Abstract
Some polymers and bioactive compounds derived from Styela clava tunic (SCT) have been reported as traditional medicine for the treatment of inflammation, oxidative stress and surgical wounds although there is little scientific evidence of their liver and kidney toxicity. To investigate the toxicity of ethanol extracts of SCT (EtSCT) in the liver and kidney of ICR mice, alterations in related markers including body weight, organ weight, urine composition, liver pathology and kidney pathology were analyzed following oral administration of 50 and 100 mg/kg body weight/day of EtSCT for 14 days. EtSCT showed a high level of free radical scavenging activity for DPPH (93.1%) and NO (16.2%) as well as the presence of 14.8 mg/mL of flavonoids and 36.2 mg/mL of phenolics, while EtSCT treated groups did not show any significant alterations in the body and organ weight, clinical phenotypes, urine parameters or mice mortality when compared with the vehicle treated group. In addition, constant levels of serum biochemical markers including alanine phosphatase (ALP), alanine aminotransferase (ALT), aspartate aminotransferase (AST), blood urea nitrogen (BUN) and serum creatinine (CRE) were maintained. Moreover, no specific histopathological features induced by most toxic compounds were observed in liver and kidney sections stained with hematoxilin and eosin. Therefore, the present results indicate that EtSCT with strong antioxidant activity cannot induce any specific toxicity in liver and kidney organs of ICR at doses of 100 mg/kg body weight/day.
Styela clava (SC) is a solitary tunicate that has been widely applied for sea food, food additives and food base in Korea [1]. SCT has being investigated in only two major materials including natural polymers and bioactive compounds with to treat inflammation, oxidative stress and surgical wounds [23].
Firstly, cellulose, one of the natural polymers, was collected from SCT using various solvents including N-methylmorpholine-N-oxide (NMMO) and [Amim]Cl ionic liquid [45]. They showed therapeutic effects on surgical wounds in the skin and bone defects in the cervical bone cervical bone of animals without significant toxicity. Cellulose films (CF) were successfully prepared from solutions of SCT cellulose powder completely dissolved in NMMO/H2O (87/13 wt%) and [Amim]Cl ionic liquid [5]. These CFs were implanted in SD rats for various lengths of time from 0 to 90 days, during which no significant alterations in the epidermal hyperplasia, inflammatory cell infiltration, redness, or edema were observed [3]. Furthermore, the hydrocolloid membrane containing SCT powder (HCM-SCT) induced significant reepithelization, decreased epidermis thickness, shorter wound diameter and increased collagen in surgical wounds of SD rats [6]. Moreover, the bioinductive effects of cellulose membrane (CM) obtained from Ascidians squirt skin was detected in bone and mesenchymal tissues in the periosteum of cervical bone defects of SD rats [7]. In addition, glycosaminoglycans that contained 35.4% protein and 18.5% sulfate, which can serve as a lubricant or a shock absorber, were successfully extracted from SCT using sodium phosphate at 105℃ for 2 h followed by deproteinization with trichloroacetic acid or hydrochloride [8].
Several bioactive compounds and extracts collected from SCT by extraction with different solvents have also been found to have biological activity in cells and animals. TNF-α-induced NF-κB activation and the expression of two inflammatory factors (VCAM-1 and iNOS) were effectively suppressed by chondroitin sulfate extracted from SCT in JB6 P+ cells derived from BALB/c mice [9]. Moreover, carotenoids detected in high levels in SCT showed strong hydroxyl radical scavenging activities, reducing power and inhibitory effects against linoleic acid peroxidation [10]. A high level of tyrosinase inhibition and antioxidant activity were also induced by nine extracts collected from SCT using different solvents because they have high total phenolic and flavonoid contents [2]. However, the toxicity of SCT toward liver and kidney organs of ICR mice has not been investigated to date, despite the increased attention and publications regarding the therapeutic effects of EtSCT as a natural polymer and bioactive compound.
Therefore, in this study, toxicity was investigated in the livers and kidneys of ICR mice treated with 50 mg/kg and 100 mg/kg EtSCT for 14 days. The results of the present study provide basic information for determining the optimal concentration of EtSCT as a therapeutic drug for the treatment of human chronic diseases such as inflammation, surgical wounds and oxidative stress.
