOD and caspase proteins are considered to be part of the inflammasome constituent of nucleotide-binding domain, leucine rich containing (NLR) family proteins [37]. It is because of these complex innate immunological intersecting pathways that gene ablations and/or mutations induced in knockout and transgenic mouse models are so applicable to the study of disease syndromes [3638].
Another important limb of the innate immune system are the Pathogen Associated Molecular Patterns (PAMP) molecules, of which the TLR protein molecules are excellent examples of their activities. TLRs are transmembrane cell surface receptors that are associated with cell membranes [30] and are important mechanisms by which pathogen-associated molecular patterns are recognized in the activation of innate immune responses, especially to bacteria, but also to viruses and fungi [3940414243]. They were initially recognized as genetic homologues to the Drosophila Toll gene family. In humans, there are ten different TLRs that have been characterized, all of which recognize different pathogen-associated molecular patterns [40]. The TLRs recognize different aspects of pathogen structure. TLR9 recognizes unmethylated CpG dinucleotides in bacterial DNA; TLR2 recognizes diacyl lipopeptides present in gram negative cell walls; and TLR4 binds to lipopolysaccharide extracellular cell domains and stimulates signaling cascades as demonstrated by recent crystal structures demonstrating the protein in complex with a functional antagonist [40]. The TLR family functions appear to be conserved in vertebrates, and were most likely established as a part of the early innate immune defense system allowing detection of lipoprotein, peptidoglycan, lipopolysaccharides, flagellin, double or single strand RNA and cytosine poly-guanine (CpG) DNA as pathogen associated molecular patterns early in evolution [40].
Adaptive immune system functional associations for CD and UC appear to function through IL23 receptor signaling and Th17 cell subsets [25]. Substances involved in these phenomena include interleukin 12A (IL12A, p40) and Signal transducer and activator of transcription 3 (STAT3) proteins [25] among others. STAT3 protein phosphorylation activation roles in IBD are likely to be both complex and confounding given that this protein family is downstream of pro- and anti-inflammatory cytokines and is activated directly by IL23 signaling through the IL23R complex [25]. There is continuous cross talk and regulation between Th1, Th2, Th17 and FoxP3+ CD4+ T regulatory subset populations in association with changes in IBD disease phenotype expression [3]. Within the Th17 functional pathway, Th17 cells defend against Gram positive and Gram negative bacteria and upon activation secrete IL17A, IL17E, IL17F, IL21, IL22, IL26, TNF alpha, interferon gamma, and various chemokines [325]. IL21 expressing effector cells in this pathway, typically mucosal epithelia and skin, function as a part of enhancing barrier immunity [3].
Other Th17 associated genes expressed in CD phenotypes include C-C motive chemokine receptor 6 (CCR6) in dendritic cells and memory T cells where they mediate B cell differentiation, dendritic and memory T cell migration; and tumor necrosis factor ligand superfamily member 15 (TNFSF15), which facilitates Th17, Th1 and Th2 cell differentiation [25]. Similarly, the combined presence of IL1, IL6, IL21 and TGF beta will induce IL23R upregulation, resulting in Th17 cell differentiation in some settings [3], and highlighting linkage between autoimmunity and IBD [2]. TGFβ functions in this manner through reciprocal induction of forkhead box P3 (FoxP3) and retinoid-related orphan receptor gamma (RORγ) [2]. FoxP3 interactions with RORγt suppress IL17 transcription, while the presence of IL6, IL21 and IL23 will decrease FoxP3 inhibition by RORγt [2]. Through these mechanisms which are dysregulated by IBD, Th17 and T regulatory cells are reciprocally regulated, and homeostatic expression levels determine self-tolerance and absence of bowel inflammation [2325].
The Th17 functional pathway is important in modulating mucosal responses to pathogen exposure. It exerts considerable effect in inflammatory bowel disease as well as inflammatory diseases affecting the lung and skin [3]. Two CD associated genes, chemokine receptor 6 (CCR6) and tumor necrosis factor ligand superfamily member 15 (TNFSF15) (http://www.ncbi.nlm.nih.gov/gene/9966), enhance IL17 expression in Th17, Th1 and Th2 cells. These variants appear to be important factors in CD development among people of European and Asian ancestry [3]. Both CCR6 and TNFSF15 have been linked to Th17 cell differentiation and function [25]. It is worth noting that there are differences in Th17 cell development between mice and humans resulting in variable IL1β and other pro-inflammatory cytokine spectra and expression levels between species [3].
TGFβ and IL1β appear to contribute to Th17 cell development and differentiation at different levels between species [3]. Th17 cells can differentiate into interferon-gamma producing effector cell populations that resemble Th1 cells under certain conditions, but their contribution to UC and CD has not been fully demonstrated [3]. Overall, however, cross regulations of Th17, Th1 and Treg cells are important modifiers of disease expression, with TGFβ causing activation of both T17 and Treg cell subsets [25]. TGFβ alone stimulates Treg cell differentiation, while the presence of IL1, IL6 and IL21 causes induction of IL23R expression, and differentiation of Treg cell subsets [25]. Based on recent studies, it appears that there are gene perturbations, in common, between CD and UC, namely, those activated along the IL23 pathway (IL23R, IL12B, and STAT3) [25]. Ongoing studies continue to define single nucleotide polymorphisms in these regions as well as elsewhere throughout the human genome [233] and in animal models of this disease [182225].
UC syndromes are associated with IL10 and actinrelated protein 2/3 complex subunit 2 (ARPC2) regulatory pathways, intestinal epithelial cell function extracellular matrix 1 (ECM1) and activation of the E3 ubiquitin ligase containing hect domain and RLD 2 (HERC2 ) [2]. HERC2 expression is important in DNA repair function and share a common immune dysregulation genetic association with human leucocyte antigen regions of the major histo-compatibility complex [2]. Inflammatory bowel associated immune related genes have also been shown to be associated with other diseases in humans [2] including diabetes mellitus types 1 and 2, psoriasis, systemic lupus erythematosus, Graves' Disease and rheumatoid arthritis among others. This association suggests that a more general tendency to develop immune related disease may exist in some individuals that when exacerbated by additional environmental or epigenetic factors activates a pathway resulting in an individual and specific disease syndrome(s) among different human populations [2]. Because the clinical expression and therapeutic response characteristics of inflammatory bowel disease is similar across patient populations investigators have suggested that there is a limited disease phenotypic range for these diseases in humans [2].
There is an increased risk for colonic adenocarcinoma in infectivity models such as Helicobacter hepaticas, Citrobacter rodentium, Lawsonia intracellular, use of chemical mutagens, and the presence of predisposing genetic mutations including adenomatous polyposis coli-multiple intestinal neoplasia (APCmin and Cdx2 genes) [2212233]. Equally important appears to be the developing interest in associating microbiota population characteristics with disease induction, expression and progression, as enterocolitis and colonic cancer expression can vary in rodent models in part in accordance with the presence or absence of defined bowel microbial species and load [2451844]. However, the complex and sometimes confounding pathologic responses observed clinically in human patients and in relevant animals models reflect putative variation in pathogenic disease mechanisms, suggesting that these two disease phenotypes may represent a disease continuum, rather than distinct entities, and highlight our need for further delineation of cell reactivity lineages, functional pathways and resultant phenotype expression in these diseases [2]. Transgenic rodent models can serve as excellent model systems for mechanistic studies to further define underlying pathogenic disease factors, and in initial proof of concept studies to determine therapeutic approaches for future clinical efficacy.
Spontaneous inflammatory bowel disease has been noted in a number of rodent models which differ in genetic strain background, induced mutation, microbiota influences and immunopathogenic pathways [2]. There are now many different inflammatory bowel disease animal models, with recent publications identifying in excess of 1800 recent individual publications in this area within the past three years [921224546]. One comprehensive review by Neurath in 2012 has identified over 69 spontaneous and induced rodent models in which clinicopathologic and therapeutic models of either CD or UC, or both, was invoked [9]. Animal models of inflammatory bowel disease continue to be very useful in examining basic pathogenic mechanisms of disease as well as in developing and evaluating new types of biological therapies such as anti-tumor necrosis factor, anti-integrin and anti-interleukin therapies [19283346474849]. Many IBD animal models exhibit Th1 cell dominant immune reactivity (Mizoguchi A and Mizoguchi E 2010), with associated pro-inflammatory cytokine expression profiles, e.g. interferon γ, tumor necrosis factor-α and IL2 [50]. Non-T cellular monocytic cell populations are important regulators of bowel mucosal immunity so that when modified they can result in disease expression. Antigen presenting cells seem to be important determinants of Th2 cell immune response induction when challenged with infectious agents, thus providing a linkage between adaptive and innate immune responses in IBD disease onset [33]. There are significant and complex interactions between adaptive immunity, innate immunity and intestinal microbiota (including aspects of microbial species population as well as metabolic compound assessment) and external environmental influences, suggesting that there are many opportunities for future basic and translational animal model development in this area.
One of the earliest transgenic mouse models involved in driving control of TGFβ via use of a dominant negative mutant of the TGFβ type II receptor, the ITF-dnRII transgenic mouse (Table 1), first described in 2001 to explore the effects of TGFβ1 on bowel immunity, extracellular matrix protein expression, and carcinogenesis [515253]. This model examined the hypothesis that modified TGFβ signaling was responsible for intestinal epithelial cell degradation and subsequent IBD disease development in mice similar to human inflammatory bowel disease expression [515253]. The role of the TGFβ signaling pathway was initially examined in ITF-dnRII mice through overexpression of mutant dominant negative TGF-β receptor II, resulting in functional inactivation of TGFβRII receptor proteins under the control of intestinal trefoil peptide promoter (ITF/TFF3) in a tissue restricted fashion. Resulting ITF/dnRII transgenic mice exhibited clinical IBD disease when not maintained in specific pathogen free conditions, including weight loss, ruffled coat, diarrhea, hematochezia and anal/rectal prolapse [51]. Loss of TGFβRII expression was noted throughout the epithelial and muscular layers in transgenic mice. When maintained in specific pathogen free conditions these mice exhibited similar signs only after treatment with dextran sodium sulfate in drinking water which has been shown previously to produce acute and chronic ulcerative colitis in mice [51]. Characteristic histopathogical changes were noted including severe, extensive mucosal ulceration, severe inflammatory cell infiltration and increased myeloperoxidase reactivity indicative of the presence of granulocytic cells. Additional clinicopathologic characteristics included generation of major histocompatibility complex class II antibodies in sera, increased matrix metalloproteinase activity within bower epithelium and autoantibody generated against intestinal goblet cells [51]. In subsequent additional studies of dominant negative TGFβII reception constructs expressing downregulation of TGFβIIR in the intestine, their absence was associated with higher cell nuclear antigen proliferation indexes following infection with Helicobacter pylori. When treated with azoxymethane, a colon carcinogenic agent, these mice also exhibited increased numbers of aberrant crypt foci and higher incidences of dysplastic precancerous aberrant crypt foci and colonic cancer [52]. Loss of TGFβ intestinal signaling appears to increase organotypic susceptibility to UC, appears to influence level of tissue injury during inflammatory disease and may be associated with associated colonic neoplastic changes [51]. Later studies have examined inflammatory colitis induced through dextran sodium sulfate and azoxymethane treatment of Smad3-/- mice, which are deficient in TGFβ signaling molecule smad3 [53]. The outcome of these studies suggest that it most closely models CD and is significantly associated with subsequent carcinogenesis, including colonic epithelia crypt herniation, chronic-active repair characterized by epithelial hyperplasia, crypt herniation, squamous metaplasia, epithelial dysplasia, and subsequent tumor development [1953]. Neoplastic lesions ranged from adenomatous polyps to mucinous adenocarcinoma [53].
