Abstract
Inflammation has recently been implicated in cancer formation and progression. As tissue transglutaminase (TG2) has been associated with both inflammatory signaling and tumor cell behavior, we propose that TG2 may be an important link inducing interleukin-6 (IL-6)-mediated cancer cell aggressiveness, including cancer stem cell-like characteristics and distant hematogenous metastasis. We evaluated the effect of differential TG2 and IL-6 expression on in vivo distant metastasis of human ovarian cancer cells. IL-6 production in human ovarian cancer cells was dependent on their TG2 expression levels. The size and efficiency of tumor sphere formation were correlated with TG2 expression levels and were dependent on TG2-mediated IL-6 secretion in human ovarian cancer cells. Primary tumor growth and propagation in the peritoneum and distant hematogenous metastasis into the liver and lung were also dependent on TG2 and downstream IL-6 expression levels in human ovarian cancer cells. In this report, we provide evidence that TG2 is an important link in IL-6-mediated tumor cell aggressiveness, and that TG2 and downstream IL-6 could be important mediators of distant hematogenous metastasis of human ovarian cancer cells. Intervention specific to TG2 and/or downstream IL-6 in ovarian cancer cells could provide a promising means to control tumor metastasis.
Tissue transglutaminase, also known as transglutaminase 2 (TG2), is a ubiquitously expressed enzyme that catalyzes the transamidation reaction producing crosslinked or polyaminated proteins [1]. With a widespread expression pattern and presence both intracellularly and extracellularly, TG2 has been implicated in various biological processes, encompassing cell adhesion, extracellular matrix (ECM) formation, apoptosis, and survival [12]. Not surprisingly, TG2 has been shown to be an important prognostic biomarker for several types of cancer [34] and TG2 expression is correlated with the advanced or aggressive form of cancer and development of drug resistance [45678].
Ovarian cancer is the most lethal malignancy of the female reproductive organs and has a poor 5-year survival rate of less than 40% because it is usually diagnosed at an advanced stage. The most common route of metastasis of ovarian cancer is peritoneal spreading, but distant hematogenous dissemination is not infrequent in patients with advanced disease [9]. The overexpression of TG2 in ovarian cancers was associated with significantly worse overall patient survival in both univariate and multivariate analyses [10]. Transfection of TG2 into SK-OV-3 ovarian cancer cells promoted attachment and spreading on fibronectin and directional cell migration in vitro in a b1 integrin-dependent manner and increased peritoneal tumor dissemination in an in vivo xenograft model [11]. TG2 silencing of ovarian cancer cells with antisense constructs significantly decreased the invasive potential of the cells and peritoneal spreading and also increased cisplatin- or docetaxel-induced cell death [12].
TG2 expressed in tumor cells increased their adhesion to tissue culture matrix and migration [13]. TG2 expression constitutively activated focal adhesion kinase (FAK) by promoting posttranslational PTEN down-regulation that resulted in the activation of cell survival FAK/PI3K/AKT pathway in pancreatic cancer cells [14]. Close approximation of TG2 at the leading edge of cancer cells showed the critical role of TG2 and downstream Rho GTPase in cancer migration and invasion [15]. TG2's role in drug resistance of cancer cells is related its role in activation of nuclear factor-κB (NF-κB) signaling [16]. Expression of TG2 in various cancer types is associated with increased constitutive activation of NF-κB [1718]. TG2 has been reported to mediate polymerization of IκBα and TG2 binding to IκBα, which prevents its interaction with the p65/p52 subunit of NF-κB [19].
Interleukin-6 (IL-6) is an important downstream effector of NF-κB signaling. High serum IL-6 levels correlate with poor disease outcome and reduced clinical prognosis in patients with cancer [2021] and cancer formation in a murine inflammation-associated colon cancer model [22]. In addition to bone marrow-derived cells, IL-6 produced in epithelial cancer cells themselves plays an important role in tumor growth and metastasis in an autocrine and/or paracrine manner. IL-6 signaling in epithelial cancer cells has also been linked to in vivo aggressiveness by affecting the epithelial-to-mesenchymal transition (EMT) [2324] or conferring the cancer stem cell-like properties of these cells [2526]. The key molecular links leading to IL-6 production in epithelial cancer cells, which are correlated with distant metastasis and cancer stem cell-like properties, are currently under active investigation. Recently, we showed that noninfectious stimuli activating the IL-6 signaling lead to fibrosis through TG2 in pulmonary epithelial cells [27]. Because fibrosis and invasion of cancer have common characteristics [28], we propose that TG2 expressed in epithelial cancer cells might provide a critical link leading to IL-6 induction in ovarian cancer cells.
In the present study, we evaluated the importance of the TG2-NF-κB-IL-6 axis in ovarian cancer cell aggressiveness in vivo. TG2 expression levels were correlated with cancer cell aggressiveness and IL-6 production by cancer cells was correlated with TG2 expression levels in ovarian cancer cells. The size and efficiency of tumor sphere formation were correlated with TG2 expression levels and were dependent on TG2-mediated IL-6 secretion in ovarian cancer cells. Using highly immunocompromised mice, we evaluated the critical role of TG2 and downstream IL-6 in distant hematogenous metastasis of ovarian cancer cells. Thus, in this report, we provide evidence that TG2 is an important link in IL-6-mediated tumor aggressiveness in vivo.