SCT powder was prepared as previously described [35]. Briefly, 330 g of SCT in 10% NaOH aqueous solution (9,900 mL) were boiled at 100℃ for 2 h to remove sediments and debris after collection from the beach of the South Sea in Gosung-gun, Korea (Figure 1Aa). SCTs were then washed with distilled water three times, after which they were boiled in 5% CH3COOH solution at 100℃ for 2 h to neutralize the NaOH solution. Following washing with distilled water three times, these samples were bleached by separate boiling and washing in 10% H2O2 solution (Figure 1Ab). The washed SCT samples were then dried at 100-120℃ for 2-3 hrs, after which they were ground in a pin mill machine (Daehwa, Korea) using a proprietary commercial process in which they were passed through a combination of 30 mesh sieves for 10 min once, then 120 mesh sieves for 10 min twice (Figure 1Ac). The particle size of SCT powders was measured using a Bettersize 2000 Laser Particle Size Analyzer (Bettersize Instruments Ltd., China) with a wide detection range (0.02-2,000 m), high ultrasonic power (50W), wide circulating flow rate (500-2,500 mL/min) and large photo detectors (90 ea). Most particles of SCT powder were distributed in the range of 6.34-306.2 µm, with particles 12.4-268.2 µm in diameter accounting for 80% of the total (Figure 1Ad).
EtSCT was prepared as previously described [2]. Briefly, ethanol extracts were purified from 100 g of SCT powder for 3 h at 80℃ using circulating extraction equipment (IKA Labortechnik, Staufen, Germany) following adding 1,000 mL of 100% ethanol. After repeating this process three times, a solution of the extracts was concentrated to dry pellets in a rotary evaporator (EYELA, Tokyo, Japan) after filtration through filter paper (Whatman No. 1, 100-125, Whatman International Ltd., Maidstone, England). Samples were then stored at -80℃ until further use.
Total phenolic contents were measured by the Folin-Ciocalteu method, with slight modification [11]. Briefly, 1 mL of EtSCT solution was mixed with 5 mL of Folin-Ciocalteu reagent (Sigma-Aldrich Co., St. Louis, MO, USA), then incubated at room temperature for 5 min. The mixture was subsequently added to 15 mL of 20% Na2CO3 and vortexed for 30 sec, after which the absorbance was repeatedly measured at 765 nm using a Versa-max plate reader (Molecular Devices, Sunnyvale, CA, USA). A standard calibration curve was made using different concentrations of gallic acid (Sigma-Aldrich Co.), and the concentration of total phenolic contents in EtSCT was presented as mg gallic acid equivalent of extract.
The flavonoid contents were measured as previously described [12]. Briefly, 200 µL of several different concentrations of EtSCT were mixed with 60 µL of 5% NaNO2 (Sigma-Aldrich Co.) and 60 µL of 10% AlCl3 (Sigma-Aldrich Co.). Following incubation at 25℃ for 5 min, the mixture was added to 400 µL of 1M NaOH and the absorbance was repeatedly measured at 510 nm using a Versa-max plate reader (Molecular Devices). A standard calibration curve was then made using different concentrations of catechin (Sigma-Aldrich Co.). The concentration of flavonoid contents in EE-SCT was presented as mg catechin equivalent of extract.
The scavenging activity of 2,2-diphenyl-1-picrylhydrazyl (DPPH) radicals was measured as previously described [13]. Briefly, each sample (250 µL) of EtSCT was mixed with 500 µL of 0.2 mM DPPH (Sigma-Aldrich Co.) in 95% ethanol solution or 100 µL of 95% ethanol solution, then incubated for 30 min at room temperature. The absorbance of the reaction mixture was subsequently measured at 517 nm using a Versa-max plate reader (Molecular Devices). The DPPH radical scavenging activity of the EtSCT was expressed as the percent decrease in absorbance relative to the control.
The scavenging activity of nitric oxide (NO) was measured as previously described [14]. Briefly, each sample of EtSCT (500 µL) was mixed with 500 µL of 10 mM sodium nitroprusside (Sigma-Aldrich Co.), then incubated at 25℃ for 150 min. This mixture was subsequently added to 500 µL of 1% sulfanilamide solution and 500 µL of 0.1% N-(1-naphthyl) ethylenediamine dihydrochloride solution and incubated at room temperature for 10 min. Next, the absorbance of the reaction mixture was measured at 546 nm using a Versamax plate reader (Molecular Devices). The NO scavenging activity of the EtSCT was expressed as the percentage absorbance relative to a control treated with dimethyl sulfoxide (DMSO, 3047-4460, DAEJUNG Chemicals & Metals Co., LTD, Siheung, Korea).
