Abstract
To evaluate the hepatotoxicity and nephrotoxicity of Galla Rhois (GR) toward the liver and kidney of ICR mice, alterations in related markers including body weight, organ weight, urine composition, liver pathology and kidney pathology were analyzed after oral administration of 250, 500 and 1,000 mg/kg body weight/day of gallotannin-enriched extract of GR (GEGR) for 14 days. GEGR contained 68.7±2.5% of gallotannin, 25.3±0.9% of gallic acid and 4.4±0.1% of methyl gallate. Also, the level of malondialdehyde (MDA), a marker of lipid peroxidation, was decreased with 19% in the serum of high dose GEGR (HGEGR)-treated mice. The body and organ weight, clinical phenotypes, urine parameters and mice mortality did not differ among GEGR-treated groups and the vehicle-treated group. Furthermore, no significant increase was observed in alkaline phosphatase (ALP), alanine aminotransferase (ALT), aspartate aminotransferase (AST), lactate dehydrogenase (LDH), blood urea nitrogen (BUN) and the serum creatinine (Cr) in the GEGR-treated group relative to the vehicle-treated group. Moreover, the specific pathological features induced by most toxic compounds such as CCl4 were not observed upon liver and kidney histological analysis. Overall, the results of the present study suggest that GEGR does not induce any specific toxicity in liver and kidney organs of ICR at doses of 1,000 mg/kg body weight/day, indicating that this is no observed adverse effect level (NOAEL).
Galla Rhois (GR) is a type of excrescence formed by parasitic aphids, primarily Schlechtendalia chinensis Bell, on the leaf of sumac, Rhus javanica L. (Anacardiaceae) [12]. The extract of GR contains several compounds including methyl gallate, 3-galloyl-gallic acid, 4-galloyl-gallic acid isomers, 1,2,3,4,6-penta-O-galloyl-β-D-glucose, gallic acid methyl ester and gallic acid [3]. In traditional Korean medicine, GR has long been used for treatment of various diseases including diarrhea, seminal emissions, excessive sweating, bleeding and chronic cough, although there is little scientific evidence supporting these pharmacological effects [456]. However, several studies have provided some clues for the therapeutic effects of GR against various human diseases and their mechanisms. Several compounds and extracts purified from GR showed good antibacterial activity against many pathogenic bacteria strains, including Salmonella spp., Escherichia coli, Eimeria tenella, Brucella abortus, Staphylococcus aurous and Clostridium perfringens [78910]. Methyl gallate and ethyl gallate isolated from GR were also found to exert significant anti-inflammatory activity in lipopolysaccharide (LPS)-stimulated RAW264.7 macrophages via the induction of heme oxygenase 1 and suppression of iNOS/COX-2 [1112]. Moreover, galloyl glucose (GG6-10) isolated from GR inhibited the invasion of metastatic HT-1080 cells into a reconstituted basement membrane via inhibition of the gelationolysis mediated by MMP-2 and -9, while ellagic acid extracted from GR showed anticancer activity against nasopharyngeal carcinoma cells via down-regulated expression of COX-2 and stathmin [1314]. Furthermore, oral administration of GR 85% methanol extract reduced brain infarct volume by 37.5% and lipid peroxidation in middle cerebral artery occlusion, while also improving sensory motor function in a transient focal cerebral ischemia rat model [15]. Moreover, tacrine, nitrofurantoin and tert-butyl hydroperoxide-induced hepatotoxicity in HepG2 cells was greatly retained by an equilibrium mixture of 3-galloyl-gallic acid, 4-galloyl-gallic acid isomers, and 1,2,3,4,6-penta-O-galloyl-β-D-glucose isolated from GR [316]. Despite the increase in attention and publications regarding the therapeutic effects of GR, its toxicity toward specific organs of mice has not been investigated to date.