The human ovarian cancer cell lines MDAH-2774 (ATCC CRL-10303) and SK-OV-3 (ATCC HTB-77) were purchased from the American Type Culture Collection (Manassas, VA, USA) and cultured in RPMI 1640 (WelGENE, Daegu, South Korea) supplemented with 10% heat-inactivated fetal bovine serum (GIBCO, Grand Island, NY, USA) and 1% antibiotics (100 U/mL penicillin and 100 µg/mL streptomycin) at 37℃ in a humidified 5% CO2 atmosphere. TG2-knocked-down MDAH-2774 cells (shTG2_2774) and IL-6-knocked-down MDAH-2774 cells (shIL-6_2774) were established using the short hairpin RNA (shRNA) vector. The target sequence for TG2 was 5'-GGGCGAACCACCTGAACAA-3'. IL-6 shRNA vector (shIL-6) was kindly provided by Prof. Hyunggee Kim of Korea University, Korea. Briefly, MDAH-2774 cells were cotransfected with the pcDNA3.1 plus pSUPER plasmid containing shRNA for TG2 using Promofectin (PromoKine, Heidelberg, Germany), according to the manufacturer's instruction. Control cells were transfected with the pcDNA3.1 vector only. Stable transfected clones were established by selection with G418 (Sigma-Aldrich, St. Louis, MO, USA) at a concentration of 500 µg/mL for 3 weeks. TG2 knockdown in selected clones was shown by Western blot analysis. MDAH-2774 cells transfected with the shIL-6 vector were cultured in the presence of G418, and IL-6-knocked-down MDAH-2774 (shIL-6_2774) clones were selected by performing an IL-6 ELISA.
Cells were harvested in a lysis solution containing 50 mM Tris/HCl (pH 7.6), 1% NP40, 150 mM NaCl, 2 mM EDTA with 100 µM PMSF, a protease inhibitor cocktail (Roche Applied Science, Basel, Switzerland), and a phosphatase inhibitor (Sigma-Aldrich). After a 30-min incubation on ice, cellular debris was removed by centrifugation (10 min, 4℃). The protein concentration was measured by the BCA protein assay reagent (Thermo Scientific, Rockford, IL, USA). Proteins (10 µg) were separated by SDS-PAGE and then transferred to a polyvinylidene difluoride membrane. After blocking with 5% skim milk, the membranes were probed with an appropriate antibody. Blots were developed with an enhanced chemiluminescence Western-blotting detection system (Amersham, GE Healthcare, Buckinghamshire, UK). The antibodies used were as follows: anti-TG2 (Neomarkers, Fremont, CA, USA), anti-β-actin (Sigma- Aldrich), anti-Bcl-2 (Santa Cruz Biotechnology, Santa Cruz, CA, USA), anti-cIAP2 (Santa Cruz Biotechnology), anti-vimentin (DAKO, Glostrup, Denmark), and antifibronectin (Santa Cruz Biotechnology).
In total, 1×104 cells were plated on a 24-well plate and allowed to adhere overnight. Then, the medium was replaced, and cells were permitted to grow for 24, 48, or 72 h. To inhibit TG2 activity, some cells were treated with 0.1, 0.5, or 1.0 mM cysteamine (Sigma-Aldrich). Supernatants were collected and assayed to quantify soluble IL-6 production by ELISA. The MTT assay was performed to evaluate the effects of cysteamine on cell proliferation. After treatment, the cells were washed with PBS and incubated with MTT (0.5 mg/mL in RPMI) for 4 h at 37℃. The formazan crystals were solubilized in DMSO for 10 min, and the optical density was measured with an ELISA plate reader at 560 mm. All assays were performed in triplicate and were repeated twice or three times under independent conditions. Data are presented as means±SDs.
Cells grown in an anchorage-independent condition were trypsinized, washed twice with PBS, and resuspended in serum-free medium consisting of a 1:1 mixture of F-12 Nutrient Mixture (Ham's F-12) and DMEM, supplemented with 20 ng/mL epithelial growth factor (Invitrogen, Carlsbad, CA, USA), 20 ng/mL basic fibroblast growth factor (Millipore, Billerica, MA, USA), 0.5 nM hydrocortisone (Sigma-Aldrich), B-27 supplement (GIBCO), 2 mM L-glutamine (GIBCO), and 1% antibiotics (100 U/mL penicillin and 100 µg/mL streptomycin). Cells were plated on a non-adherent 96-well plate (Corning, Corning, NY, USA) at 10, 100, or 1000 cells/100 µL/well. To inhibit TG2 activity, some cells were treated with 0.1, 0.5, and 1.0 mM cysteamine (Sigma-Aldrich). In some wells, 10 µg/mL anti-IL-6 mAb (eBioscience, San Diego, CA, USA) or 10 ng/mL recombinant IL-6 (eBioscience) was added. The medium was replaced every 3 days. Visible spheres were counted under a microscope on day 8 post-plating.
NOD/scid/IL-2Rγ-/- (NOG) mice were obtained from the Jackson Laboratory (Bar Harbor, ME, USA) and were bred and maintained in specific pathogen-free conditions at the animal facility of Seoul National University College of Medicine. All animal experiments were performed with the approval of the Institutional Animal Care and Use Committee of Seoul National University.
To assess tumorigenicity of the cancer cell lines, the human ovarian cancer cell line MDAH-2774 (5×105 cells/each mouse), stably transfected with shTG2 or empty vector, was injected intraperitoneally into 8-week-old NOG mice. Six weeks after the injection, the mice were euthanized, peritoneal tumor masses, livers, and lungs were fixed in 4% paraformaldehyde for 24 h, and embedded in paraffin. Sections (4 µm) were stained with hematoxylin and eosin for histopathological analysis. The number of tumor masses in liver and lung were counted under a dissecting microscope.
Total RNA was isolated using RNeasy kit (QIAGEN). cDNA generated from 1 mg of total RNA by M-MLV (TAKARA) was analyzed by PCR. Following primer pairs were used for PCR. GAPDH; 5'-GTCAGTGGTGGACCTGACCT-3'/5'-AGGGGTCTACATGGCAACT G-3', MMP2; 5'-CACTTTCCTGGGCAACAAAT-3'/5'-GCCTCGTATACCGCATCAAT-3', MMP7; 5'-CAGATGTGGAGTGCCAGATG-3'/5'-TGTCAGCAGTTCCCCATACA-3', MMP9; 5'-GGCGCTCATGTACCCTATGT-3'/5'-TCAAAGACCGAGTCCAGCTT-3', MMP14; 5'-CAAGCATTGGGTGTTTGATG-3'/5'-CTTGGGGTACTCGCTATCCA-3', PCR products were analyzed in 2% agarose gel electrophoresis and subjected to densitometric analysis (Labworks 4.6, UVP BioImaging Systems) after ethidium bromide staining.
Intracellular TG2 activity was evaluated by measuring 5-(biotinamido) pentylamine (BP, Pierce) incorporation into cellular proteins. Freshly isolated cells from peritoneal mass or MDAH-2774 cells (1×105) were plated on a 12-well plate and incubated with 0.2 mM BP for 48 h. Cells were harvested in a lysis solution described above. TG2 activity was evaluated by SDS-PAGE probing with streptavidin-HRP followed by chemiluminescence detection.
MDAH-2774 cells (2×105) were seeded on a 12-well plate and allowed to adhere for overnight. Cells were incubated for 12 h in RPMI 1640 supplemented with 0.2% FBS. The cell monolayer was then scratched with a pipette tip and washed with PBS. The migration of cells into the wound area was induced by treatment of 10 ng/mL IL-6 for 20 h.