The animal protocols used in our study were reviewed and approved based on the ethical procedures and scientific care for animals set by the Pusan National University-Institutional Animal Care and Use Committee (PNU-IACUC; Approval Number PNU-2015-0804). Eight-week-old female ICR mice were obtained from Samtako (Osan, Korea) and handled at the Pusan National University Laboratory Animal Resources Center, which is accredited by AAALAC International (Accredited Unit Number-001525) in accordance with the United States National Institutes of Health guidelines and the Korea Food & Drug Administration (KFDA; Accredited Unit Number-00231) in accordance with the Laboratory Animals Act. All mice were provided with a standard irradiated chow diet (Purina Mills, Seoungnam, Korea) ad libitum, and were maintained in a specific pathogen-free state under a strict light cycle (lights on at 06:00 h and off at 18:00 h) at 23±2℃ and 50±10% relative humidity.
Eight-week-old ICR mice (n=28) were assigned to one of the following four groups: control group (n=7), vehicle-treated group (n=7), low dosage EtSCT-treated group (LEtSCT, n=7) and high dosage EtSCT-treated group (HEtSCT, n=7). Vehicle-treated mice received a daily comparable volume of water per body weight via gavage, whereas the others received 50mg/kg/body weight/day of EtSCT (LEtSCT-treated group), and 100 mg/kg/body weight/day of EtSCT (HEtSCT-treated group). The administration period for a toxicity test was citied as the guideline for drug toxicity published by the Korea Food and Drug Administration (KFDA). After EtSCT treatment for 14 days, all mice were immediately sacrificed using CO2 gas to acquire blood and tissue samples for further analysis.
The body weight of ICR mice in subset groups was observed using an electronic balance (Mettler Toledo, Greifensee, Switzerland) every day according to the KFDA guidelines. Finally, the weights of eight organs (brain, testis, liver, kidney, spleen, thymus, heart and lung) collected from the sacrificed mice were determined using the same method employed to detect the body weight.
All mice were sacrificed using CO2 gas at 24 h following the final treatment, after which urine was collected from their bladders and assayed for ketones, ascorbic acid, leucocytes, pH and specific gravity with an URiSCAN optima II urine analyzer (Yeongdong Electronics Co., Ltd., Yongin, Korea). All assays were conducted in triplicate using fresh urine.
Following the final treatment with EtSCT, all mice were fasted for 12 h, after which whole blood was collected from the abdominal vein. Serum was then obtained using serum separating tubes (SST, 365967, BD Microtainer, Franklin Lakes, NJ, USA), incubated for 30 min at room temperature to allow coagulation, and centrifuged at 1,500×g for 15 min at 4℃. The serum concentrations of ALP, ALT, AST, lactate dehydrogenase (LDH), BUN, CRE, total bilirubin (T-BIL) and total protein (TP) were then assayed using an automated chemical analyzer BS-120 (Mindray Medical International Limited, Shenzhen, China). All assays were conducted with fresh serum using standard enzymatic methods and all measurements were conducted in duplicate.
Liver and kidney tissues collected from ICR mice were fixed with 10% formalin for 48 h, embedded in paraffin wax, and then sectioned into 4 µm thick slices. Next, liver and kidney sections were stained with hematoxylin (Sigma-Aldrich Co.) and eosin (Sigma-Aldrich Co.), after which they were examined by light microscopy for alteration of histological structures. Additionally, pathological changes were measured using Leica Application Suite (Leica Microsystems, Wetzlar, Germany).
One-way analysis of variance (ANOVA) was used to identify the significant differences between control, vehicle- and EtSCT treated ICR mice using SPSS for Windows, Release 10.10, Standard Version (SPSS Inc., Chicago, IL, USA). All values are reported as the means±standard deviation (SD). A P value of <0.05 was considered significant.
To analyze the antioxidant properties of EtSCT, the concentration of flavonoids and phenolics were measured using the method described in the materials and methods. As shown in Table 1, EtSCT showed 14.8 mg/mL of flavonoids and 36.2 mg/mL of phenolics. EtSCT also showed a high level of free radical scavenging activity for DPPH (93.1%) and NO (16.2%) (Table 1). Taken together, these results demonstrated that EtSCT has a high level of DPPH and NO scavenging activity.