Therefore, in this study, we investigated GEGR toxicity in the liver and kidney of ICR mice following short-term treatment. The results indicate that there is a scientific basis for determining the optimal concentration of GEGR as a therapeutic drug for the treatment of human chronic diseases.
Briefly, samples of original GR were collected from plantations in the Hongcheon area of Korea during October of 2013 and dried in a hot-air drying machine (JSR, Seoul, Korea) at 60℃ for 24 h. Voucher specimens of dried GR (WPC-14-001) were deposited in the functional materials bank of the PNU-Wellbeing RIS Center at Pusan National University. GEGR was prepared using a modified version of previously described methods [17]. Briefly, dried samples of GR were reduced to powder using an electric blender, after which they were extracted in water at 90℃ for 9 h in a fixed liquor ratio (solid GR powder/water ratio, 1:10) using circulating extraction equipment (IKA Labortechnik, Staufen, Germany). The extracts were subsequently filtered through a 0.4 µm filter, after which they were concentrated by vacuum evaporation and lyophilization using circulating extraction equipment (IKA Labortechnik, Staufen, Germany). Finally, the powder was dissolved in distilled water (dH2O) to 1 mg/mL, then further diluted with phosphate buffered saline (PBS) to the required concentration.
To analyze the three main components in GEGR, gallotannin (IUPAC name; 3,5-dihydroxy-2-(3,4,5-trihydroxybenzoyl) oxy-6-[(3,4,5-trihydroxybenzoyl)oxymethyl] oxan-4-yl] 3,4,5-trihydroxybenzoate, MW: 1701.20 g/mol, Sigma-Aldrich Co., St. Louis, MO, USA), gallic acid monohydrate (IUPAC name; 3,4,5-trihydroxybenzoic acid, MW: 170.12 g/mol, Sigma-Aldrich, St. Louis, MO, USA) and methyl gallate (IUPAC name; methyl 3,4,5-trihydroxybenzoate, MW: 184.15 g/mol, Sigma-Aldrich) were used as standard compounds. The wavelengths of the maximum absorption of pure gallic acid, pure methyl gallate, commercial gallotannin, and gallnut extract were 212/257, 214/268, 213/278 and 212/275 nm, respectively. The UV-VIS spectra for pure gallic acid, pure methyl gallate, pure gallotannin, and gallnut extract showed two bands at 212-214 nm and 257-278 nm, which were both assigned to the π→π* transitions of the given aromatic units and C=O groups in the UV-VIS region (Albu et al., 2009). Finally, the UV-Vis spectra were analyzed using a curve-resolving technique based on linear least-squares analysis to fit the combination of the Lorentzian and Gaussian curve shapes.
The animal protocol used in this study was reviewed and approved by the Pusan National University-Institutional Animal Care and Use Committee (PNU-IACUC; Approval Number PNU-2014-0746). All ICR mice were handled in the Pusan National University-Laboratory Animal Resources Center, which is accredited by the Korea Food and Drug Administration (FDA) in accordance with the Laboratory Animal Act (Accredited Unit Number-000231) and AAALAC International according to the National Institutes of Health guidelines (Accredited Unit Number; 001525). Eight-week-old female ICR mice were purchased from Samtako (Osan, Korea) and provided with ad libitum access to a standard irradiated chow diet (Samtako) consisting of moisture (12.5%), crude protein (25.43%), crude fat (6.06%), crude fiber (3.9%), crude ash (5.31%), calcium (1.14%), phosphorus (0.99%) and water throughout the feeding study. During the experiment, these mice were maintained in a specific pathogen-free state under a strict light cycle (lights on at 08:00 h and off at 20:00 h) at 23±2℃ and 50±10% relative humidity.