The IL-6 production and cell viability results are expressed as means±SEMs. A two-tailed Student's t-test was used to compare measurements taken from the two samples. For in vivo experiments, differences in the number of tumor masses and tumor volume were analyzed using a two-tailed Student's t-test. All P-values<0.05 were deemed to indicate statistical significance. The SPSS software (ver. 11.0; SPSS Inc., Chicago, IL, USA) was used for all statistical analyses.
To evaluate the correlation between TG2 expression and biological characteristics of cancer cells, we first analyzed TG2 expression in two human ovarian cancer cells. TG2 expression in ovarian cancer cells was correlated with in vivo aggressive behaviors in xenograft models: TG2-high-expressing MDAH-2774 cells showed more rapid tumor growth in vivo in immunocompromised mice than TG2-low-expressing SK-OV-3 cells (Figure 1A, our unpublished data). Next, we measured IL-6 production from ovarian cancer cell culture supernatants and found that cells expressing a high level of TG2 produced a large amount of IL-6 and those with low levels of TG2 secreted a minimal amount of IL-6 (Figure 1B).
To evaluate whether modulation of TG2 expression levels in the given cancer cells leads to a change in IL-6 production, we compared IL-6 levels in control empty vector-transfected TG2-high-expressing MDAH-2774 cells (cont_2774) and TG2-knocked-down MDAH-2774 cells using shRNA vectors (shTG2_2774#2 and shTG2_2774#3; Figure 1C). Both shTG2_2774#2 and shTG2_2774#3 showed reduced IL-6 production compared with cont_2774 (Figure 1D). A causal link between TG2 activity and IL-6 production was confirmed using the TG2 inhibitor cysteamine. Inhibition of TG2 enzymatic activity (Figure 1E), but not cysteamine cytotoxicity (Figure 1F), reduced IL-6 production from TG2-high-expressing cont_2774 cells. These findings indicate that TG2 is a key regulator of IL-6 production in ovarian cancer cells. We also established IL-6-knocked-down ovarian cancer cells (shIL-6_2774#11) using the shIL-6 vector. shIL-6_2774#11 showed reduced IL-6 secretion (Figure 1G), but revealed TG2 expression levels comparable to that of control cells (Figure 1G). Thus, IL-6 production occurred downstream of TG2 activity in ovarian cancer cells.
TG2 expression endows aggressiveness and invasiveness to cancer cells through multiple effector pathways [29]. As tumor cell behavior favoring invasion and metastasis has been attributed to their predisposition to contactindependent growth and tumor sphere formation [30], along with the fact that tumor subpopulations that form tumor spheres show enhanced invasive characteristics in vitro and advanced primary tumors from patients show an increased potential to form tumor spheres [2531], we evaluated whether TG2 and downstream IL-6 were involved in tumor sphere formation in vitro. Sphere formation decreased markedly in both TG2-knocked-down and IL-6-knocked-down ovarian cancer cells (shTG2_2774#3 and shIL-6_2774#11, respectively) compared with the control empty vector-transfected cells (Figure 2A). The numbers and sizes of the spheres decreased in both TG2-knocked-down and IL-6-knocked-down ovarian cancer cells (Figure 2A and B). Tumor sphere formation in control cancer cells (cont_2774) was reduced by both treatment with cysteamine and anti-IL-6 blocking antibody (Figure 2C and D), and adding recombinant IL-6 increased the number and size of shTG2_2774#3 spheres (IL-6-low-expressing; Figure 2E). Control cancer cells (cont_2774) cultured in sphere media expressed more anti-apoptotic molecules, such as cIAP2 and Bcl-2, than TG2-knocked-down cells (shTG2_2774#3) (Figure 2F). These data indicate that TG2 supported cell survival under an anchorageindependent condition.
Previous results have demonstrated that TG2 expression in ovarian cancer cells regulated IL-6 production, resulting in tumor sphere formation. To evaluate the role of TG2 and downstream IL-6 in tumor cell behavior in vitro, the effect of TG2 and its downstream IL-6 on in vivo tumor spreading and distant metastasis was evaluated using highly immunocompromised NOD/scid/IL-2Rγ chain-deficient (NOG) mice, which completely lack NK activity as well as T and B cell activity, as recipients [32].
Ovarian cancer cells with high TG2 levels (cont_2774) showed extensive peritoneal tumor spreading 6 weeks after an intraperitoneal inoculation of tumor cells compared to TG2-knocked-down cells (shTG2_2774#3) and IL-6-knocked-down cells (shIL-6_2774#11) (Figure 3A, C, and D). Additionally, NOG mice intraperitoneally injected with cont_2774 ovarian cancer cells showed distant hematogenous metastases, revealing multiple visible tumor masses in the lung and liver parenchyma (Figure 3A-C). Metastatic tumor cells in these organs were removed from the surface and were present around the vessels, indicating that these tumors were not simply surface spreading through the mesothelial lining of the abdominal and thoracic cavities. In contrast, mice inoculated with shTG2_2774#3 revealed no distant metastasis in 14 mice tested and mice injected with shIL-6_2774#11 showed few, if any, distant lung or liver metastases. There were fewer than three visible metastases in five mice and the remaining seven mice showed no visible metastases in the liver; there were fewer than six visible metastases in six mice, and the remaining six mice showed no visible metastasis in the lung (Figure 3C).
Next, we evaluated the underlying signaling differences enabling increased tumor growth, peritoneal spreading, and distant metastasis of TG2- and IL-6-high-expressing ovarian cancer cells. As the tumor progressed in the peritoneal space, increased TG2 expression levels (Figure 4A) and more dissociated spindle-shaped cells (Figure 4B) were evident in the cells cultured from the peritoneal tumor mass compared with the original control ovarian cancer cells (cont_2774). Increased vimentin expression, an EMT-related marker, and increased crosslinked products of fibronectin were prominent in the peritoneal mass of control ovarian cancer cells but not in that of TG2-knocked-down ovarian cancer cells (Figure 4C). These findings suggest that TG2 expression contributed to the EMT of ovarian cancer cells in the peritoneal space. Moreover, control ovarian cancer cells revealed higher expression of several matrix metalloproteinases (MMPs), a group of metal-dependent enzymes that are involved in extracellular matrix remodeling and are critical for tumor cell migration and invasion, particularly MMP-2, MMP-7, MMP-9, and MMP-14 as compared to TG2-knocked-down and IL-6-knocked-down ovarian cancer cells (Figure 4D). As the role of TG2 in in vitro migration and invasion of tumor cells was reported as enhanced cell attachment via beta-integrins and fibronectins with extracellular TG2 [131533] and increased MMP-2 expression [34], we evaluated whether downstream IL-6 also affects ovarian cancer cell migration. IL-6 increased the migration of ovarian cancer cells in an in vitro migration assay (Figure 4E).