The double transgenic mouse dominant-negative knockout (dnKO) construct develop fulminant UC that is sensitive to anti-cytokine treatment and broad spectrum antibiotics [54]. dnKO transgenic mice were constructed on C57B6 background with dominant negative TGFβRII mice that were crossed with class II cytokine receptor family member 2 [CRF2-4-deficient (IL10R2-/-)] mice [55] that also resided on C57B6 background, resulting in mice that were null mutants for IL-10R2 and expressed dominant negative phenotypic expression of TGFβRII in the CD4+ and CD8+ compartments. Further breeding of dnTGFβRII mice with IL10R2-/- mice resulted in the development of the dnKO transgenic mouse, with restricted lymphocytic phenotypic expression as a result, with three other control conditions generated similarly: IL10R2-/-, dnTGFβRII and IL10R2+/- [54]. dnKO mice exhibit failure to thrive at an early age with rapid weight loss and early mortality, a much earlier and more severe expression of disease than is commonly found in single transgenic mutants, and in parallel with human patient clinical presentation in a subgroup of IBD affected individuals [54]. The pathological presentation of this model is most consistent with UC forms of IBD. Gross pathological changes included focal punctate ulceration, mucosal thickening in the cecum, descending colon and rectum, and milder changes in the ascending colon with minimal inflammatory change or damage noted in deeper intestinal layers and the absence of granulomata, colonic fistulas and strictures [54]. Severe inflammation was noted histologically and featured predominantly T cell infiltrates with additional immature myeloid/monocytic, neutrophilic, natural killer and B cell infiltrates as assessed using immunohistochemistry [54]. Histopathological changes were consistent with mucosal responses to inflammatory injury and included epithelial hyperplasia characterized by elevated crypt wall height and M-phase bodies per crypt ratios, statistically significant decreases in crypt numbers accompanied by increased crypt width and microabcessation, reduced numbers of goblet cells and eroded surface epithelium [54]. Cellular infiltrates consisted of mixed cell infiltrates into the mucosa and submucosa of the cecum, descending colon and rectum; leukocytic infiltrates were also detected in the ascending colon but epithelial and goblet cells escaped severe injury [54]. Proinflammatory Th1 cytokine expression (interferon γ, TNFα, and IL6) was marked with elevated sera concentrations, and was consistent with the present of large numbers of expanded T cell populations among the inflammatory infiltrate. Expression of colitis in these mice could be reversed through anti-cytokine (interferon γ and TNFα) antibody treatment and inhibited following high dose ciprofloxacin and metronidazole antibiotic treatment [54]. Treated animals gained weight, did not exhibit any clinical or histological signs of inflammation and injury, and produced minimal proinflammatory cytokine levels, demonstrating the efficacy of these two therapeutic interventions and the important role the luminal bacterial exert in the pathogenesis of UC in this model.
In their review DeVoss and Diehl identify two transgenic mice that exhibit clinical signs of ileitis were identified as being commonly used IBD animal models [21]. One of these is the tumor necrosis factor (TNF) deficient TNFΔARE transgenic mouse, which was generated from an original single TNF mutation on a C57/Bl6 background as a model of rheumatoid arthritis and other inflammatory arthridities [56]. The development of a double mutant mouse using Cre-Lox recombinant technology and carrying a deletion of the AU-rich elements 3' untranslated region (ARE 3'UTR) regulatory element for tumor necrosis factor resulted in an effective double disease model that can be used for investigative pathogenesis inquiry as well as for preclinical drug discovery [57]. ARE consist of a class of regulatory sequence characterized by the presence of an AUUUA pentanucleotide that acts to regulate mRNA stability and translation in TNF and other biologically active molecules [57]. Differential substitution of this mutant nucleotide into either a TNF type I or II receptor mutant genetic background mouse resulted in loss of posttranscriptional regulation of this gene. While double homozygous mice developed in a completely normal fashion (TNFΔARE/ΔARE/TNFRI-/-), TNFΔARE produced animals that exhibit inflammatory polyarthritis and bowel disease [57] (Biomedcode www.biomedcode.com/gr/en/content/tnfdaredual-disease-model). Heterozygous and homozygous TNFΔARE/- and TNFΔARE/ΔARE mice exhibit signs of arthritis (joint swelling, paw distortion and severe movement abnormalities) and inflammatory bowel disease consistent with chronic inflammation and CD. These include severe weight loss (to 6-7 grams) and early onset of disease by 12 days postnatally, and early mortality in homozygous mice by 5-12 weeks of age for TNFΔARE/ΔARE mice suggesting that they must be monitored closely to alleviate potential animal welfare and husbandry concerns.
Histopathologic intestinal lesions were first noted at 2 to 4 weeks of age in TNFΔARE and were localized to the distal ileum with occasional colonic involvement. Lesions progressed from mucosal injury characterized by villus blunting and broadening and chronic-active mucosal and submucosal infiltrates composed of scattered neutrophils, mononuclear lymphocytes and plasma cells [57]. Severe intestinal inflammation was noted by 4 weeks of age in TNFΔARE/ΔARE and by 8 weeks of age for TNFΔARE/+, progressing by 4-7 months of age to severe transmural inflammation characterized by submucosal lymphoid aggregates and follicles, granulomata that resembled non-caseating granulomatous change in human IBD, and multinucleated giant cells, with superficial epithelial layers exhibiting loss of villus structures. [57]. Subsequent studies have found that this phenotype occurs secondary to expression of Th-1 cytokine activation patterns including IL-12, interferon and requiring CD8+ T cell activation, with additional influences from redundant downstream cellular kinase activation pathways [58].
Mice deficient in both TNF and IL-10 (T/I mice) that were developed from knockout IL-10-/- on a C57B6 background (B6.129P2-Il10tm1Cgn/J) held under specific pathogen free conditions do not develop colitis. However, when this transgenic mouse is crossed with knockout TNF-deficient mice on a C57B6 background the result is a double knockout transgenic mouse (B6.129S6-Tnftm1Gkl/J) (Tnf+/-Il10-/- [T-het/I] and Tnf-/- Il10-/-/J) (http:/jaxmice.jax.org/strain/003008.html) that exhibits a UC phenotype and develops spontaneous colonic tumors without any exogenous triggers shortly after weaning [59]. This UC phenotype preferentially affected the terminal colon and rectum after 15 weeks of age with linear continuous inflammation involving the colon. Histopathological lesions consisted of large numbers of neutrophils within the lamina propria, crypt abscesses and mucosal ulceration that generally did not affect tissue deeper than the muscularis mucosae and submucosa [59]. Inflammatory bowel disease in this model was completed prevented in T/I mice using a drug cocktail consisting of amoxicillin, clarithromycin, metronidazole antibiotics and omeprazole acid suppression agent to modify the luminal environment and suppress or eradicate colitogenic bacteria [59]. Colon cancer phenotypes generated in this model ranged from invasive mucinous adenocarcinoma to locally advanced transmural invasive tumor breeching the exterior colonic mesenteric serosal surface in mice older than 25 weeks of age, and without accompanying metastases. This model suggests that relative TNF insufficiency may result in mucosal compromise in a primary or secondary fashion resulting in expression of UC IBD, with concomitant elevated risk of colonic cancer development in the face of intestinal inflammation in this double knockout transgenic mouse strain [59].
Similarly, SAMP1/Yit/Fc transgenic mice were generated on an AKR/J background and exhibit elevated numbers of macrophages, neutrophils and lymphocytes within the ileum indicative of immune activation in this site [606162]. SAMP1/Yit/Fc mice exhibit spontaneous small intestinal inflammation characterized by early secretary cell expansion, e.g. goblet, paneth and intermediate phenotype cells, within the epithelium of the ileum and discontinuous "skip" pattern of transmural and segmental chronic-active cellular infiltrates. These cells include lymphocytes, macrophages and neutrophils with initial crypt elongation. Inflammatory infiltrates target epithelial crypt epithelial locations and subsequently promote development of cryptitis, microabcessation, focal granulomatous inflammation and basal plasmacytosis with progressive disruption of the epithelium and tissue atrophy [62]. This granulomatous inflammation is observed under specific pathogen free conditions, commencing at 10 weeks of age with 100 % penetrance by 30 weeks of age and severe bowel strictures noted in mice older than 40 weeks of age [6162]. This is an unusual aspect of this model as clinicopathologic signs rarely develop under specific pathogen free conditions in many IBD rodent models, and especially those that are dependent in part on chemical induction of intestinal inflammation. Additional pathologies have been noted in SAMP1/Tit/Fc transgenic mice, including chronic Helicobacter-negative Crohn's like gastritis and autoimmune hepatitis [62]. The pathogenesis of this animal model is similar to that observed with human IBD disease in that early activation of Th1 mediated pathways are associated with disease induction and intraluminal commensal bacteria act as antigenic stimulants that result in immunological dysregulation, or dysbiosis, of mucosal immunity in these mice [62]. STAT3 is activated over the course of disease progression in SAMP1/Yit/Fc mice; accordingly, it is considered an important regulator of the inflammation in the SAMP1/Yit/Fc mouse as well as a potential therapeutic target [61].
Cytokine-associated murine colitis has been described in IBD animal models [435063]. These include one of the only Th2 cytokine predominant murine colitis IBD model described in the literature, Wiskott-Aldrich Syndrome protein (WASP) deficient mice [5064]. The WASP molecule is primarily expressed in hematopoietic cells where it integrates surface receptor and actin cytoskeleton signaling networks, and is absent, changed or diminished in WASP human patients. An X-linked disease, human patients affected with this deletion exhibit eczema, thrombocytopenia, lymphoreticular cancers, recurrent infections and autoimmune diseases [50]. Affected mice exhibit weight loss, diarrhea, rectal prolapse and early mortality by 3 months, and all surviving mice tend to be affected by 6 months of age. Clinicopathologic signs include lymphopenia, thrombocytopenia, cytoskeletal abnormalities characterized by cellular migration defects, T cell signaling defects and decreased regulator T cell quantity and diminished function [50].
This model is histologically characterized by the presence of neutrophils; CD8+, CD4+ and Treg cells; increased macrophage and dendritic cell populations within the colonic lamina propria by 3 months; crypt elongation followed by abcessation, goblet cell depletion, epithelial hyperplasia, and the absence of granulomata. In addition, a thickened bowel wall is noted accompanied by increased Th2 cytokine expression and dysfunctional natural regulatory T cell function that closely mimics human disease in all aspects. Interestingly, the WASP transgenic mice express normal TCR α+ κ+ peripheral T cells, and elevated IL13 expression thought to originate from natural killer T cells, which are also thought to be a colitogenic stimulus. It is not known if these cells are important actors in immune dysregulation associated with WASP deficiency in this model, however [50]. WASP transgenic mice have been shown to also lack the ability to fully polymerize cellular actin and express colitis following Helicobacter species infection [65]. The presence of Helicobacter appears to be necessary for subsequent development of colonic cancer in these mice [65].
The transgenic rat model, the HLA-B27 rat is the only transgenic rat model in this class [6667]. HLA-B27 transgenic rats contain multiple inserted integrated copies of the HLA-B27 gene on a Lewis rat (LEW) or Fisher 344 (F344) rat genome background [66]. These animals exhibit a dose responsive significant association with several chronic inflammatory diseases categorized as Spondyloarthropathies (SpA), a complex of diseases that affect the bowel (IBD), joints and axial skeleton (arthritis), and skin, and which involve the major histocompatibility complex class I gene for human leukocyte antigen (HLA) [6667]. At least two different lines of HLA-B27 rats have been developed, the 21-4H lines and the 33-3 lines HLA-B27. Disease does not occur in animals maintained under specific pathogen free conditions. Modulating diet to include increased levels of non-digestible carbohydrates have been shown moderate intestinal microbiome populations and decrease chronic intestinal inflammation in this model [67]. Recent evidence in experimental cell construct has demonstrated that human HLA-B27 misfolds in HLAB2 rat macrophages during these inflammatory episodes [68]. It is noted that increased protein misfolding can act in a proinflammatory manner and that inappropriate unfolded protein responses to normal levels of misfolding can initiate inflammatory pathways [68].
HLA-B27 transgenic rats exhibit UC and CD phenotypes with intestinal inflammation noted within the epithelium of the stomach, large and small intestine, with histological ulceration typical of UC and expression of Th1 cytokines usually associated with CD, including interferon γ, IL2, IL1α, IL1β, TNFα and macrophage inflammatory protein 2 (MIP2) within the bowel mucosa and elevated levels of TNF-α and IL6 within affected rat sera [66]. Experimental rats also exhibit functionally defective dendritic cell populations that is HLA-B27 dose dependent. Clinical signs with associated evidence of histological inflammation include onset of diarrhea after 10 weeks of age, followed by peripheral arthritis. Administration of fructo-oligosaccharides (FOS) followed by examination of bacterial species using 16S RNA prevalence demonstrated decreased the total overall number of bacteria noted in fecal samples. Among the fecal microbiota examined were elevated amounts of Bifidobacteria spp., significantly decreased numbers of Enterobacteriaceae spp. and C. Difficile spp. toxin B. Inulin decreased the level of Lactobacillus spp. group and Clostridia spp. cluster organisms found. All of these effects were less pronounced in cecal microbiota, but the cecal bifidobacterial, clostridial clusters I and IV I FOS treated animals were quite different than those treated with inulin [67]. It appears that 1) Bifidobacteria spp. are consistently and negatively correlated with chronic intestinal inflammation, 2) Bacterioides spp. bacteria can induce colitis in germ free rats but can act to protect against E.coli induced colitis under those conditions also [67]. Bifidobacterium spp. correlated negatively and Cloistridium spp. cluster XI correlated positively with inflammatory bowel disease in these studies [67]. Consequently, the role of this organism in IBD pathogenesis is not clear despite the observation that human IBD patients more frequently carry abundant amounts of Bacterioides spp. organisms within their intestine.