We observed the clinical phenotypes and mortality of ICR mice for 14 days to investigate the effects of EtSCT on phenotypic toxicity. No significant pathological symptoms (e.g., depression, hypokinesia, gait abnormality and tremors) were detected in any EtSCT treated group regardless of the EtSCT concentration. In addition, no mortality was observed in the three EtSCT treated groups. There were also no significant differences in body and organ weight between vehicle- and EtSCT-treated groups, although the thymus weight was slightly lower in the HEtSCT treated group than the vehicle treated group (Figure 1B and Table 2). Furthermore, the brain, ovary, kidney, spleen, liver, thymus, heart, and lungs showed normal morphology in all EtSCT-treated groups compared to the vehicle-treated group (data not shown). Taken together, these results indicate that EtSCT does not induce any significant alterations in pathological symptoms or mortality. Furthermore, the minimum lethal dose (MLD) was found to be higher than 100 mg/kg of EtSCT.
To investigate the effects of EtSCT treatment on the urine of ICR mice, seven urine factors were measured in the EtSCT treated group. No significant changes in the levels of urine parameters including ketones, ascorbic acid, leucocytes, pH and specific gravity, were detected in the EtSCT-treated group relative to the vehicle treated group (Table 3). These results indicate that EtSCT treatment for 14 days does not induce any toxicity toward urine parameters in ICR mice.
We also investigated the liver toxicity of EtSCT in ICR mice using serum biochemical and histological analyses. The level of ALT, AST, ALP and LDH representing liver toxicity were not changed in the EtSCT-treated group although LDH levels were slightly decreased in the EtSCT treated group compared with the control group (Table 4). Furthermore, no significant pathological changes such as inflammation, necrosis, apoptosis, and fibrosis were observed in the H&E stained liver tissue of vehicle- and EtSCT-treated groups. Finally, the majority of the histological structures were very similar to those of the vehicle treated group (Figure 2A). Taken together, these results suggest that EtSCT treatment does not induce any significant toxicity in the livers of ICR mice.
Finally, to investigate whether EtSCT treatment for 14 days induced kidney toxicity in ICR mice, alterations in the biochemical factors representing kidney toxicity and histopathological features were measured in the blood serum and kidney tissue. The level of BUN or CRE in the EtSCT-treated group was very similar to that of the vehicle-treated group (Table 4). Furthermore, no specific pathological symptoms such as degeneration and necrosis of the glomerulus and renal tubes were observed in the EtSCT-treated groups (Figure 2B). Overall, these results suggest that EtSCT treatment dose not induced significant toxicity in kidneys tissue of ICR mice.
SC, a solitary oviparous hermaphrodite tunicate, is distributed in East Asia including Korea, as well as in Australia, New Zealand, North America and Europe [15161718]. In Korea, annual production in suspended culture increased to 2,759 t in 2013 due to demand for it as a raw material in food preparation [1920]. However, increased SC results in increased SCT wastes, which causes environmental problems [221]. To provide a solution for this problem, some studies have investigated the possibility for use of novel bioactive materials. As part of the above studies, we investigated the toxicity of EtSCT in the liver and kidney of ICR mice after short term administration. Our results demonstrated that treatment with EtSCT for 14 days did not induce significant toxicity against the livers and kidneys of ICR mice at less than 100 mg/kg body weight.
Few studies have investigated the toxicity of compounds and extract purified from SCT to date. Significant toxicity of chondroitin sulfate extracted from SCT was detected in JB6 P+ cells treated with more than 5 mg/mL of concentration [9]. However, regenerated CF prepared from SCT did not induce any significant toxicity after application onto surgical wounds for 2 weeks [4]. Moreover, cutaneous wounds in SD rats treated with hydrocolloid membrane containing SCT (HSM-SCT) did not induce any specific toxicity on body weight, or biological function of the liver and kidney [6]. Furthermore, the levels of serum biochemical factors representing liver and kidney toxicity were maintained as constant levels in SCT-CF implanted into the subcutaneous pocket compared with the control group, while no significant alterations in the epidermal hyperplasia, infiltration of immune cells, redness and edema were observed in the implanted area of these animals [3]. In the present study, ICR mice administered two different concentrations of EtSCT (50 and 100 mg/kg bw) for 14 days showed no significant changes in pathological toxicity toward the liver and kidney. These results are similar to those of a previous study in which the toxicity of CF and HCM containing SCT in animals was investigated.