Metastasis is one of the most serious and relevant problems in cancer treatment. Cancer metastasis is a complex process that requires the action of various effectors not yet completely identified. In this study, we constructed a human ovarian cancer distant metastasis model in highly immunocompromised mice, which completely lack T, B, and NK activity [32]. We found that TG2 expression and downstream IL-6 in cancer cells were essential for tumor growth, peritoneal spreading, and distant hematogenous metastasis of human ovarian cancer.
Tumor sphere formation in vitro reflects their contact-independent survival and is thus related to the anoikis-resistance [253035]. For distant hematogenous or lymphogenous metastasis, detached cells from the cancer mass enter the circulation. The survival of circulating cancer cells is affected by inflammatory cytokines, such as tumor necrosis factor alpha (TNF-α) and IL-6 [36]. TNF-a has controversial effects in cancer pathogenesis, including induction of apoptosis or the EMT of cancer cells [3738]. IL-6 is produced from epithelial tumor cells themselves as well as infiltrating inflammatory cells, and cancer-derived IL-6 induces a cancer stem cell-like phenotype in these cells [25]. In addition to this, sustained activation of STAT3, a downstream IL-6 signaling mediator, is important for untransformed stem cell self-renewal [3940].
In the present study, in vitro tumor sphere formation of ovarian cancer cells was largely dependent on TG2 expression and inhibited by a TG2 enzymatic inhibitor. IL-6 production from ovarian cancer cells was dependent on TG2 expression, and tumor sphere formation was correlated with TG2-dependent IL-6 production in ovarian cancer cells, indicating that the effect of TG2 on tumor sphere formation is mediated through downstream IL-6 production. A correlation between in vitro tumor sphere formation and in vivo tumorigenesis was confirmed in xenograft models of ovarian cancer cells, and TG2 expression and downstream IL-6 production were profoundly correlated with primary tumor growth, peritoneal spreading, and distant hematogenous lung and liver metastasis of human ovarian cancer cells.
Tissue invasion and distant metastasis of epithelial cancer cells have been critically related to their phenotypic change through the EMT [41]. Tumor cells undergoing EMT expressed mesenchymal tissue-specific markers such as vimentin and showed characteristic myofibroblast-like properties with a spindle-shaped appearance and increased fibronectin polymerization [41]. These events in human ovarian cancer cells were dependent on their TG2 expression in the current study (Figure 4), and recent reports have revealed important roles of TG2 during the EMT process of ovarian and breast cancer cells [4243]. The EMT of epithelial cancer cells has been clearly linked to the EMT of epithelial cells during fibrosis. In our hands, TG2 is also an important mediator of fibrosis downstream of TGF-β [27]. Thus, TG2 (and downstream IL-6) may play a critical role in the EMT process of both normal and malignant epithelial cells, leading to organ fibrosis and cancer invasion and metastasis.
As most ovarian cancer originates from surface epithelial cells, the primary spreading of ovarian cancer cells is by a peritoneal route [9]. NOG mice injected intraperitoneally with control MDAH-2774 cells revealed visible multiple metastatic nodules in the lung and liver, while none of the mice injected with TG2-knocked-down cells showed visible metastases. Mice injected with IL-6-knocked-down cells displayed decreased distant metastasis to the lung and liver. Thus, TG2 and downstream IL-6 produced from epithelial ovarian cancer cells are important factors for distant hematogenous lung and liver metastases of these cells.
In the present study, the TG2 and downstream IL-6 signaling pathway in human ovarian cancer cells may have acted as a convergence site for cancer stem cell and EMT phenotypes, leading to enhanced tumor growth, peritoneal spreading, and distant hematogenous lung and liver metastasis. Thus, targeting either TG2 or downstream IL-6 would be a very efficient strategy to inhibit primary tumor growth, peritoneal spreading, and distant hematogenous metastasis of ovarian cancer cells. More profound reduction of peritoneal tumor spreading and distant lung and liver metastases by TG2-knockdown compared with IL-6-knockdown in ovarian cancer cells might simply be a result of the more complete knockdown of TG2 in ovarian cancer cells in our experiments. In addition to the IL-6 signaling pathway, TG2 can directly modulate the ECM structure [1329333444], activate focal adhesion kinase via binding with integrins [13], and activate growth factors such as TGF-β [45], a key inducer of EMT, buried in the ECM networks. Thus, this may affect a broader aspect of tumor biology, which may also explain the differences between TG2-knockdown and IL-6 knockdown of ovarian cancer cells.
The clinical significance of TG2 expression in ovarian cancer has been reported; TG2 is upregulated in cancer tissue compared to its normal counterpart [1146], and TG2 overexpression is associated with high tumor stage [10]. As increased TG2 expression has also been reported in metastatic tumor tissue, we propose that TG2 is an important mediator and/or prognostic marker for distant metastasis, affecting both cancer stem cell-like and EMT phenotypes. Thus, intervention specific to TG2 and/or downstream IL-6 in epithelial ovarian cancer cells could provide a promising means to control tumor metastasis.
Acknowledgments
This work was supported by the Seoul National University Hospital Research Fund (03-2014-0050 to D.S.L., 2014-1294) and Education and Research Encouragement Fund of Seoul National University Hospital.
References
1. Lorand L, Graham RM. Transglutaminases: crosslinking enzymes with pleiotropic functions. Nat Rev Mol Cell Biol. 2003; 4(2):140–156. PMID: 12563291.