The first human genetic risk factor associated with IBD syndromes was the NOD2 gene (NOD2), which encodes the intracellular receptor for bacterial wall component muramyl dipeptide, and three deficiency models generated by deletion of exons 1, 3, and a frameshift deletion, respectively, have been developed to model IBD [33]. NOD2-deficient mice are susceptible to intestinal inflammation following dextran sodium sulfate chemical injury and exhibit acute intestinal inflammation that is worsened in the absence of normal commensal bacteria, unlike most murine IBD models [33]. NOD2 deficient mice also exhibit altered TLR2 signaling, suggesting that this gene functions in part by inhibiting Th1 cell mediated responses [33]. TLRs are linked to myeloid differentiation factor 88 (MyD 88) [69], and together promote mucosal integrity, barrier function and tissue repair by recognizing and combating the effects of bacterial products prior to injury [70]. TLR2 appears to regulate tight junction barriers found in intestinal stroma, and serve a more protective role [70] while in a Citrobacter rodentium infection-mediated IBD model in TLR chimeric mice, TLR4 was active in recruiting fibroblast like cells to colonic crypts, stimulating apoptosis in that location, stimulating hematopoietic cell populations, and activating STAT3 transcription factors, thus promoting inflammatory changes [70]. Further study in this area is especially warranted, because investigators have suggested that this dual protective and injurious effect incurred by TLR signaling in the bowel may underlie the inability of tumor necrosis factor α antibody therapy to effectively treat a substantial minority of IBD patients, and, subsequent induction of IL11 cytokine and STAT3 signaling [70].
The X-linked gene encoding NF-κB essential modulator (NEMO) is an important regulator of intestinal homeostatic growth, inflammation and tight junctional permeability. It has been suggested that NF-κB activation is a key mediator of mucosal homeostatic barrier function through STAT5 mediated maintenance of zonula occulens protein: cytoskeletal junctional stability and resultant colonic barrier integrity [26]. Absence of STAT5 in stat5IECKO intestinal epithelial cell knockout deficient mice results in increased susceptibility to dextran sodium sulfateinduced chemical colitis and severe ileitis with associated severe inflammation and elevated numbers of CD4+ CD25+ Foxp3+ regulatory T cells, CD4+ T cells, and macrophages accompanied by elevated proinflammatory cytokine expression [26]. In addition, rectal bleeding, shortened colon, slow weight gain, stool quality that was soft to frank diarrhea, and inflamed intestinal epithelial cells were observed [26]. These mice also exhibited increased NF-κB activation in epithelial nuclei accompanied by decreased zonula occulens abundance, upregulation of myosin light chain kinase in epithelial cells, increased focal colonic apoptosis of epithelial cells, and increased epithelial proliferation [26]. The result is a persistent mucosal barrier dysfunction and impairment of mucosal wound healing in this model [26].
IKK-γNEMO/IKKακ deficient mice exhibit severe chronic pancolitis from neonatal life due to conditional targeting of the NRMO regulatory complex governing NF-κB signaling [45]. TLR mediated bacterial recognition is also thought to play a major role in crypt destruction exhibited by NEMOIEC-/- knockout mice [45]. Similarly, NEMO mice also express differences in expression of myosin light chain kinase, which modifies epithelial barrier function and promotes intestinal inflammation [26]. Dual knockout mice expressing defective TGFβRII and IL10RII exhibit severe, fulminant ulcerative colitis and Crohn's disease that is similar to that experienced by human patients, and which has been, despite the many types of genetically modified models now available, difficult to replicate in rodent models [54]. It is thought that the cause of the severe colitis is overactive T cell populations; unlike other models, this one can be completely reversed with broad spectrum antibiotics illustrating the importance of the intestinal microbiome in development, progression, severity and cessation of IBD symptomatology and pathogenic changes [54], as well as the efficacy of IL10 and TGFβ pathway synergism in disease pathogenesis [54].
Another example of a dysfunctional mucosal permeability IBD model is the K8-/- keratin null transgenic mouse [71]. Human IBD has been associated with abnormalities in intestinal epithelial cell-specific intermediate filament class keratin 8, 18 and 19 expression [71]. Intermediate filaments are one of three cellular cytoskeleton classes (the other two being microfilaments and microtubules) forming a type and cell-specific strong scaffold within animal cells [71]. It is thought that keratins 8 and 18 colocalize in cells with the cytoplasmic domain of TNFRII where they function to moderate TNF-induced transcription factor signaling and activation [71]. Several different transgenic mice have been constructed that are keratin deficient; one, the K8-/- null transgenic mouse, will develop colitis without additional stimulation and exhibits diarrhea with electrophysiological modifications such as reduced short circuit current production that is thought to be associated with reversed net CL-/- intestinal secretion. In addition, altered epithelial marker expression from basal crypts to apical and lateral locations, and abnormal ion transporter distribution have been noted [71]. Some of these changes are thought to correspond to mistargeted protein translocation, which could promote imbalanced lumen microbiota growth rate and species distributions. Both UC and CD human patients have documented keratin filament mutations, including K8 (K464N), K8 (I63V), K8 (G62C) [26].
The endoplasmic reticulum is the cellular organelle responsible for polypeptide synthesis, posttranslational modification and peptide folding in the process of producing functional proteins [68]. Endoplasmic reticulum stress has been associated with excess levels of proteins with abnormal folding patterns ("misfolded proteins"), a phenomena that is linked with a variety of different sporadic and inherited human diseases including neurodegenerative, developmental, metabolic, carcinogenic, infectious and inflammatory diseases [68]. Intestinal secretory epithelial cells are particularly important in these processes and as described in this article, are frequently deleteriously affected during waxing and waning IBD episodes. As discussed in an in-depth and excellent review, McGuckin and colleagues defined the complex cellular pathophysiologic pathways involving the unfolded protein response (UPR) and endoplasmic reticulum associated protein degradation (ERAD) [68]. These pathways are becoming increasingly important in defining underlying stressors in these types of diseases through induction of endoplasmic reticulum stress related proteins, chaperones, autophagy and protein degradation.
X box-binding protein 1 (XBP1) transcription factor mRNA is the major substrate for inositol-requiring enzyme (IRE) 1-α/β, which is one of several initiating molecule for unfolded protein response pathways [68]. Under normal conditions, IRE1 splices XBP1 mRNA which results in coding for a transcription factor inducing UPR target genes and ERAD molecules. Unspliced XBP1 mRNA codes for a transcription factor that suppresses UPR target genes; accordingly, one measure of UPR activation is the ratio of spliced to unspliced XBP1 mRNA. This is particularly important because the UPR affects translation, protein folding and degradation of misfolded proteins, a complex process under normal conditions that is crucial in normal homeostasis for removal of abnormal proteins and polypeptides whose accumulation can be a significant cause of morbidity and mortality in a diverse array of inherited or spontaneous disease syndromes [68]. Intestinal secretory cells such as colonic goblet which produce mucin, and small intestinal crypt paneth cells, which produce defensins, lysozyme, antimicrobial lectins and collectins and so are extensively involved in protein manufacture, processing and granular storage of proteins that must maintain normal folding patterns for exit from the endoplasmic reticulum and targeting to appropriate cellular locations. Paneth cell metaplasia has been noted with UC and CD, and inflammatory cytokines, PAMP molecules, growth factors and NOD proteins are all known to increase cellular transcription in intestinal secretory cells, thus placing increased stress on endoplasmic reticular systems. McGuckin MA and colleagues propose that ER stress should be considered an emerging pathway in intestinal inflammation that influences disease development, progression, and response by decreasing mucosal barrier integrity and functional effectiveness, and through UPR-initiated proinflammatory signals released by stressed secretory cells [68].
Several different transgenic mice have been proposed as examples of this pathway. X-box binding protein (XBP1) deficient mice are inducible XBP1 UPR transcription factor Cre-recombinase knockout constructs using the villin promoter (Xbp1villinCre) that suppress colitis development and develop abnormal unfolded protein during expression of their intestinal inflammatory episodes [3368]. XBP-1 transgenic mice exhibit paneth and goblet cell apoptosis, spontaneous ileal inflammation [68]. These mice express defective UPR and partial Xbp1 deficiency in the colon resulting in goblet cell loss, absence of spontaneous colitis but increased sensitivity to dextran sodium sulfate colitis induction. Ire1β-/- mice are knockout mice with loss of the Ire1κ UPR endonuclease that cleaves Xbpr mRNA, and were the first transgenic mice to demonstrate linkage between inappropriate UPR and intestinal inflammation [68]. This isoform of Ire1 is expressed in colonic and gastric epithelial cells. Interestingly, Ire1 knockout mice do not develop spontaneous intestinal inflammation but are more sensitive to dextran sodium sulfate induced colitis also.
Agr2-/- mice are germline (Agr2loxP) conditional allele or inducible knockout mice that abolish Muc2 biosynthesis, resulting in spontaneous intestinal inflammation, paneth and goblet cell apoptosis, and severe inflammation [68]. Protein folding is determined in part by primary sequence but also by the presence of intermolecular disulfide bond formation, and reduction of these bonds is necessary to correct misfolded proteins and to remove them from the endoplasmic reticulum. There is a family of isomerases that are important facilitators of this task, the protein disulfide isomerase (PDI) family, of which one member, the anterior gradient 2 (Agr2) isomerase appears to be highly upregulated during endoreticular stress in intestinal cells [68]. AGR2 also is important in stimulating cell proliferation, cell adhesion, motility; and in inhibiting apoptosis [72]. The presence of high levels of Agr2 mRNA and protein is associated with cell proliferation and inhibited apoptosis in gastrointestinal and mammary gland adenocarcinoma [72] while decreased expression in humans is associated with increased risk of UC and CD [72].
Accordingly, Agr2-/- germline knockout mice generated in FVB/NTgN(ACTB-Cre)2Mrt strain mice [73] have abrogated anterior gradient 2 isomerase expression in Muc2 produced in goblet cells, and do not produce mucus within the intestine [73]. There is apparently mild endoplasmic reticulum stress in these mice, which suggests that UPR-mediated mechanisms may cause differentiating goblet cells to cease their production of this mucin [68]. Inducible Agr2 knockout mouse were constructed using a nested approach. These investigators first developed Agr2flox/ mice with deletions of Agr 2 exons 2, 3 and 4 derived from intestinal mRNA by crossing protamine-Cre transgenic mice [7274] with Tg PRM-Cre mice that exhibited Agr2 deletions in the male germline. The resulting heterozygotes were selectively bred to generate Agr 2-/- mice germline deletion mice [7274]. Inducible Agr 2-/- mice were developed using a Rosa26-CreER strain with inducible systemic Cre recombinase activation responsive to tamoxifen. These Tg Tam-Cre transgenic mice crossed with Agr2flox/flox generated Agr2flox/flox and inducible Agr2/ mice [7275]. Transgenic Agr2flox/flox: TgTam-Cre tamoxifen inducible Cre loxP mice were combined, and then induced to stop expressing Agr 2 following tamoxifen injection by stimulating the Cre-loxP system that takes advantage of using nuclear hormonal receptor import mechanisms to import targeted Cre-fusion proteins into the nucleus while avoiding lethality [7276]. In these experiments inducible strain Agr 2 null allele mice exhibited expanded paneth cell populations and ectopic paneth cell locations within intestinal villi, with mature secretory cells that appear to experience severe endoplasmic reticulum stress, resulting in major epithelial damage [6872]. Germline and inducible knockout mouse constructs develop severe terminal ileitis and colitis characterized by neutrophillic infiltrates surrounding crypt base and in submucosal perivascular spaces, peyers patch lymphoid follicular hyperplasia with granulomatous inflammation consisting of large numbers of multinucleated giant cells locater within interfollicular areas [72]. Colonic immunopathology was characterized by the presence of mixed inflammatory infiltrates with isolated lymphoid follicles distributed within the colonic lamina propria [72]. Tamcre control mice did not exhibit these severe ileocolonic pathological lesions [72]. Paneth cells exhibited cell hypertrophy and expansion following induction of decreased Agr2 expression in inducible transgenic mice within 24 hours of tamoxifen injection followed by goblet cell degeneration, acute neutrophillic inflammatory infiltrates into the lamina propria, further loss of paneth cells, villus enterocyte blunting [72]. These histopathologic changes correlate with increasing apoptosis within all regions of the small intestine and colon [72].