Female ICR mice (n=28) were assigned to either a vehicle-treated group (n=7), low dosage GEGR-treated group (LGEGR, n=7), middle dosage GEGR-treated group (MGEGR, n=7), or high dosage GEGR-treated group (HGEGR, n=7). Additionally, one group of ICR mice received an equivalent volume of water via gavage daily (vehicle-treated group) as a control, whereas the others groups received 250 mg/kg body weight/day of GEGR (LGEGR), 500 mg/kg body weight/day of GEGR (MGEGR), or 1,000 mg/kg body weight/day of GEGR (HGEGR) via gavage for 14 days. The administration period for the toxicity test was as described in the Guideline for Drug Toxicity published by the Korea Food and Drug Administration. After GEGR treatment for 14 days, all mice were immediately sacrificed using CO2 gas and urine, blood, liver and kidney samples were prepared.
Clinical signs and the number of animals that died were recorded more than twice a day for 14 days. In addition, alterations in body weight were observed using an electronic balance (Mettler Toledo, Greifensse, Switzerland) every day according to the KFDA guidelines. Finally, the weights of eight organs (brain, ovary, kidney, spleen, liver, thymus, heart, and lung) collected from the sacrificed ICR mice were determined using the same method employed to detect the body weight.
Urine was collected from the bladders of sacrificed mice and assayed for bilirubin, urobilinogen, ketones, protein, pH, specific gravity and leucocytes using a URiSCAN optima II urine analyzer (Yeongdong Electronics Co., Ltd., Yongin, Korea). All assays were conducted in triplicate using fresh urine.
After fasting for 8 h, whole blood of each mouse in the subset groups was collected from their abdominal veins and incubated for 1 h at room temperature. Serum was then obtained by centrifugation of blood and analyzed for ALP, ALT, AST, LDH, BUN and CRE using an Automatic Serum Analyzer (HITACHI 747, Tokyo, Japan). All assays were conducted in triplicate using fresh serum.
The MDA level was assayed using a Lipid Peroxidation (MDA) Assay Kit (Cat. No. MAK085, Sigma-Aldrich Co.) according to the manufacturer's protocols. Briefly, the sera from each mice was mixed with MDA Lysis Buffer containing butylhydroxytoluene (BHT), after which the homogenates were stored at -20℃ until analysis. The sample or standards and TBA solution (70 mM thiobarbituric acid, 5.0M glacial acetic acid) were incubated in micro-centrifuge tube at 95℃ for 60 min, then cooled to room temperature in an ice bath for 10 min, after which the reaction absorbance at 450 nm was read using a Vmax ELISA reader (Molecular Devices).
Liver and kidney tissues dissected from mice and fixed in 4% neutral buffered formaldehyde (pH 6.8) overnight, after which each tissue was dehydrated and embedded in paraffin. Next, a series of liver and kidney sections (4 µm) was cut from paraffin-embedded tissue using a Leica microtome (Leica Microsystems, Bannockburn, IL, USA). These sections were then deparaffinized with xylene, rehydrated with ethanol at a graded decreasing concentration of 100-70%, and finally washed with distilled water. The slides with liver and kidney sections were stained with hematoxylin (Sigma-Aldrich Co.) and eosin (Sigma-Aldrich Co.), then washed with dH2O, after which pathological changes were measured using the Leica Application Suite (Leica Microsystems, Wetzlar, Germany).
Significant differences between the vehicle- and GEGR-treated groups were identified by one-way analysis of variance (ANOVA) using SPSS for Windows, Release 10.10, Standard Version (SPSS Inc., Chicago, IL, USA). All values are reported as the mean±standard deviation (SD) and a P value of <0.05 was considered as a significance.
As shown in Table 1, three functional compounds including gallotannin, gallic acid and methyl gallate were analyzed in GEGR. Among these compounds, gallotannin was found to be the highest level (68.7±2.5%), followed by gallic acid (25.3±0.9%) and methyl gallate (4.4±0.1%), respectively. Therefore, the results indicate that GEGR may contain high levels of gallotanin and gallic acid, but low levels of methyl gallate.