2. Fesus L, Piacentini M. Transglutaminase 2: an enigmatic enzyme with diverse functions. Trends Biochem Sci. 2002; 27(10):534–539. PMID: 12368090.


3. Mehta K. Biological and therapeutic significance of tissue transglutaminase in pancreatic cancer. Amino Acids. 2009; 36(4):709–716. PMID: 18594944.


4. Mehta K, Fok J, Miller FR, Koul D, Sahin AA. Prognostic significance of tissue transglutaminase in drug resistant and metastatic breast cancer. Clin Cancer Res. 2004; 10(23):8068–8076. PMID: 15585642.


5. Verma A, Mehta K. Tissue transglutaminase-mediated chemoresistance in cancer cells. Drug Resist Updat. 2007; 10(4-5):144–151. PMID: 17662645.


6. Verma A, Wang H, Manavathi B, Fok JY, Mann AP, Kumar R, Mehta K. Increased expression of tissue transglutaminase in pancreatic ductal adenocarcinoma and its implications in drug resistance and metastasis. Cancer Res. 2006; 66(21):10525–10533. PMID: 17079475.


7. Antonyak MA, Miller AM, Jansen JM, Boehm JE, Balkman CE, Wakshlag JJ, Page RL, Cerione RA. Augmentation of tissue transglutaminase expression and activation by epidermal growth factor inhibit doxorubicin-induced apoptosis in human breast cancer cells. J Biol Chem. 2004; 279(40):41461–41467. PMID: 15272014.


8. Ai L, Kim WJ, Demircan B, Dyer LM, Bray KJ, Skehan RR, Massoll NA, Brown KD. The transglutaminase 2 gene (TGM2), a potential molecular marker for chemotherapeutic drug sensitivity, is epigenetically silenced in breast cancer. Carcinogenesis. 2008; 29(3):510–518. PMID: 18174247.
9. Chi DS, Sabbatini P. Advanced ovarian cancer. Curr Treat Options Oncol. 2000; 1(2):139–146. PMID: 12057051.


10. Hwang JY, Mangala LS, Fok JY, Lin YG, Merritt WM, Spannuth WA, Nick AM, Fiterman DJ, Vivas-Mejia PE, Deavers MT, Coleman RL, Lopez-Berestein G, Mehta K, Sood AK. Clinical and biological significance of tissue transglutaminase in ovarian carcinoma. Cancer Res. 2008; 68(14):5849–5858. PMID: 18632639.


11. Satpathy M, Cao L, Pincheira R, Emerson R, Bigsby R, Nakshatri H, Matei D. Enhanced peritoneal ovarian tumor dissemination by tissue transglutaminase. Cancer Res. 2007; 67(15):7194–7202. PMID: 17671187.


12. Cao L, Petrusca DN, Satpathy M, Nakshatri H, Petrache I, Matei D. Tissue transglutaminase protects epithelial ovarian cancer cells from cisplatin-induced apoptosis by promoting cell survival signaling. Carcinogenesis. 2008; 29(10):1893–1900. PMID: 18667446.


13. Mangala LS, Fok JY, Zorrilla-Calancha IR, Verma A, Mehta K. Tissue transglutaminase expression promotes cell attachment, invasion and survival in breast cancer cells. Oncogene. 2007; 26(17):2459–2470. PMID: 17043648.
14. Verma A, Guha S, Wang H, Fok JY, Koul D, Abbruzzese J, Mehta K. Tissue transglutaminase regulates focal adhesion kinase/AKT activation by modulating PTEN expression in pancreatic cancer cells. Clin Cancer Res. 2008; 14(7):1997–2005. PMID: 18381937.


15. Antonyak MA, Li B, Regan AD, Feng Q, Dusaban SS, Cerione RA. Tissue transglutaminase is an essential participant in the epidermal growth factor-stimulated signaling pathway leading to cancer cell migration and invasion. J Biol Chem. 2009; 284(27):17914–17925. PMID: 19403524.


16. Kim DS, Park SS, Nam BH, Kim IH, Kim SY. Reversal of drug resistance in breast cancer cells by transglutaminase 2 inhibition and nuclear factor-kappaB inactivation. Cancer Res. 2006; 66(22):10936–10943. PMID: 17108131.
17. Mann AP, Verma A, Sethi G, Manavathi B, Wang H, Fok JY, Kunnumakkara AB, Kumar R, Aggarwal BB, Mehta K. Overexpression of tissue transglutaminase leads to constitutive activation of nuclear factor-kappaB in cancer cells: delineation of a novel pathway. Cancer Res. 2006; 66(17):8788–8795. PMID: 16951195.
18. Jang GY, Jeon JH, Cho SY, Shin DM, Kim CW, Jeong EM, Bae HC, Kim TW, Lee SH, Choi Y, Lee DS, Park SC, Kim IG. Transglutaminase 2 suppresses apoptosis by modulating caspase 3 and NF-kappaB activity in hypoxic tumor cells. Oncogene. 2010; 29(3):356–367. PMID: 19838207.
19. Lee J, Kim YS, Choi DH, Bang MS, Han TR, Joh TH, Kim SY. Transglutaminase 2 induces nuclear factor-kappaB activation via a novel pathway in BV-2 microglia. J Biol Chem. 2004; 279(51):53725–53735. PMID: 15471861.
20. Knüpfer H, Preiss R. Significance of interleukin-6 (IL-6) in breast cancer (review). Breast Cancer Res Treat. 2007; 102(2):129–135. PMID: 16927176.