While there is limited biochemical evidence for ER stress and UPR initiation influences in UC and CD, it has been suggested through genome wide association studies that autophagy allele are directly linked with CD (ATG16L1 and IRGM1) and UC (ATG16L1), and that NOD2, which is strongly linked to ileal CD, activates autophagy processes [68]. The presence of these factors could result in dysbiosis, or loss of intestinal homeostasis, and a tendency towards ongoing inflammatory reactivity result in UC and CD [68]. Because there are limited investigative efforts currently in this are exploration of the role of ER stress could potentially be a fruitful area for future examination of mechanisms of disease and potential therapeutic targets within these cellular pathways.
Bridging Integrator (BinI) transgenic mice exhibit modified intestinal permeability through the action on intestinal tight junctional maintenance [77]. Interestingly, ablating BIN1 does not appear to modify histological appearance of tissues alone, but does increase functional resistance of mice to action by dextran sodium sulfateinduced colitis: treated mice exhibited increased survival, and decreased clinical manifestations of IBD such as hematochezia, diarrhea, and weight loss that was specifically associated with immune dysfunction, and not with general disruption of tight junction permeability as documented by treatment of control mice with phorbol ester or sodium caprate [77].
Interleukin 23 (IL23) is an important factor in developing IBD in engineered rodent models but it is not yet fully understood how this signaling pathway affects IBD onset, progression, or cessation [2545]. An intact IL23 signaling pathway is required for intestinal inflammation development in IBD mouse models and human [42]. IL23/Th17 cell pathways have been implicated in IBD pathogenesis during human genetic investigations, suggesting that mucosal epithelial defense is a key component of disease protection [33]. Important functional partners in regulatory networks that govern mucosal immunity in response to commensal bacterial populations are Th17 cell function and CD4+ T reg Fox 3+ cell function [33]. In addition, IL12B (p40) forms a core basis of IL12B and IL23; JAK 2 binds and causes phosphorylation of IL23R, which then results in STAT3 activation, phosphorylation and nuclear translocation [66178]. IL12 was previously thought to be an essential component in IBD pathogenesis involving both adaptive and innate immunities but more recent studies have shown that it is IL23 that is crucial. Using IL23p19 knockout mice and Il12p35 knockout mice to assess the role of each of these cytokines in IBD models has demonstrated the IL12 is an essential mediator of disease expression [33].
Spontaneous transmural enterocolitis and colonic adenocarcinoma are also observed in mice carrying specific disruption and concomitant deficiency of the signal transducer and activator of transcription 3 (STAT3) in STAT3 deficiency transgenic mice [4578]. STAT3 inducible deficient mice are generated using an intercross between the MX-1 Cre transgenic mouse with Cre recombinase controlled by the interferon-responsive Mx1 promoter, and, STAT2 "floxed" allele strain mice, with experimental adult M+/STAT3fl/fl mice carrying the STAT3 allele deletion, and MX-/STAT3fl/fl mice serving as controls [78]. Following type 1 interferon stimulation by repeated intraperitoneal injections of synthetic double stranded RNA immunostimulant preparation polyinosinic: polycytidylic acid (pIpC) [7980] exhibit a clinical wasting syndrome that is associated with severe fatal enterocolitis within 2 to 3 weeks after treatment [78]. Experimental mice exhibited severe dehydration, lethargy, weight loss, diarrhea, anal bleeding and prolapse by day 11 and were typically sacrificed by day 17 due to significant morbidity. Clinicopathological indices included elevated circulating monocytes and neutrophils; multifocal macrophage and lymphocytic aggregates in peritoneal tissues; and colon enlargement, hematochezia and diarrhea. Histologic examination revealed the presence of mixed cell infiltrates consisting of lymphocytes and neutrophils that extended into the lamina propria and submucosa of the colon and were accompanied by ulceration, bleeding, lymphoid follicles, crypt abcesses with increased epithelial mitoses and ultimately destruction of intestinal glands and secretory cell populations. Lesions were more severe in the rectum than in more proximal colonic segments. Immunohistochemical evaluation identified predominantly CD4+ T lymphocytes among the lymphoid population, accompanied by proinflammatory cytokines and endothelial adhesion molecules in areas of reactive cell infiltration: IL-1β, TNFα, interferon, vascular cell adhesion molecule-1 (VCAM-1) and inflammatory cell adhesion molecule-1 (ICAM-1); and, monocyte chemoattractant protein-1 and nitric oxide [78]. STAT3 expression was detected in inflammatory infiltrate and colonic epithelial cell nuclei where it is constitutively phosphorylated and was noted for a slightly extended period following the first pIpC stimulant injection only. The disease course in this model could not be modified with antibiotic treatment, but was obliterated by neutralizing IL12/IL23 effects using anti-p40 antibodies, including the well documented role that this molecule plays in Th1 responses as well as suggesting that IL12 may stimulate natural killer effector cells and endothelial ICAM and VCAM expression, contributing to the overall immune dysbiosis found in IBD disease and animal models.
STAT3, while considered to be a nonspecific transcription factor, will bind downstream of many inflammatory cytokines that exhibit opposing actions, as well as inducing IL23R expression and Th17 differentiation. This complex combination of actions involving both pro- and anti-inflammatory mechanisms are undoubtedly activated in IBD, reflecting the presence of a broadly inflammatory environment as well as in STAT3 deficiency mouse models, demonstrating the suppressive/regulatory effects of this transcription factor [7]. Similarly, STAT4 is specifically associated with IL12/IL23 receptor signaling, and increased STAT4 expression through construction of overexpression transgenic mice under the control of cytomegalovirus promotor systems targeting elevated STAT4 levels to splenic and lamina propria CD4+ T cells [45] When immunostimulated with hapten-linked antigens these mice exhibit severe transmural colitis that is thought to be in part due to abnormal activation of T helper 1 cell pathways.
Many of the transgenic strains were developed to assess mechanisms of inflammation, injury and repair. One example of this type of model is the IL10 transgenic mice; TCRα-/- transgenic mice. These mice develop severe mucosal inflammation at 12-16 weeks of age, possibly due to Th2 cell dysregulation [45]. Recent studies have also identified familial early-onset Crohn's disease in some human populations that is associated with homozygous IL-10 receptor mutations present in hematopoietic cells, based on disease rescue observed following allogeneic stem cell transplantation in human patients. In mice transgenic mice IL10-/-, IL10rb-/- and in those carrying a Stat3 in circulating macrophages individuals develop spontaneous intestinal inflammation [5981]. IL10-/- mice exhibit a significant increase in splenic macrophages when generated on Non-Obese Diabetic (NOD) strain backgrounds, but not on C57B6 genetic strain backgrounds. NOD based IL10 deficient mice housed under conventional husbandry conditions also exhibited high levels of rectal prolapse and histological evidence of colitis in comparison to control mice, along with abrogation of spontaneous diabetes and reduced sialoadenitis usually observed in a subset of NOD mice housed in conventional facilities [81]. Using TLR CpG motif oligonucleotides as agonists in this IL-10 deficient NOD model resulted in elevated proinflammatory sera levels for TNFα, interferon γ, and IL7 while IL12p70 was unaffected (Rajagopalan G et al 2006).
IL10 is thought to be an important factor in regulating aspects of intestinal homeostasis. Actually, mice that are IL10 deficient as well as those that have been treated with anti-IL10 antibody both develop significant chronic intestinal inflammation [27]. Similarly, IL10 supplementation is protective against colitis disease development and appears to be associated with TGFβ1 potentiation of activated T cell effector and regulatory CD4+ T cell populations [27]. Also, in IL10-/- double null mice, tumor necrosis factor inducible nitric oxide synthase (iNOS) appears to activate the intestinal epithelial cell apoptosis pathway normally responsible for crypt replenishment of epithelial bowel cells, contributing to increased epithelial degeneration and colitis in humans afflicted with IBD, and in these transgenic mice constructs [23]. SAMP1/Yit mice exhibit spontaneous colitis without genetic manipulation which is also thought to be mediated through Stat3 activation pathways [2261]. STAT3 expression is also important for epithelial restitution and enterocyte survival with induced somatic inactivation of this gene resulting in fulminant colitis [78]. Expression of epithelial E-cadherin (CD324, associated with UC gene CDH1, and clinically, UC) mediates adhesion between epithelial cells and formation of an effective barrier. An interruption of these fundamental homeostatic mechanisms can predispose towards developing IBD. E-cadherin is expressed in dendritic cells that are considered to be important in maintaining a balance between self-tolerance and expression of autoimmunity through intestinal portals [82]. The IL23 pathway appears to be responsible for the onset and progression of spontaneous colitis in IL10 deficient mice [64783]. Multiple murine colitis models have been identified with IL23 pathway-mediated colitis due to expression blockade or deficiency [25]. Additionally, both IL-10 deficient mice and Gαi2 mice, which are missing G protein subunit Gαi2 that normally regulates the inhibitory control of adenylyl cyclase, and negatively regulate TLR2 and TLR4-induced cytokine expression with subsequent decreased TNFα and IL6 expression [43], have been associated with colon cancer development [84].
As a result of many recent large scale, individual and collaborative genome wide association scanning studies assessing IBD patients and experimental models for disease correlation with susceptibility loci, the number of known association loci has increased from 3 to 100 [34]. Now that genetic susceptibility data have been categorized and identified it is time to put this information into a clinical context through translational medicine, with a goal of finding appropriate cellular and molecular targets for therapeutic intervention, and, to better understand this complex disease pathogenesis at the level of the nucleic acid, protein assembly, microbiomic, metagenomic and ecological levels. The proliferation of different genetically engineered rodent IBD models which produce different aspects of this disease syndrome it would be prudent to consider areas in which less has yet been clearly described in the pathogenesis of the IBD syndromes, but which appear to greatly influence disease outcomes and patient therapeutic responses. One important aspect going forward in this line of research would be to ensure that rodent models under development and evaluation as putative IBD animal models exhibit well characterized intestinal microbiome prior to induction of this disease [85]. In particular, models should be negative for Helicobacter spp. infection, as this infection with this organism has been clearly associated with IBD disease expression in one transgenic rodent model [86]. Equally important is appropriate institutional animal care and use committee (IACUC) attention to investigator knowledge, experience and consideration of the medical implications involved in developing these types of animals' models. It will be very important to make provision for adequate veterinary care and animal husbandry aspects involving accommodation of induced putative deficits in activities of daily living (such as motor ability, food and water ingestion, and bodily functions); and, assessment of discomfort and pain as several of the IBD transgenic murine models induce significant morbidity, and for some strains, mortality.
These studies also suggest that it is important to further delineate gut microbiota as a way of characterizing conventional and specific pathogen free facilities [44], including those in which serological testing has suggested that common mouse and rat pathogens and commensal organisms are absent. Epigenetic, environmental (microbiome, metabolome) and nutritional factors are important in IBD pathogenesis, and evaluating ways in which they influence disease expression represent potential investigative approaches with the greatest potential for new discoveries. These iterative approaches may produce new insights into underlying pathogenic mechanisms affecting IBD syndrome onset, progression, and cessation as observed in human and animal patients, and in animal disease models.
References
1. Beatty PL. MUC1 in the relationship between inflammation and cancer in IBD. Dissertation. University of Pittsburgh;2006.
2. Kaser A, Zeissig S, Blumberg RS. Inflammatory bowel disease. Annu Rev Immunol. 2010; 28:573–621. PMID: 20192811.