To investigate the inhibitory activity of GEGR against the oxidative stress, MDA concentration was measured in the serum of subset group. The concentration of MDA was decreased as increasing concentration of GEGR, although a significant decrease was detected in olny HGEGR-treated group compared with vehicle-treated group (Figure 1). Therefore, the present results suggest that HGEGR treatment for 14 days can inhibit the lipid peroxidation in the serum of ICR mice.
To investigate whether GEGR treatment affects phenotypical toxicity, the clinical phenotypes and mortality were observed in ICR mice for 14 days. No significant pathological symptoms (e.g., melancholy, hypokinesia, gait abnormality and tremors) were detected in any mice treated with three different concentrations of GEGR. In addition, dead mice were not observed in the same group. These results show that GEGR does not induce any pathological symptoms or mortality and thus the minimum lethal dose (MLD) is higher than 1,000 mg/kg of GEGR.
To examine the effects of GEGR treatment on the weights of body and organs and urine biochemical composition of ICR mice, the weights of eight organs and seven urine factors were measured. No significant difference in body weight was observed between the vehicle- and GEGR-treated group throughout the 14 day experimental period (Data not shown). Moreover, no significant differences in body and organ weight at day 14 were observed between vehicle- and GEGR-treated groups (Table 2). Furthermore, no morphological changes in the brain, ovary, kidney, spleen, liver, thymus, heart, and lungs were observed in any of the GEGR-treated groups when compared to the vehicle-treated group (Data not shown). Finally, the levels of urine parameters including bilirubin, urobilinogen, protein, pH, specific gravity and leucocytes in the GEGR-treated group were very similar to those of the vehicle-treated group, although the ketone level was slightly higher in the same group (Table 3). These results indicate that GEGR treatment for 14 days does not induce any toxicity toward body weight, organ weights and urine parameters in ICR mice.
To investigate whether GEGR treatment for 14 days induced hepatotoxicity in ICR mice, alteration of several enzymes related to liver metabolism were measured in the serum. There was no significant increase in liver toxicity indicators such as ALP, AST, ALP and LDH in the GEGR-treated group (Figure 2A). To confirm the absence of toxicity, liver sections were stained with H&E and observed microscopically. There were no significant pathological changes such as inflammation, necrosis, apoptosis, and fibrosis observed in the liver tissue of vehicle- and GEGR-treated groups. Additionally, its histological structure was very similar to that of the vehicle treated group (Figure 2B). Therefore, these results suggest that GEGR treatment does not induce hepatotoxicity in the liver of ICR mice.
Finally, the nephrotoxicity of GEGR was investigated in ICR mice using serum biochemical and histological analyses. Serum biochemical analysis revealed no significant alterations in BUN or CRE in the GEGR-treated group relative to the vehicle-treated group (Figure 3A). Furthermore, histological analysis revealed that specific pathological symptoms such as degeneration and necrosis of the glomerulus and renal tubes were not observed in any GEGR-treated groups (Figure 3B). These results indicate that short-term GEGR treatment does not induce nephrotoxicity in kidney tissue of ICR mice.
Natural products have long been considered as a source of leads for the development of therapeutic drugs available for the treatment of human diseases because they serve as active principles in drugs and as templates for the synthesis of new drugs [1819]. However, there are some problems that must be overcome to successfully develop new drugs, including the large-scale procurement of sufficient source materials for bulk production, the potency and inherent toxicity of many natural products and the development of suitable vehicles and dosing schedules for the administration of new drugs to clinical patients [20]. Among these problems, toxicity has long been considered one of the most important issues during preclinical investigations of natural products. In this study, we investigated the hepatotoxicity and nephrotoxicity of GEGR in ICR mice after short term administration. The results demonstrated that treatment with GEGR did not induce significant toxicity against the liver and kidney of ICR mice at less than 1,000 mg/kg.