21. Bachelot T, Ray-Coquard I, Menetrier-Caux C, Rastkha M, Duc A, Blay JY. Prognostic value of serum levels of interleukin 6 and of serum and plasma levels of vascular endothelial growth factor in hormone-refractory metastatic breast cancer patients. Br J Cancer. 2003; 88(11):1721–1726. PMID: 12771987.


22. Grivennikov S, Karin E, Terzic J, Mucida D, Yu GY, Vallabhapurapu S, Scheller J, Rose-John S, Cheroutre H, Eckmann L, Karin M. IL-6 and Stat3 are required for survival of intestinal epithelial cells and development of colitis-associated cancer. Cancer Cell. 2009; 15(2):103–113. PMID: 19185845.
23. Huber MA, Azoitei N, Baumann B, Grünert S, Sommer A, Pehamberger H, Kraut N, Beug H, Wirth T. NF-kappaB is essential for epithelial-mesenchymal transition and metastasis in a model of breast cancer progression. J Clin Invest. 2004; 114(4):569–581. PMID: 15314694.
24. Sullivan NJ, Sasser AK, Axel AE, Vesuna F, Raman V, Ramirez N, Oberyszyn TM, Hall BM. Interleukin-6 induces an epithelial-mesenchymal transition phenotype in human breast cancer cells. Oncogene. 2009; 28(33):2940–2947. PMID: 19581928.


25. Sansone P, Storci G, Tavolari S, Guarnieri T, Giovannini C, Taffurelli M, Ceccarelli C, Santini D, Paterini P, Marcu KB, Chieco P, Bonafè M. IL-6 triggers malignant features in mammospheres from human ductal breast carcinoma and normal mammary gland. J Clin Invest. 2007; 117(17):3988–4002. PMID: 18060036.


26. Pardal R, Clarke MF, Morrison SJ. Applying the principles of stem-cell biology to cancer. Nat Rev Cancer. 2003; 3(12):895–902. PMID: 14737120.


27. Oh K, Park HB, Byoun OJ, Shin DM, Jeong EM, Kim YW, Kim YS, Melino G, Kim IG, Lee DS. Epithelial transglutaminase 2 is needed for T cell interleukin-17 production and subsequent pulmonary inflammation and fibrosis in bleomycin-treated mice. J Exp Med. 2011; 208(8):1707–1719. PMID: 21746810.


28. Schäfer M, Werner S. Cancer as an overhealing wound: an old hypothesis revisited. Nat Rev Mol Cell Biol. 2008; 9(8):628–638. PMID: 18628784.


29. Collighan RJ, Griffin M. Transglutaminase 2 cross-linking of matrix proteins: biological significance and medical applications. Amino Acids. 2009; 36(4):659–670. PMID: 18982407.


30. Mani SA, Guo W, Liao MJ, Eaton EN, Ayyanan A, Zhou AY, Brooks M, Reinhard F, Zhang CC, Shipitsin M, Campbell LL, Polyak K, Brisken C, Yang J, Weinberg RA. The epithelial-mesenchymal transition generates cells with properties of stem cells. Cell. 2008; 133(4):704–715. PMID: 18485877.


31. Sodek KL, Ringuette MJ, Brown TJ. Compact spheroid formation by ovarian cancer cells is associated with contractile behavior and an invasive phenotype. Int J Cancer. 2009; 124(9):2060–2070. PMID: 19132753.


32. Ito M, Hiramatsu H, Kobayashi K, Suzue K, Kawahata M, Hioki K, Ueyama Y, Koyanagi Y, Sugamura K, Tsuji K, Heike T, Nakahata T. NOD/SCID/gamma(c)(null) mouse: an excellent recipient mouse model for engraftment of human cells. Blood. 2002; 100(9):3175–3182. PMID: 12384415.
33. Verderio EA, Telci D, Okoye A, Melino G, Griffin M. A novel RGD-independent cel adhesion pathway mediated by fibronectin-bound tissue transglutaminase rescues cells from anoikis. J Biol Chem. 2003; 278(43):42604–42614. PMID: 12732629.
34. Satpathy M, Shao M, Emerson R, Donner DB, Matei D. Tissue transglutaminase regulates matrix metalloproteinase-2 in ovarian cancer by modulating cAMP-response element-binding protein activity. J Biol Chem. 2009; 284(23):15390–15399. PMID: 19324884.


35. Chiarugi P, Giannoni E. Anoikis: a necessary death program for anchorage-dependent cells. Biochem Pharmacol. 2008; 76(11):1352–1364. PMID: 18708031.


36. Kim MY, Oskarsson T, Acharyya S, Nguyen DX, Zhang XH, Norton L, Massagué J. Tumor self-seeding by circulating cancer cells. Cell. 2009; 139(7):1315–1326. PMID: 20064377.


37. Daniel D, Wilson NS. Tumor necrosis factor: renaissance as a cancer therapeutic? Curr Cancer Drug Targets. 2008; 8(2):124–131. PMID: 18336195.


38. Takahashi E, Nagano O, Ishimoto T, Yae T, Suzuki Y, Shinoda T, Nakamura S, Niwa S, Ikeda S, Koga H, Tanihara H, Saya H. Tumor necrosis factor-alpha regulates transforming growth factor-beta-dependent epithelial-mesenchymal transition by promoting hyaluronan-CD44-moesin interaction. J Biol Chem. 2010; 285(6):4060–4073. PMID: 19965872.
39. Chung YJ, Park BB, Kang YJ, Kim TM, Eaves CJ, Oh IH. Unique effects of Stat3 on the early phase of hematopoietic stem cell regeneration. Blood. 2006; 108(4):1208–1215. PMID: 16614239.


40. Müller S, Chakrapani BP, Schwegler H, Hofmann HD, Kirsch M. Neurogenesis in the dentate gyrus depends on ciliary neurotrophic factor and signal transducer and activator of transcription 3 signaling. Stem Cells. 2009; 27(2):431–441. PMID: 19023034.


41. Thiery JP, Acloque H, Huang RY, Nieto MA. Epithelial-mesenchymal transitions in development and disease. Cell. 2009; 139(5):871–890. PMID: 19945376.