3. Weaver CT, Elson CO, Fouser LA, Kolls JK. The Th17 pathway and inflammatory diseases of the intestines, lungs, and skin. Annu Rev Pathol. 2013; 8:477–512. PMID: 23157335.


4. Tomasello G, Tralongo P, Damiani P, Sinagra E, Di Trapani B, Zeenny MN, Hussein IH, Jurjus A, Leone A. Dismicrobism in inflammatory bowel disease and colorectal cancer: changes in response of colocytes. World J Gastroenterol. 2014; 20(48):18121–18130. PMID: 25561781.


5. Hajj-Hussein IA, Jurjus R, Saliba J, Ghanem S, Diab R, Bou Assi T, Daouk H, Leone A, Jurjus A. Modulation of Beta2 and Beta3 integrins in experimental colitis induced by iodoacetamide and enteropathogenic E. coli. J Biol Regul Homeost Agents. 2013; 27(2):351–363. PMID: 23830386.
6. Khanna PV, Shih DQ, Haritunians T, McGovern DP, Targan S. Use of animal models in elucidating disease pathogenesis in IBD. Semin Immunopathol. 2014; 36(5):541–551. PMID: 25212688.


7. Li Y, de Haar C, Chen M, Deuring J, Gerrits MM, Smits R, Xia B, Kuipers EJ, van der Woude CJ. Disease-related expression of the IL6/STAT3/SOCS3 signalling pathway in ulcerative colitis and ulcerative colitis-related carcinogenesis. Gut. 2010; 59(2):227–235. PMID: 19926618.