In this study, we selected two target organs, the liver and kidney, to investigate the toxicity of EtSCT in the animals. Among several organs, the liver is the primary target responsible for metabolism of chemicals, drugs, alcohol, food additives, pesticides and natural products, while the kidney is commonly considered a toxicity indicator; therefore, it is often weighed and analyzed in toxicological experiments [2223]. Liver toxicity is also evaluated based on alterations in the levels of ALT, AST, ALP and LDH as well as histological alterations such as the degree of steatosis, lobular inflammation, hepatocellular ballooning, and fibrosis [2425]. Increased of BUN, CRE, and red blood cells in the glomerulus and tubule luminal diameter upon histological analysis are considered key markers of kidney toxicity [2627]. In this study, serum biochemical markers and histopathological indicators reported in previous studies were used as markers of liver and kidney toxicity. Most of our results indicated that these markers successfully reflected the absence of toxicity in the livers of ICR mice treated with two different doses of EtSCT for 14 days.
Although most factors indicated the absence of liver and kidney toxicity, the weight of the thymus was significantly decreased in the HEtSCT treated group relative to the vehicle treated group as shown Table 2. However, no reports have shown evidence of a direct correlation between thymus weight and HEtSCT treatment. Meanwhile, several reports provide important clue for above issue. The weight of thymus was significantly influenced by dietary fat saturation and concentration [28]. Moreover, age-dependent degeneration of the thymus was delayed by administration of low fat diets to SD rats, while a significant decrease in thymus weight was observed in a 10% mayonnaise treated group [2930]. Therefore, the decrease in thymus weight observed in the present study may be correlated with the composition of HEtSCT.
Taken together, these results indicate that short term administration of EtSCT had no toxic effects on liver and kidney tissues of ICR mice. Furthermore, these findings provide vital information regarding the development of EtSCT as a therapeutic drug for the treatment of various chronic diseases.
Acknowledgments
We thank Jin Hyang Hwang for directing the animal facility and breeding SPF animals at the Laboratory Animal Resources Center. This study was supported by a grant to Professor Dae Youn Hwang from the Korea Institute of Marine Science & Technology Promotion (112088-3).
References
1. Richard CK, Walter YR. Market potential for Styela clava, a non-indigenous pest invading New England coastal waters. Aquat Invasions. 2009; 4(1):295–297.
2. Lee SM, Kang EJ, Go TH, Jeong SY, Park GT, Lee HS, Hwang DY, Jung YJ, Son HJ. Screening of biological activity of solvent extract from Styela clava tunic for fishery waste recycling. J Environ Sci Int. 2014; 23(1):89–96.
3. Song SH, Kim JE, Lee YJ, Kwak MH, Sung GY, Kwon SH, Son HJ, Lee HS, Jung YJ, Hwang DY. Cellulose film regenerated from Styela clava tunics have biodegradability, toxicity and biocompatibility in the skin of SD rats. J Mater Sci Mater Med. 2014; 25(6):1519–1530. PMID: 24577945.
4. Jung YJ. Properties of regenerated cellulose films prepared from the tunicate Styela clava. J Korean Fish Soc. 2008; 41(4):237–242.
5. Seong KY, Koh EK, Lee SH, Kwak MH, Son HJ, Lee HS, Hwang DY, Jung YJ. Preparation and characterization of high absorptive cellulose film derived from Styela clava tunic for wound dressing. Tex Color Fin. 2015; 27(1):70–79.
6. Kwak MH, Go J, Kim JE, Lee YJ, Lee SH, Lee HS, Son HJ, Jung YJ, Hwang DY. Property and efficacy analysis of hydrocolloid membrane containing Styela clava tunic on the wound repair of skin in SD rats. Biomater Res. 2013; 17(3):91–101.
7. Kim SM, Lee JH, Cho JA, Lee SC, Lee SK. Development of a bioactive cellulose membrane from sea squirt skin for bone regeneration-A preliminary research. J Korean Assoc Oral Maxillofac Surg. 2005; 31:440–453.