Lipid peroxidation is known as an indicator of oxidative stress as well as a well-established mechanism of cellular injury in cells and tissues of plants and animals [2122]. MDA is the most abundant lipid peroxides and an important reactive carbonyl compounds during lipid peroxidation [23]. A significant increase of MDA levels has been associated with a variety of chronic diseases including diabetes, cancer and Alzheimer's disease in both humans and animal models [242526]. Furthermore, this level was rapidly deceased after the treatment of medical plants [27] and several natural products including antioxidant compounds [2829]. Especially, tannic acid, gallic acid, ellagic acid, and propyl gallate could be effectively prevented the lipid peroxidation induced by H2O2 in IMR-90 cells [30], while gallic acid suppressed lipid peroxidation in sodium fluoride (NaF)-induced oxidative stress of Wistar rats [31]. In the present study, GEGR contained gallotannin, gallic acid and methyl gallate suppressed the lipid peroxidation in the serum of ICR mice. Therefore, our results provide addition evidence that tannin related compounds could contribute to the prevention of lipid peroxidation in animals system.
Ketone is known as metabolic end products of fatty acid metabolism in the liver [32]. The concentration of ketone in the urine can be affected with various abnormal physical conditions although only small amount of ketone are excreted during the normal condition. Especially, the main reasons for abnormally large quantities of ketone include abnormal food or nutrition intake, disorders of increased metabolism, cute or severe illness, burns, fever, hyperthyroidism, lactation and pregnancy [3334]. In the present study, GEGR-treated group showed high level of ketone concentration compared with vehicle-treated group regardless of GEGR concentration. We believe that the increase of ketone concentration in GEGR-treated group is attributable to the administration of abnoraml food and nutrition for 14 days.
Tannin, one of major components of GR, is naturally distributed in the leaves, twigs, bark, wood and fruit of many species of plants utilized for food component [35]. Although tannins have been shown to have low acute, subchronic and chronic oral toxicities in animals studies, humans consume large amounts of these compounds in coffee, tea, wine, tobacco, catechu, pomegranates, legumes and chocolate [36]. After oral administration of tannins to rabbits, they were absorbed by the gastrointestinal tract, metabolized and excreted within 24 hr [37]. Also, tannins have been shown to exert low acute oral toxicity when administrated to rats [38]. Although the oral LD50 values of various tannins-contained sources including Aleppo, tara, Chinese, Sililian sumac and Douglas fir were found to range from 1,550 to 7,500 mg/kg [39], the LD50 of tannins was found to be 2.26±0.083 g/kg bw [38]. However, subchronic oral toxicity analysis revealed no significant differences in body weight, food intake, liver and kidney weight or histopathology between male and female rats administered 8, 80 or 800 mg/kg bw/day of Aleppo or tara tannin for 12 weeks [40]. In the present study, ICR mice administered three different concentrations of GEGR (250, 500 and 1,000 mg/kg BW) for 14 days showed no significant changes in pathological toxicity, hepatotoxicity or nephrotoxicity. These findings are similar to those of a previous study in which the toxicity of extract containing tannins at 1,550 to 7,500 mg/kg was investigated.
ICR mice were widely applied to various research fields including toxicology, neurobiology, oncology, infection, pharmacology and safety test because they characterized by rapid growth, high productivity, docile nature and low incidence of spontaneous tumor [4142]. Especially, toxicity test for many natural products and chemical compounds were conducted using ICR mice in the previous studies [434445]. Therefore, we selected ICR mice to evaluate the toxicity of GEGR which was considered as one of natural products. The present results indicated that ICR mice could be successfully applied to investigate the toxicity of GEGR.
Meanwhile, there is one report on the acute and subchronic toxicity and safety pharmacology of GR solution. Xiang et al. [46] showed that the LD50 of GR solution was greater than 500 mg/kg/day and NOAEL of GR solution was lesser than 1,500mg/kg/day. Furthermore, the safety pharmacology study indicated that GR solution did not induce any side effects to SD rats in respiratory system, cardiovascular system and central nerve system. In our study, any significant toxicity on the liver and kidney organs of ICR mice did not detected at 1,000mg/kg/day of GEGR. These results are in agreement with the previous report although the extraction method and animals used were differenced.