8. Monteleone G, Pallone F, Stolfi C. The dual role of inflammation in colon carcinogenesis. Int J Mol Sci. 2012; 13(9):11071–11084. PMID: 23109839.


9. Neurath MF. Animal models of inflammatory bowel diseases: illuminating the pathogenesis of colitis, ileitis and cancer. Dig Dis. 2012; 30:91–94. PMID: 23075875.


10. Kolodziejska-Sawerska A, Rychlik A, Depta A, Wdowiak M, Nowicki M, Kander M. Cytokines in canine inflammatory bowel disease. Pol J Vet Sci. 2013; 16(1):165–171. PMID: 23691593.
11. Wdowiak M, Rychlik A, Kołodziejska-Sawerska A. Biomarkers in canine inflammatory bowel disease diagnostics. Pol J Vet Sci. 2013; 16(3):601–610. PMID: 24195302.


12. García-Sancho M, Rodríguez-Franco F, Sainz A, Mancho C, Rodríguez A. Evaluation of clinical, macroscopic, and histopathologic response to treatment in nonhypoproteinemic dogs with lymphocytic-plasmacytic enteritis. J Vet Intern Med. 2007; 21(1):11–17. PMID: 17338144.
13. Day MJ, Bilzer T, Mansell J, Wilcock B, Hall EJ, Jergens A, Minami T, Willard M, Washabau R. World Small Animal Veterinary Association Gastrointestinal Standardization Group. Histopathological standards for the diagnosis of gastrointestinal inflammation in endoscopic biopsy samples from the dog and cat: a report from the World Small Animal Veterinary Association Gastrointestinal Standardization Group. J Comp Pathol. 2008; 138:S1–S43. PMID: 18336828.


14. Whitley NT, Day MJ. Immunomodulatory drugs and their application to the management of canine immune-mediated disease. J Small Anim Pract. 2011; 52(2):70–85. PMID: 21265846.


15. Kaikkonen R, Niinistö K1, Sykes B, Anttila M, Sankari S, Raekallio M. Diagnostic evaluation and short-term outcome as indicators of long-term prognosis in horses with findings suggestive of inflammatory bowel disease treated with corticosteroids and anthelmintics. Acta Vet Scand. 2014; 56:35. PMID: 24894126.


16. Kanthaswamy S, Elfenbein HA, Ardeshir A, Ng J, Hyde D, Smith DG, Lerche N. Familial aggregation of chronic diarrhea disease (CDD) in rhesus macaques (Macaca mulatta). Am J Primatol. 2014; 76(3):262–270. PMID: 24532180.


17. Over K, Crandall PG, O'Bryan CA, Ricke SC. Current perspectives on Mycobacterium avium subsp. paratuberculosis, Johne's disease, and Crohn's disease: a review. Crit Rev Microbiol. 2011; 37(2):141–156. PMID: 21254832.
18. Jurjus AR, Khoury NN, Reimund JM. Animal models of inflammatory bowel disease. J Pharmacol Toxicol Methods. 2004; 50(2):81–92. PMID: 15385082.


19. Khan J, Islam MN. Morphology of the intestinal barrier in different physiological and pathological conditions. Histopathology-Reviews and Recent Advances. Rijeka, Croatia: Intech Publishers;2012. p. 133–152.
20. Reimund JM, Tavernier M, Viennot S, Hajj Hussein IA, Dupont B, Justum A-PM, Jurus AR, Freund JN, Lechevrel M. Ulcerative colitis-associated colorectal cancer prevention by 5-Aminosalicylates: current status and perspectives. Rijeka, Croatia: Intech Publishers;www.inotechopen.com.
21. DeVoss J, Diehl L. Murine models of inflammatory bowel disease (IBD): challenges of modeling human disease. Toxicol Pathol. 2014; 42(1):99–110. PMID: 24231829.
22. Pizarro TT, Arseneau KO, Cominelli F. Lessons from genetically engineered animal models XI. Novel mouse models to study pathogenic mechanisms of Crohn's disease. Am J Physiol Gastrointest Liver Physiol. 2000; 278(5):G665–G669. PMID: 10801257.
23. Goretsky T, Dirisina R, Sinh P, Mittal N, Managlia E, Williams DB, Posca D, Ryu H, Katzman RB, Barrett TA. p53 mediates TNF-induced epithelial cell apoptosis in IBD. Am J Pathol. 2012; 181(4):1306–1315. PMID: 22863952.


24. Li Z, Arijs I, De Hertogh G, Vermeire S, Noman M, Bullens D, Coorevits L, Sagaert X, Schuit F, Rutgeerts P, Ceuppens JL, Van Assche G. Reciprocal changes of Foxp3 expression in blood and intestinal mucosa in IBD patients responding to infliximab. Inflamm Bowel Dis. 2010; 16(8):1299–1310. PMID: 20196149.


25. Abraham C, Cho JH. IL-23 and autoimmunity: new insights into the pathogenesis of inflammatory bowel disease. Annu Rev Med. 2009; 60:97–110. PMID: 18976050.


26. Gilbert S, Zhang R, Denson L, Moriggl R, Steinbrecher K, Shroyer N, Lin J, Han X. Enterocyte STAT5 promotes mucosal wound healing via suppression of myosin light chain kinasemediated loss of barrier function and inflammation. EMBO Mol Med. 2012; 4(2):109–124. PMID: 22228679.


27. Gad M. Regulatory T cells in experimental colitis. Curr Top Microbiol Immunol. 2005; 293:179–208. PMID: 15981481.


28. Mizoguchi A, Andoh A. Animal models of inflammatory bowel disease for drug discovery. Chapter 22. Animal Models of the Study of Human Disease. Elsevier Publishers;2013. p. 499–527.
29. Parlato M, Yeretssian G2. NOD-like receptors in intestinal homeostasis and epithelial tissue repair. Int J Mol Sci. 2014; 15(6):9594–9627. PMID: 24886810.


30. Strober W, Murray PJ, Kitani A, Watanabe T. Signalling pathways and molecular interactions of NOD1 and NOD2. Nat Rev Immunol. 2006; 6(1):9–20. PMID: 16493424.


31. Denzel A, Horejsi V, Hayday A. The 5th EFIS Tatra Immunology Conference on 'Molecular determinants of T cell immunity' held in the High Tatra Mountains, Slovakia, September 7-11, 2002. Immunol Lett. 2003; 86(1):1–6. PMID: 12600738.


32. Hajj Hussein IA, Tohme R, Barada K, Mostafa MH, Freund JN, Jurjus RA, Karam W, Jurjus A. Inflammatory bowel disease in rats: bacterial and chemical interaction. World J Gastroenterol. 2008; 14(25):4028–4039. PMID: 18609687.
33. Mizoguchi A, Mizoguchi E. Animal models of IBD: linkage to human disease. Curr Opin Pharmacol. 2010; 10(5):578–587. PMID: 20860919.