8. Ahn SH, Jung SH, Kang SJ, Jeong TS, Choi BD. Extraction of glycosaminoglycans from Styela clava tunic. Korean J Biotechnol Bioeng. 2003; 18(3):180–185.
9. Xu CX, Jin H, Chung YS, Shin JY, Woo MA, Lee KH, Palmos GN, Choi BD, Cho MH. Chondroitin sulfate extracted from the Styela clava tunic suppresses TNF-alpha-induced expression of inflammatory factors, VCAM-1 and iNOS by blocking Akt/NF-kappaB signal in JB6 cells. Cancer Lett. 2008; 264(1):93–100. PMID: 18295395.
10. Loda MN, Kang SJ, Choi BD. Antioxidative activity of carotenoids in Mideodeok Styela clava. Fish Aquat Sci. 2011; 14(4):243–249.
11. Singleton VL, Rossi JA. Colorimetry of total phenolics with phosphomolybdic-phosphotungstic acid reagents. Am J Enol Vitic. 1965; 16(3):144–158.
12. Zhishen J, Mengcheng T, Jianming W. The determination of flavonoid contents in mulberry and their scavenging effects on superoxide radicals. Food Chem. 1999; 64:555–559.


13. Oh H, Ko EK, Kim DH, Jang KK, Park SE, Lee HS, Kim YC. Secoiridoid glucosides with free radical scavenging activity from the leaves of Syringa dilatata. Phytother Res. 2003; 17(4):417–419. PMID: 12722154.
14. Marcocci L, Maguire JJ, Droy-Lefaix MT, Packer L. The nitric oxide-scavenging properties of Ginkgo biloba extract EGb 761. Biochem Biophys Res Commun. 1994; 201(2):748–755. PMID: 8003011.
15. Millar H. The identity of the ascidians Styela mammiculata Carlisle and Styela clava Herdman. J Mar Biol Assoc UK. 1960; 39:509–511.
16. Carlisle DB. Styela mammiculata n. sp. a new species of ascidian from the Plymouth area. J Mar Biol Assoc UK. 1954; 33:329–334.
17. Kang PA, Kim Y, Yoon DS. Studies on the hanging culture of oyster, Crassostrea gigas, in the Korean coastal waters 4 On the fouling organisms associated with culturing oysters at the oyster culture farms in Chungmu. Bull Natl Fish Res Dev Agency (Korea). 1980; 25:29–34.
18. Davis MH, Davis ME. First record of Styela clava (Tunicata: Ascidiacea) from the Mediterranean Sea. Aquat Invasions. 2008; 3:125–132.
19. Park JH, Suh YC. GIS-Based suitable site selection for aquaculture using scope for growth of Styela clava. J Korean Assoc Geogr Inf Stud. 2013; 16(3):81–90.
20. Shin YK, Park JJ, Park MS, Myeong JI, Hur YB. Effect of temperature and dissolved oxygen on the survival rate and physiological response of the warty sea squirt Styela clava. Korean J Environ Biol. 2014; 32(3):216–224.
21. Ahn SH, Jung SH, Kang SJ, Jeong TS, Choi BD. Extraction of glycosaminoglycans from Styela clava tunic. Korean J Biotechnol Bioeng. 2003; 18:180–185.
22. Bissell DM, Gores GJ, Laskin DL, Hoofnagle JH. Drug-induced liver injury: mechanisms and test systems. Hepatology. 2001; 33(4):1009–1013. PMID: 11283870.


23. Michael B, Yano B, Sellers RS, Perry R, Morton D, Roome N, Johnson JK, Schafer K, Pitsch S. Evaluation of organ weights for rodent and non-rodent toxicity studies: a review of regulatory guidelines and a survey of current practices. Toxicol Pathol. 2007; 35(5):742–750. PMID: 17849357.


24. Kim JJ, Kim SJ, Kim SH, Park HR, Lee SC. Antioxidant and anticancer activities of extracts from Styla plicata. J Korean Soc Food Sci Nutr. 2005; 34:937–941.
25. Kim JJ, Kim SJ, Kim SH, Park HR, Lee SC. Antioxidant and anticancer activities of extracts from Styela clava according to the processing methods and solvents. J Korean Soc Food Sci Nutr. 2006; 35:278–283.