The liver is the primary target responsible for metabolism of chemicals, drugs alcohol, food additives, pesticides and natural products [47]. During evaluation of liver toxicity caused by various compounds, the degree of steatosis, lobular inflammation, hepatocellular ballooning, and fibrosis are often used as toxicity markers [48]. Liver toxicity has also been evaluated based on alterations in the levels of ALT, AST, ALP and LDH. Among these enzymes, AST and ALT have been reported as noninvasive methods of evaluating liver fibrosis and cirrhosis [49] and used as diagnostic markers for identification of the appearance of cirrhosis in patients infected by hepatitis C virus [50]. Moreover, the level of ALP and LDH can be used to diagnose the condition for liver disease [51]. ALP activity is enhanced by diseases that lead to increased bile secretion, such as primary biliary cirrhosis and extra- and intra-hepatic cholestasis [52]. Moreover, LDH is present in almost all animal tissues and upregulated in response to cell damage [53]. In this study, serum biochemical markers and histopathological indicators reported in previous studies were used as indicators of liver toxicity. The results indicate that they reflected no toxicity in the liver of ICR mice treated with three different doses of GEGR for 14 weeks.
The kidney is commonly considered a toxicity indicator; therefore, it is often weighed and analyzed in toxicological experiments [54]. Cellular damage can be caused kidney dysfunction owing to metabolites of the toxic chemicals that are excreted from the kidneys [55], and the degree of kidney dysfunction is evaluated based on increases in red blood cells in the glomerulus and tubule luminal diameter upon histological analysis [56]. In addition, the serum levels of BUN and CRE are considered key markers of nephrotoxicity, and their levels increase dramatically in patients with renal trauma [57]. Accordingly, these parameters are commonly used to detect kidney toxicity in preclinical and clinical studies, as well as during routine clinical care [58]. In the present study, no significant alterations in kidney weights or histological changes were observed throughout the experiment. Additionally, the levels of BUN and CRE in the serum of ICR mice treated with GEGR for 14 weeks did not differ.
Taken together, these results indicate that GEGR has no toxic effects on liver and kidney tissues of ICR mice. Furthermore, these findings provide vital information regarding the development of GEGR as a therapeutic drug for the treatment of various chronic diseases.
Acknowledgments
We thank Professor Young Hee Lee in Department of Organic Material Science and Engineering of Pusan National University for kindly providing Galla Rhios samples. This study was supported by grants to Dr. Dae Youn Hwang from the Korea Institute of Planning Evaluation for Technology of Food, Agriculture, Forestry and Fisheries (114034-03-1-HD030).
References
1. Lee SM, Lee DW, Park JD, Kim JI. Study on formation and development of gall in Rhus javanica. Korean J Appl Entomol. 1997; 36(1):83–87.
2. Ren Z, Zhu B, Wang D, Ma E, Su D, Zhong Y. Comparative population structure of Chinese sumac aphid Schlechtendalia chinensis and its primary host-plant Rhus chinensis. Genetica. 2008; 132(1):103–112. PMID: 17503190.
3. An RB, Oh H, Kim YC. Phenolic constituents of Galla Rhois with hepatoprotective effects on tacrine- and nitrofurantoin-induced cytotoxicity in Hep G2 cells. Biol Pharm Bull. 2005; 28(11):2155–2157. PMID: 16272710.


4. Song GY, Park BJ, Kim SH. Antithrombotic effect of Galla Rhois. Korean J Pharmacogn. 2002; 33(2):120–123.
5. Shim YJ, Doo HK, Ahn SY, Kim YS, Seong JK, Park IS, Min BH. Inhibitory effect of aqueous extract from the gall of Rhus chinensis on alpha-glucosidase activity and postprandial blood glucose. J Ethnopharmacol. 2003; 85(2-3):283–287. PMID: 12639753.