34. Lee JC, Parkes M. 100 genes for IBD...whatever next!? Inflamm Bowel Dis. 2011; 11(3):103–111.
35. Cho JH, Abraham C. Inflammatory bowel disease genetics: Nod2. Annu Rev Med. 2007; 58:401–416. PMID: 16987083.


36. Inohara , Chamaillard , McDonald C, Nuñez G. NOD-LRR proteins: role in host-microbial interactions and inflammatory disease. Annu Rev Biochem. 2005; 74:355–383. PMID: 15952891.


37. Palacios-Rodríguez Y, García-Laínez G, Sancho M, Gortat A, Orzáez M, Pérez-Payá E. Polypeptide modulators of caspase recruitment domain (CARD)-CARD-mediated protein-protein interactions. J Biol Chem. 2011; 286(52):44457–44466. PMID: 22065589.


38. Wilmanski JM, Petnicki-Ocwieja T, Kobayashi KS. NLR proteins: integral members of innate immunity and mediators of inflammatory diseases. J Leukoc Biol. 2008; 83(1):13–30. PMID: 17875812.


39. Netea MG, Van der Graaf C, Van der Meer JW, Kullberg BJ. Recognition of fungal pathogens by Toll-like receptors. Eur J Clin Microbiol Infect Dis. 2004; 23(9):672–676. PMID: 15322932.


40. Oshiumi H, Matsuo A, Matsumoto M, Seya T. Pan-vertebrate toll-like receptors during evolution. Curr Genomics. 2008; 9(7):488–493. PMID: 19506737.


41. Hallman M, Rämet M, Ezekowitz RA. Toll-like receptors as sensors of pathogens. Pediatr Res. 2001; 50(3):315–321. PMID: 11518816.


42. Carty M, Bowie AG. Recent insights into the role of Toll-like receptors in viral infection. Clin Exp Immunol. 2010; 161(3):397–406. PMID: 20560984.


43. Li P, Neubig RR, Zingarelli B, Borg K, Halushka PV, Cook JA, Fan H. Toll-like receptor-induced inflammatory cytokines are suppressed by gain of function or overexpression of Gα(i2) protein. Inflammation. 2012; 35(5):1611–1617. PMID: 22581266.
44. Bleich A, Hansen AK. Time to include the gut microbiota in the hygienic standardisation of laboratory rodents. Comp Immunol Microbiol Infect Dis. 2012; 35(2):81–92. PMID: 22257867.


45. Wirtz S, Neurath MF. Mouse models of inflammatory bowel disease. Adv Drug Deliv Rev. 2007; 59(11):1073–1083. PMID: 17825455.


46. Goyal N, Rana A, Ahlawat A, Bijjem KR, Kumar P. Animal models of inflammatory bowel disease: a review. Inflammopharmacology. 2014; 22(4):219–233. PMID: 24906689.


47. Bilsborough J, Viney JL. From model to mechanism: lessons of mice and men in the discovery of protein biologicals for the treatment of inflammatory bowel disease. Expert Opin Drug Discov. 2006; 1(1):69–83. PMID: 23506033.


48. Siddiqui KR, Laffont S, Powrie F. E-cadherin marks a subset of inflammatory dendritic cells that promote T cell-mediated colitis. Immunity. 2010; 32(4):557–567. PMID: 20399121.


49. Bassaganya-Riera J, DiGuardo M, Climent M, Vives C, Carbo A, Jouni ZE, Einerhand AW, O'Shea M, Hontecillas R. Activation of PPARγ and δ by dietary punicic acid ameliorates intestinal inflammation in mice. Br J Nutr. 2011; 106(6):878–886. PMID: 21736821.


50. Nguyen DD, Esteon MA, Muthyupalani S, Mobley MW, Potter AF, Taylor NS, Snapper SB, Fox JG. T1792 Helicobacter is Required for Colitis in WASP-Deficient Mice and Induces Colon Cancer. Gastroenterology. 2010; 138(5):Suppl 1. S579–S580.


51. Hahm KB, Im YH, Parks TW, Park SH, Markowitz S, Jung HY, Green J, Kim SJ. Loss of transforming growth factor beta signalling in the intestine contributes to tissue injury in inflammatory bowel disease. Gut. 2001; 49(2):190–198. PMID: 11454793.
52. Hahm KB, Lee KM, Kim YB, Hong WS, Lee WH, Han SU, Kim MW, Ahn BO, Oh TY, Lee MH, Green J, Kim SJ. Conditional loss of TGF-beta signalling leads to increased susceptibility to gastrointestinal carcinogenesis in mice. Aliment Pharmacol Ther. 2002; 16(Suppl 2):115–127. PMID: 11966532.
53. Seamons A, Treuting PM, Brabb T, Maggio-Price L. Characterization of dextran sodium sulfate-induced inflammation and colonic tumorigenesis in Smad3(-/-) mice with dysregulated TGFβ. PLoS One. 2013; 8(11):e79182. PMID: 24244446.


54. Kang SS, Bloom SM, Norian LA, Geske MJ, Flavell RA, Stappenbeck TS, Allen PM. An antibiotic-responsive mouse model of fulminant ulcerative colitis. PLoS Med. 2008; 5(3):e41. PMID: 18318596.


55. Spencer SD, Di Marco F, Hooley J, Pitts-Meek S, Bauer M, Ryan AM, Sordat B, Gibbs VC, Aguet M. The orphan receptor CRF2-4 is an essential subunit of the interleukin 10 receptor. J Exp Med. 1998; 187(4):571–578. PMID: 9463407.


56. Keffer J, Probert L, Cazlaris H, Georgopoulos S, Kaslaris E, Kioussis D, Kollias G. Transgenic mice expressing human tumour necrosis factor: a predictive genetic model of arthritis. EMBO J. 1991; 10(13):4025–4031. PMID: 1721867.


57. Kontoyiannis D, Pasparakis M, Pizarro TT, Cominelli F, Kollias G. Impaired on/off regulation of TNF biosynthesis in mice lacking TNF AU-rich elements: implications for joint and gut-associated immunopathologies. Immunity. 1999; 10(3):387–398. PMID: 10204494.
58. Kontoyiannis D, Boulougouris G, Manoloukos M, Armaka M, Apostolaki M, Pizarro T, Kotlyarov A, Forster I, Flavell R, Gaestel M, Tsichlis P, Cominelli F, Kollias G. Genetic dissection of the cellular pathways and signaling mechanisms in modeled tumor necrosis factor-induced Crohn's-like inflammatory bowel disease. J Exp Med. 2002; 196(12):1563–1574. PMID: 12486099.


59. Hale LP, Greer PK. A novel murine model of inflammatory bowel disease and inflammation-associated colon cancer with ulcerative colitis-like features. PLoS One. 2012; 7(7):e41797. PMID: 22848611.


60. Strober W, Nakamura K, Kitani A. The SAMP1/Yit mouse: another step closer to modeling human inflammatory bowel disease. J Clin Invest. 2001; 107(6):667–670. PMID: 11254665.


61. Mitsuyama K, Matsumoto S, Rose-John S, Suzuki A, Hara T, Tomiyasu N, Handa K, Tsuruta O, Funabashi H, Scheller J, Toyonaga A, Sata M. STAT3 activation via interleukin 6 transsignalling contributes to ileitis in SAMP1/Yit mice. Gut. 2006; 55(9):1263–1269. PMID: 16682432.


62. Pizarro TT, Pastorelli L, Bamias G, Garg RR, Reuter BK, Mercado JR, Chieppa M, Arseneau KO, Ley K, Cominelli F. SAMP1/YitFc mouse strain: a spontaneous model of Crohn's disease-like ileitis. Inflamm Bowel Dis. 2011; 17(12):2566–2584. PMID: 21557393.


63. Mueller C. Tumour necrosis factor in mouse models of chronic intestinal inflammation. Immunology. 2002; 105(1):1–8. PMID: 11849309.


64. Williams HR, Cox IJ, Walker DG, Cobbold JF, Taylor-Robinson SD, Marshall SE, Orchard TR. Differences in gut microbial metabolism are responsible for reduced hippurate synthesis in Crohn's disease. BMC Gastroenterol. 2010; 10:108. PMID: 20849615.


65. Nguyen DD, Esteon MA, Muthyupalani S, Mobley MW, Potter AF, Taylor NS, Snapper SB, Fox JG. T1792 Helicobacter is Required for Colitis in WASP-Deficient Mice and Induces Colon Cancer. Gastroenterology. 2010; 138(5):Suppl 1. S579–S580.


66. Milia AF, Ibba-Manneschi L, Manetti M, Benelli G, Messerini L, Matucci-Cerinic M. HLA-B27 transgenic rat: an animal model mimicking gut and joint involvement in human spondyloarthritides. Ann N Y Acad Sci. 2009; 1173:570–574. PMID: 19758201.
67. Koleva PT, Valcheva RS, Sun X, Gänzle MG, Dieleman LA. Inulin and fructo-oligosaccharides have divergent effects on colitis and commensal microbiota in HLA-B27 transgenic rats. Br J Nutr. 2012; 108(9):1633–1643. PMID: 22243836.


68. McGuckin MA, Eri RD, Das I, Lourie R, Florin TH. ER stress and the unfolded protein response in intestinal inflammation. Am J Physiol Gastrointest Liver Physiol. 2010; 298(6):G820–G832. PMID: 20338921.


69. Buchholz BM, Billiar TR, Bauer AJ. Dominant role of the MyD88-dependent signaling pathway in mediating early endotoxin-induced murine ileus. Am J Physiol Gastrointest Liver Physiol. 2010; 299(2):G531–G538. PMID: 20508155.