26. Choi SY, Kim YC. Alleviative effects of jujube water extract on the inflammation and barrier damage in hairless mice skin. Korean J Environ Toxicol. 2009; 24(4):351–357.
27. Yamaguchi Y, Takahashi K, Zmudzka BZ, Kornhauser A, Miller SA, Tadokoro T, Berens W, Beer JZ, Hearing VJ. Human skin responses to UV radiation: pigment in the upper epidermis protects against DNA damage in the lower epidermis and facilitates apoptosis. FASEB J. 2006; 20(9):1486–1488. PMID: 16793869.


28. Erickson KL, McNeill CJ, Gershwin ME, Ossmann JB. Influence of dietary fat concentration and saturation on immune ontogeny in mice. J Nutr. 1980; 110(8):1555–1572. PMID: 7400846.


29. Cho MS, Kim WY. Effects of dietary fat level on the aging process of the fibroblast cells and immune function in rats of different ages. Korean J Nutr. 1991; 24:431–441.
30. Cho YJ. Effects of dietary supplement of mayonnaise on lipid metabolism in rats. J Korean Soc Food Sci Nutr. 1999; 28(4):895–900.
Figure 1
Preparation procedures of SCT powder and alteration in body weight. (A) Preparation procedures and analysis of SCT powder. (a-c) Following collection from the beach, samples were treated with 10% H2O2 solution and then subjected to milling. (d) Particle size analyses, showing individual and cumulative distribution of particles at the same time. (B) The body weight of subset groups was measured using an electrical balance.
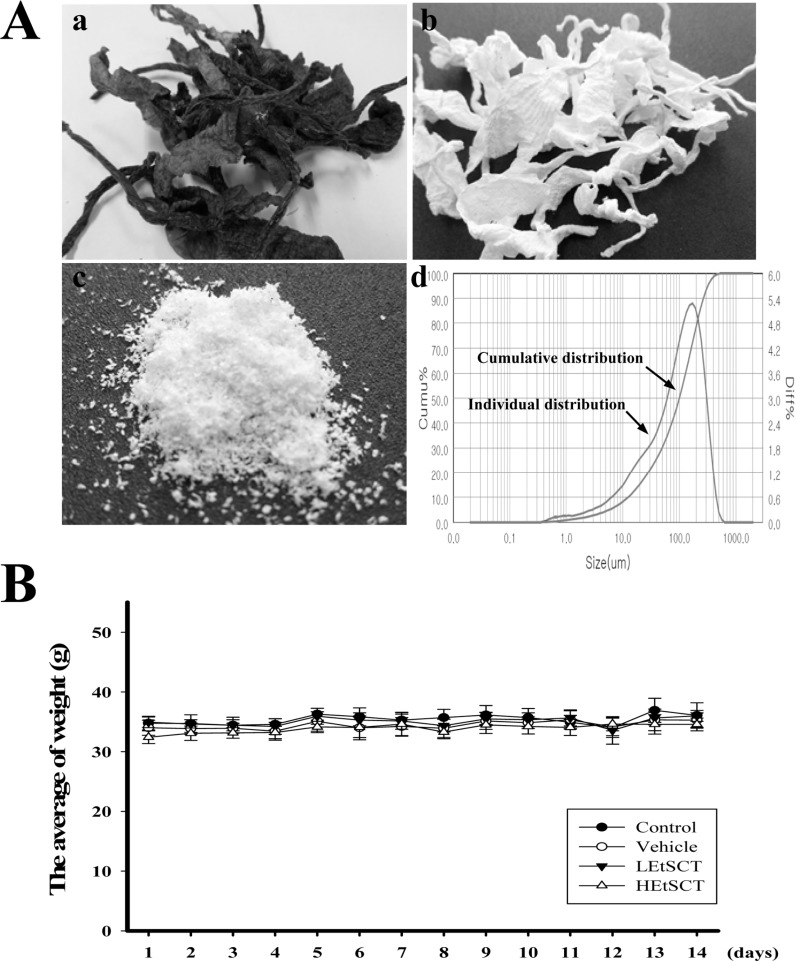
Figure 2
Effects of EtSCT treatment on histological features of the liver and kidney. (A) Liver tissues were stained with H&E and cellular morphology was viewed at 400× magnification. (B) Kidney tissues were stained with H&E, and cellular morphology was viewed at 400× magnification. Control; no treated group, Vehicle; vehicle treated group, LEtSCT; low dose of EtSCT treated group, HEtSCT; high dose of EtSCT treated group.