6. Kim SH, Park HH, Lee S, Jun CD, Choi BJ, Kim SY, Kim SH, Kim DK, Park JS, Chae BS, Shin TY. The anti-anaphylactic effect of the gall of Rhus javanica is mediated through inhibition of histamine release and inflammatory cytokine secretion. Int Immunopharmacol. 2005; 5(13-14):1820–1829. PMID: 16275618.
7. Ahn YJ, Lee CO, Kweon JH, Ahn JW, Park JH. Growth-inhibitory effects of Galla Rhois-derived tannins on intestinal bacteria. J Appl Microbiol. 1998; 84(3):439–443. PMID: 9721649.


8. Choi JG, Kang OH, Lee YS, Oh YC, Chae HS, Jang HJ, Kim JH, Sohn DH, Shin DW, Park H, Kwon DY. In vitro activity of methyl gallate isolated from galla rhois alone and in combination with ciprofloxacin against clinical isolates of salmonella. J Microbiol Biotechnol. 2008; 18(11):1848–1852. PMID: 19047831.
9. Lee JJ, Bae JH, Kim DH, Lim JJ, Kim DG, Lee HJ, Min W, Rhee MH, Chang HH, Park H, Kim S. Intracellular replication inhibitory effects of Galla Rhois ethanol extract for Brucella abortus infection. J Ethnopharmacol. 2011; 138(2):602–609. PMID: 22008879.
10. Lee JJ, Kim DH, Lim JJ, Kim DG, Min W, Kim GS, Lee HJ, Rhee MH, Park H, Kim SC, Chang HH, Kim S. Anticoccidial effect of supplemental dietary Galla Rhois against infection with Eimeria tenella in chickens. Avian Pathol. 2012; 41(4):403–407. PMID: 22834556.
11. Chae HS, Kang OH, Choi JG, Oh YC, Lee YS, Brice OO, Chong MS, Lee KN, Shin DW, Kwon DY. Methyl gallate inhibits the production of interleukin-6 and nitric oxide via down-regulation of extracellular-signal regulated protein kinase in RAW 264.7 cells. Am J Chin Med. 2010; 38(5):973–983. PMID: 20821827.


12. Park PH, Hur J, Kim YC, An RB, Sohn DH. Involvement of heme oxygenase-1 induction in inhibitory effect of ethyl gallate isolated from Galla Rhois on nitric oxide production in RAW 264.7 macrophages. Arch Pharm Res. 2011; 34(9):1545–1552. PMID: 21975817.


13. Ata N, Oku T, Hattori M, Fujii H, Nakajima M, Saiki I. Inhibition by galloylglucose (GG6-10) of tumor invasion through extracellular matrix and gelatinase-mediated degradation of type IV collagens by metastatic tumor cells. Oncol Res. 1996; 8(12):503–511. PMID: 9160354.
14. Xiang Q, Fan C, Xiao S, Pan H, Wang J, Zhao N, Tian J. Effect of gallnut extract on nasopharyngeal carcinoma 5-8F cells and its mechanism. Zhong Nan Da Xue Xue Bao Yi Xue Ban. 2012; 37(9):871–875. PMID: 23000759.
15. Lee K, Kim J, Lee BJ, Park JW, Leem KH, Bu Y. Protective effects of Galla Rhois, the excrescence produced by the sumac aphid, Schlechtendalia chinensis, on transient focal cerebral ischemia in the rat. J Insect Sci. 2012; 12:10.
16. Park EJ, Zhao YZ, An RB, Kim YC, Sohn DH. 1,2,3,4,6-penta-O-galloyl-beta-D-glucose from Galla Rhois protects primary rat hepatocytes from necrosis and apoptosis. Planta Med. 2008; 74(11):1380–1383. PMID: 18622905.