70. Gibson DL, Montero M, Ropeleski MJ, Bergstrom KS, Ma C, Ghosh S, Merkens H, Huang J, Månsson LE, Sham HP, McNagny KM, Vallance BA. Interleukin-11 reduces TLR4-induced colitis in TLR2-deficient mice and restores intestinal STAT3 signaling. Gastroenterology. 2010; 139(4):1277–1288. PMID: 20600022.


71. Majumdar D, Tiernan JP, Lobo AJ, Evans CA, Corfe BM. Keratins in colorectal epithelial function and disease. Int J Exp Pathol. 2012; 93(5):305–318. PMID: 22974212.


72. Zhao F, Edwards R, Dizon D, Afrasiabi K, Mastroianni JR, Geyfman M, Ouellette AJ, Andersen B, Lipkin SM. Disruption of Paneth and goblet cell homeostasis and increased endoplasmic reticulum stress in Agr2-/- mice. Dev Biol. 2010; 338(2):270–279. PMID: 20025862.


73. Park SW, Zhen G, Verhaeghe C, Nakagami Y, Nguyenvu LT, Barczak AJ, Killeen N, Erle DJ. The protein disulfide isomerase AGR2 is essential for production of intestinal mucus. Proc Natl Acad Sci U S A. 2009; 106(17):6950–6955. PMID: 19359471.


74. O'Gorman S, Dagenais NA, Qian M, Marchuk Y. Protamine-Cre recombinase transgenes efficiently recombine target sequences in the male germ line of mice, but not in embryonic stem cells. Proc Natl Acad Sci U S A. 1997; 94(26):14602–14607. PMID: 9405659.
75. Badea TC, Wang Y, Nathans J. A noninvasive genetic/pharmacologic strategy for visualizing cell morphology and clonal relationships in the mouse. J Neurosci. 2003; 23(6):2314–2322. PMID: 12657690.


76. Garcia EL, Mills AA. Getting around lethality with inducible Cremediated excision. Semin Cell Dev Biol. 2002; 13(2):151–158. PMID: 12127267.


77. Ryu H, Posca D, Barrett T. Bin1: a new player in IBD barrier dysfunction. Dig Dis Sci. 2012; 57(7):1751–1753. PMID: 22644742.


78. Alonzi T, Newton IP, Bryce PJ, Di Carlo E, Lattanzio G, Tripodi M, Musiani P, Poli V. Induced somatic inactivation of STAT3 in mice triggers the development of a fulminant form of enterocolitis. Cytokine. 2004; 26(2):45–56. PMID: 15050604.


79. Goodbourn S, Maniatis T. Overlapping positive and negative regulatory domains of the human beta-interferon gene. Proc Natl Acad Sci U S A. 1988; 85(5):1447–1451. PMID: 3422743.


80. Kandimalla ER, Yu D, Zhao Q, Agrawal S. Effect of chemical modifications of cytosine and guanine in a CpG-motif of oligonucleotides: structure-immunostimulatory activity relationships. Bioorg Med Chem. 2001; 9(3):807–813. PMID: 11310616.


81. Rajagopalan G, Kudva YC, Sen MM, Marietta EV, Murali N, Nath K, Moore J, David CS. IL-10-deficiency unmasks unique immune system defects and reveals differential regulation of organ-specific autoimmunity in non-obese diabetic mice. Cytokine. 2006; 34(1-2):85–95. PMID: 16740391.


82. Siddiqui KR, Laffont S, Powrie F. E-cadherin marks a subset of inflammatory dendritic cells that promote T cell-mediated colitis. Immunity. 2010; 32(4):557–567. PMID: 20399121.


83. Villarino AV, Artis D, Bezbradica JS, Miller O, Saris CJ, Joyce S, Hunter CA. IL-27R deficiency delays the onset of colitis and protects from helminth-induced pathology in a model of chronic IBD. Int Immunol. 2008; 20(6):739–752. PMID: 18375937.


84. Chen JF, Guo JH, Moxham CM, Wang HY, Malbon CC. Conditional, tissue-specific expression of Q205L G alpha i2 in vivo mimics insulin action. J Mol Med (Berl). 1997; 75(4):283–289. PMID: 9151214.
85. Schoeb TR, Bullard DC. Microbial and histopathologic considerations in the use of mouse models of inflammatory bowel diseases. Inflamm Bowel Dis. 2012; 18(8):1558–1565. PMID: 22294506.


86. Chichlowski M, Sharp JM, Vanderford DA, Myles MH, Hale LP. Helicobacter typhlonius and Helicobacter rodentium differentially affect the severity of colon inflammation and inflammationassociated neoplasia in IL10-deficient mice. Comp Med. 2008; 58(6):534–541. PMID: 19149410.
87. McNamee EN, Wermers JD, Masterson JC, Collins CB, Lebsack MD, Fillon S, Robinson ZD, Grenawalt J, Lee JJ, Jedlicka P, Furuta GT, Rivera-Nieves J. Novel model of TH2-polarized chronic ileitis: the SAMP1 mouse. Inflamm Bowel Dis. 2010; 16(5):743–752. PMID: 19856411.


88. Luedde T, Schwabe RF. NF-κB in the liver--linking injury, fibrosis and hepatocellular carcinoma. Nat Rev Gastroenterol Hepatol. 2011; 8(2):108–118. PMID: 21293511.


Table 1
Selected spontaneous and transgenic rodent IBD models
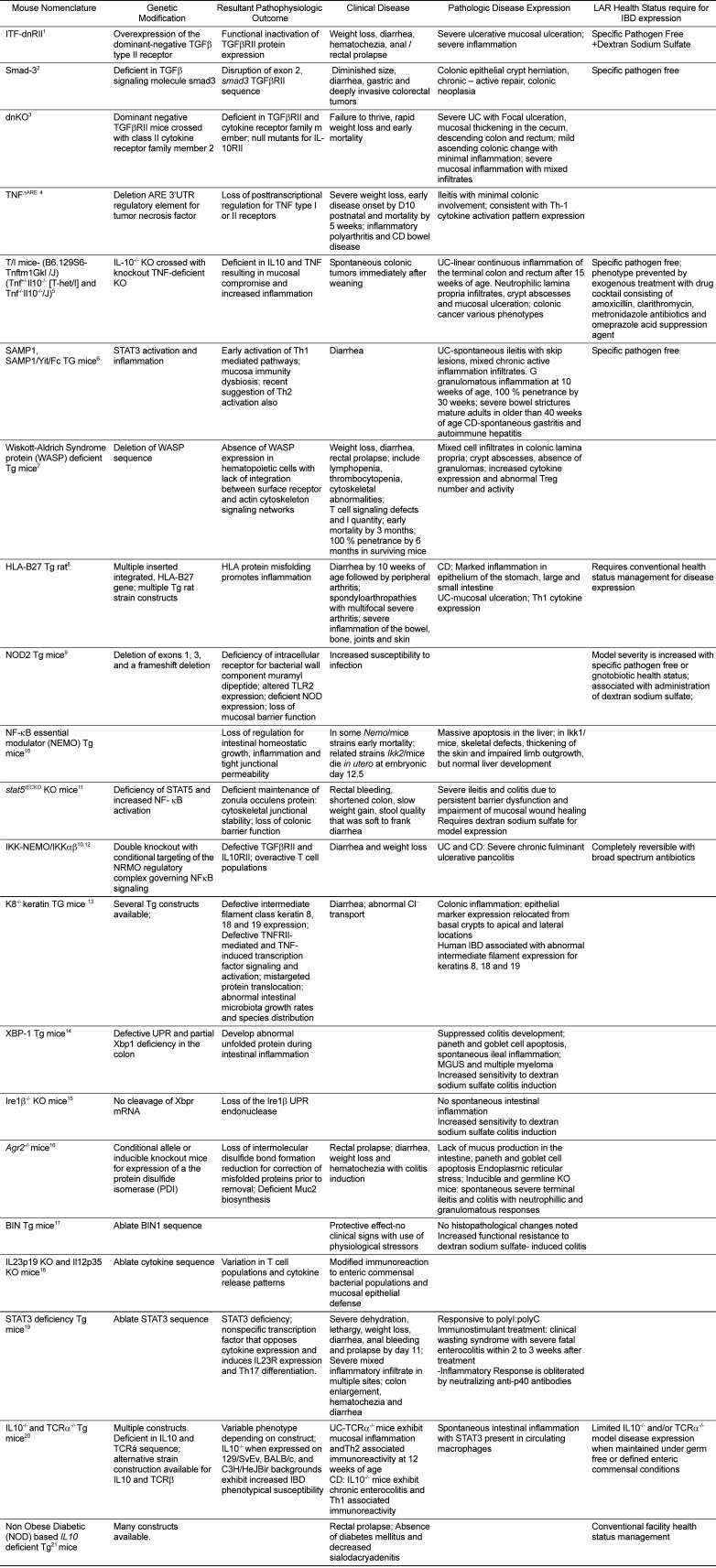
References to each model are as follows: 1[515253]. 2http://jaxmice.jax.org/strain/003451.html; 3[54]; 4[56]; 5http:/jaxmice.jax.org/strain/003008.html; 6[616287]; 7[5065]; 8[68]; 9[33]; 10[264588]; 11[26]; 12[54]; 13[71]; 14[3368]; 15[68], http://www.ncbi.nlm.nih.gov/geo/query/acc.cgi?acc=GSE6980; 16[6872], http://jaxmice.jax.org/strain/025630.html;17[77]; 18[33]; 19[74578]; 20[84559], http://jaxmice.jax.org/list/ra1655.html, http://jaxmice.jax.org/jaxnotes/502/502a.html; 21[859]