42. Shao M, Cao L, Shen C, Satpathy M, Chelladurai B, Bigsby RM, Nakshatri H, Matei D. Epithelial-to-mesenchymal transition and ovarian tumor progression induced by tissue transglutaminase. Cancer Res. 2009; 69(24):9192–9201. PMID: 19951993.


43. Kumar A, Xu J, Brady S, Gao H, Yu D, Reuben J, Mehta K. Tissue transglutaminase promotes drug resistance and invasion by inducing mesenchymal transition in mammary epithelial cells. PLoS One. 2010; 5(10):e13390. PMID: 20967228.


44. Yuan L, Siegel M, Choi K, Khosla C, Miller CR, Jackson EN, Piwnica-Worms D, Rich KM. Transglutaminase 2 inhibitor, KCC009, disrupts fibronectin assembly in the extracellular matrix and sensitizes orthotopic glioblastomas to chemotherapy. Oncogene. 2007; 26(18):2563–2573. PMID: 17099729.


45. Kojima S, Nara K, Rifkin DB. Requirement for transglutaminase in the activation of latent transforming growth factor-beta in bovine endothelial cells. J Cell Biol. 1993; 121(2):439–448. PMID: 8096847.


46. Singer CF, Hudelist G, Walter I, Rueckliniger E, Czerwenka K, Kubista E, Huber AV. Tissue array-based expression of transglutaminase-2 in human breast and ovarian cancer. Clin Exp Metastasis. 2006; 23(1):33–39. PMID: 16826431.