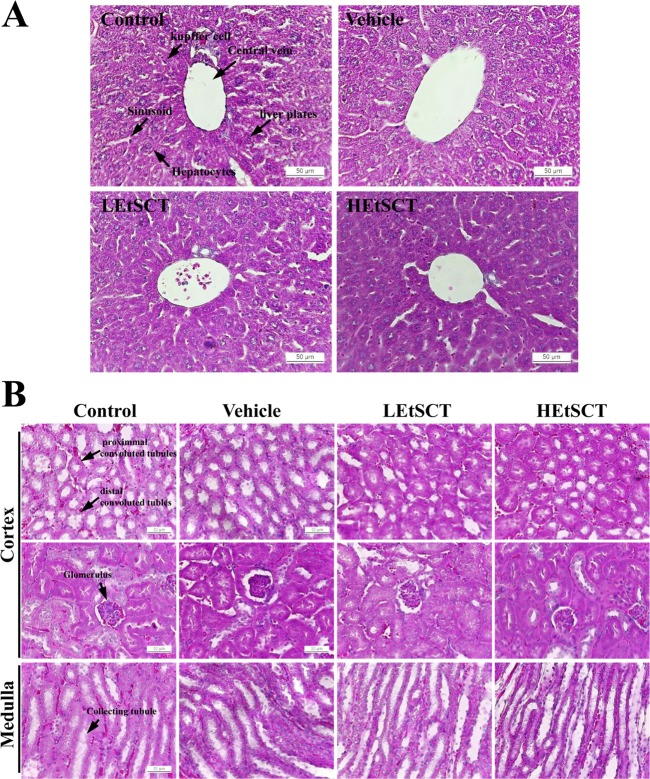
Table 1
Components and properties of EtSCT
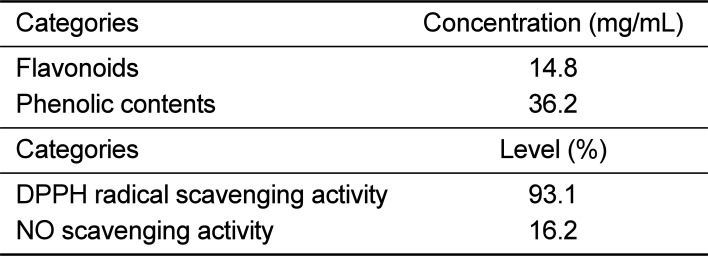
Categories | Concentration (mg/mL) |
---|---|
Flavonoids | 14.8 |
Phenolic contents | 36.2 |
Categories | Level (%) |
---|---|
DPPH radical scavenging activity | 93.1 |
NO scavenging activity | 16.2 |
Table 2
Alteration of organ weights of ICR mice
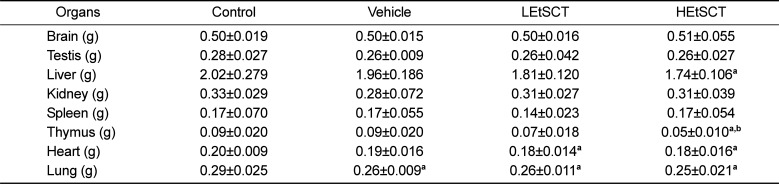
*The data is reported as the mean±SD from three replicates. a, P<0.05 is the significance level compared to the control group. b, P<0.05 is the significance level compared to the vehicle group. Control; no treated group, Vehicle; vehicle treated group, LEtSCT; low dose of EtSCT treated group, HEtSCT; high dose of EtSCT treated group.
Table 3
Alteration of urine parameters of ICR mice

Table 4
Alteration of serum parameters of ICR mice
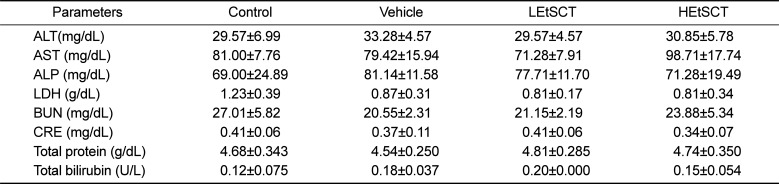