Figure 1
TG2 expression levels in cancer cells correlated with IL-6 production. (A) TG2 expression in the two human ovarian cancer cell lines was analyzed by Western blotting. (B) IL-6 levels in culture supernatants of ovarian cancer cells were determined by enzyme-linked immunosorbent assay (ELISA). Cells (1×104) were cultured in a 24-well tissue culture plate, and the supernatants were collected 24, 48, and 72 h after culture. (C) MDAH-2774 human ovarian cancer cells (cont_2774, control empty-vector transfected; shTG2_2774, TG2-knocked-down) were stably transfected with short hairpin RNAs (shRNAs) targeting TG2. The efficiency of knockdown was assessed by Western blotting. (D) IL-6 levels in culture supernatants of control and TG2-knocked-down MDAH-2774 cells were determined by ELISA. Cells (1×104) were cultured in a 24-well tissue culture plate, and the supernatants were collected 24 and 48 h after culture. (E, F) The effect of the TG2 inhibitor cyteamine (CyM) on IL-6 secretion (E) and cell viability (F) in control MDAH-2774 cells was determined by ELISA and the MTT assay, respectively. (G) MDAH-2774 cells were stably transfected with shRNAs targeting IL-6. The efficiency of knockdown was verified by measuring IL-6 levels in the culture supernatants. TG2 expression of control and IL-6-knocked-down MDAH-2774 cells was analyzed by Western blotting. Data represent the mean±standard deviation (SD), based on three independent experiments using samples from triplicate cell cultures.
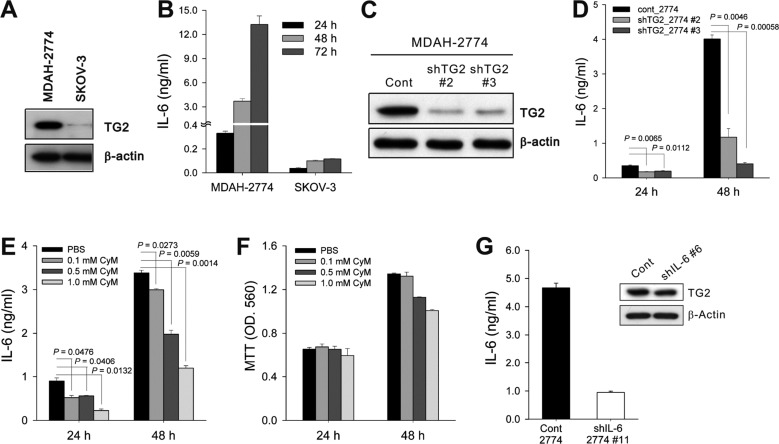
Figure 2
TG2-mediated IL-6 allows anchorage-independent survival in cancer cells. (A) Control (cont_2774) and TG2-knocked-down (shTG2_2774) MDAH-2774 cells were cultured in serum-free medium consisting of a 1:1 mixture of Ham's F-12 and DMEM, supplemented with epidermal growth factor, basic fibroblast growth factor, hydrocortisone, B-27 supplement, L-glutamine, and antibiotics. Visible spheres were counted under a microscope on day 8 post-plating. (B) Phase-contrast microscopy of day 8 spheres generated from the MDAH-2774 cell line. (C) Effect of the TG2 inhibitor cyteamine (CyM) on sphere formation. (D, E) Spheres from control (D) and TG2-knocked-down (shTG2_2774) (E) MDAH-2774 cells were generated in the presence of anti-IL-6 blocking antibody (10 mg/mL) or recombinant IL-6 (10 ng/mL), respectively. Visible spheres were counted under a microscope on day 8 post-plating. (F) cIAP2 and Bcl-2 expression was analyzed by Western blotting after culture in sphere medium. Data represent the mean±SD based on three independent experiments using samples from triplicate cell cultures.
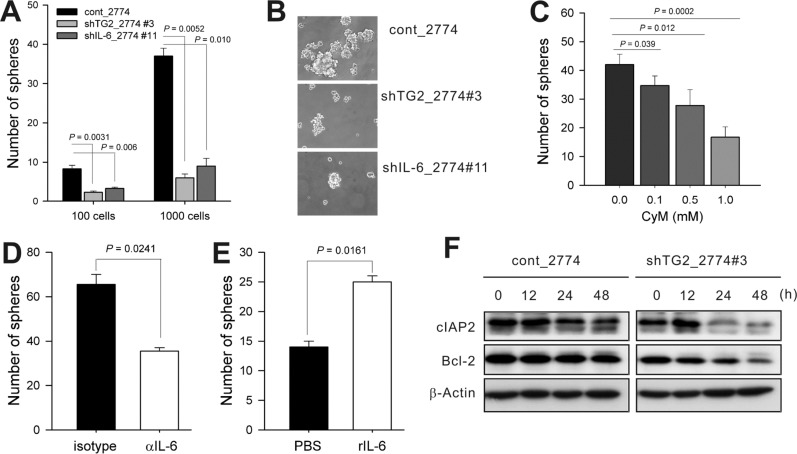
Figure 3
TG2-knocked-down and IL-6-knocked-down ovarian cancer cells revealed reduced tumor growth and distant metastasis. (A-D) NOG mice were injected intraperitoneally with control, TG2-knocked-down, or IL-6-knocked-down MDAH-2774 cells (5×105 cells/each mouse). At 6 weeks after inoculation, the mice were killed and the primary tumor masses in the abdominal cavity and distant metastases in the liver, lung, and other organs were analyzed. (A) Tumor formation in the abdominal cavities, livers, and lungs of mice xenografted with MDAH-2774 cells. (B) Lung sections from mice xenografted with MDAH-2774 cells were obtained and stained with hematoxylin and eosin. (A, B) Data shown are representative of each group. (C) Number of masses in the mesentery, liver, and lung from mice xenografted with MDAH-2774. (D) Comparison of tumor size developed in the mesentery of mice xenografted with MDAH-2774. (C, D) Data are presented as the mean±SD) of 12-14 mice for each group from two independent experiments.
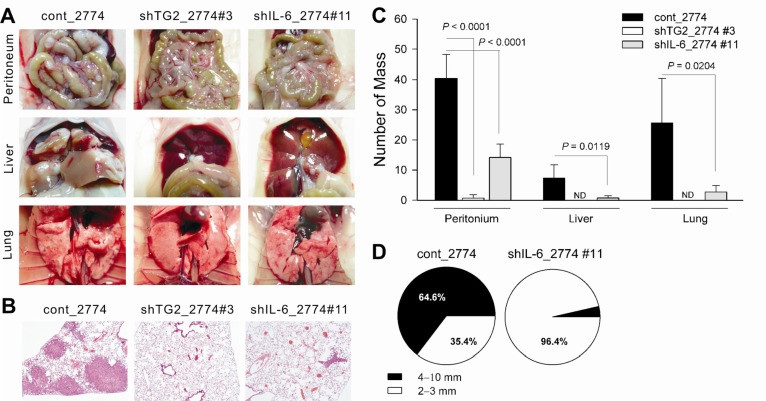
Figure 4
TG2-knocked-down or IL-6-knocked-down ovarian cancer cells revealed reduced epithelial-to-mesenchymal transition and in vitro migration. (A) Intracellular activity and expression of TG2 in the lung tissues from MDAH-2774 cells and cultivated peritoneal mass. Enzyme activity was estimated by biotinylated pentylamine (BP) incorporation probed with HRP-conjugated streptavidin (SA) in Western blot analysis. (B) Morphology of MDAH-2774 cells and cultivated peritoneal mass. Peritoneal masses from MDAH-2774-bearing mice were removed and digested with collagenase D and DNase I. Cells were suspended in 30% Percoll, layered onto the top of a 70% Percoll gradient, and centrifuged. The top layer, an enriched population of epithelial and fibroblast cells, was retained. Human cells were selected using MACS separation with antihuman MHC class I antibody (anti-HLAI). (C) Expression of TG2, fibronectin, and vimentin in MDAH-2774 cells and peritoneal mass was determined by Western blot analysis. (D) Expression of matrix metalloproteinases in MDAH-2774 cells and peritoneal mass were determined by reverse transcription-polymerase chain reaction. (E) Migration of MDAH-2774 cells into the wound areas created by scratching was induced by IL-6 (10 ng/mL) for 20 h. These results represent one of three independent experiments.
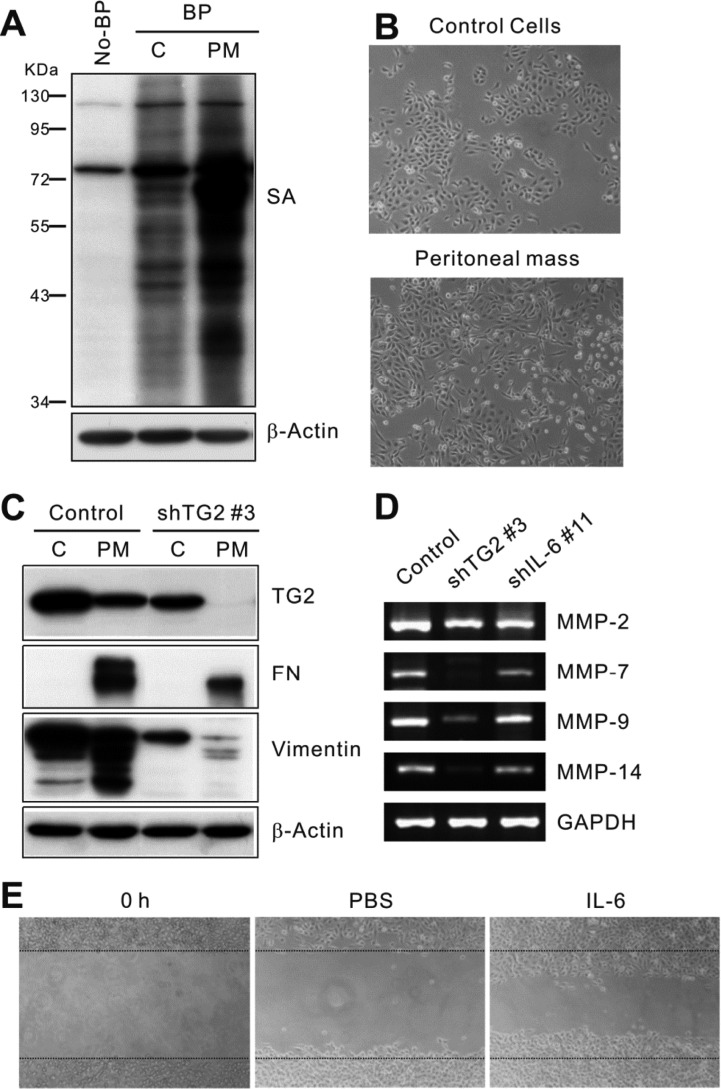