17. Lee YH. Dyeing, fastness, and deodorizing properties of cotton, silk, and wool fabrics dyed with coffee sludge (Coffea arabica L.) extract. J Appl Polym Sci. 2007; 103(1):251–257.
18. Newman DJ. Natural products as leads to potential drugs: an old process or the new hope for drug discovery? J Med Chem. 2008; 51(9):2589–2599. PMID: 18393402.


19. Efange SMN. Natural products: a continuing source of inspiration for the medical chemist. In : Iwu MM, Wootton JC, editors. Advances in phytomedicine. Amsterdam: Elsevier Science;2002.
20. Cragg GM, Newman DJ. Drug discovery and development from natural products: the way forward. 11th NAPRECA Symposium Book of Proceedings. 2005. p. 56–69.
21. Yagi K. Assay for blood plasma or serum. Methods Enzymol. 1984; 105:328–331. PMID: 6727672.
22. Cheeseman KH, Slater TF. An introduction to free radical biochemistry. Br Med Bull. 1993; 49(3):481–493. PMID: 8221017.


24. Mohamed EI, Elazomi A, Elabid BEH, Zwaik H. Evaluation of changes in levels of plasma MDA, and antioxidant vitamin E in Sudanese patients with type 2 diabetes. In : International Conference on Chemical, Environment & Biological Sciences; 2014. p. 17–18.
25. Barrera G. Oxidative stress and lipid peroxidation products in cancer progression and therapy. ISRN Oncol. 2012; 2012:137289. PMID: 23119185.


26. Montine TJ, Neely MD, Quinn JF, Beal MF, Markesbery WR, Roberts LJ, Morrow JD. Lipid peroxidation in aging brain and Alzheimer's disease. Free Radic Biol Med. 2002; 33(5):620–626. PMID: 12208348.
27. Dahanukar SA, Kulkarni RA, Rege NN. Pharmacology of medicinal plants and natural products. Indian J Pharmacol. 2000; 32:S81–S118.
28. Dragoev SG. Inhibition of lipid peroxidation of frozen mackerel by pre-storage antioxidant superficial treatment. Bul J Agri Sci. 2008; 14(3):283–289.
29. Ng TB, Liu F, Wang ZT. Antioxidative activity of natural products from plants. Life Sci. 2000; 66(8):709–723. PMID: 10680579.


30. Chen CH, Liu TZ, Chen CH, Wong CH, Chen CH, Lu FJ, Chen SC. The efficacy of protective effects of tannic acid, gallic acid, ellagic acid, and propyl gallate against hydrogen peroxide-induced oxidative stress and DNA damages in IMR-90 cells. Mol Nutr Food Res. 2007; 51(8):962–968. PMID: 17628875.


31. Nabavi SF, Habtemariam S, Sureda A, Hajizadeh Moghaddam A, Daglia M, Nabavi SM. In vivo protective effects of gallic acid isolated from Peltiphyllum peltatum against sodium fluoride-induced oxidative stress in rat erythrocytes. Arh Hig Rada Toksikol. 2013; 64(4):553–559. PMID: 24384762.
32. Laffel L. Ketone bodies: a review of physiology, pathophysiology and application of monitoring to diabetes. Diabetes Metab Res Rev. 1999; 15(6):412–426. PMID: 10634967.


33. KREBS HA. The physiological role of the ketone bodies. Biochem J. 1961; 80:225–233. PMID: 13754174.


34. VanItallie TB, Nufert TH. Ketones: metabolism's ugly duckling. Nutr Rev. 2003; 61(10):327–341. PMID: 14604265.


35. Ferrell KE, Thorington RW. Squirrels: the animal answer guide. Johns Hopkins University Press;2006. p. 91.
36. Muller-Harvey I, McAllan AB. Tannins: Their biochemistry and nutritional properties. Adv Plant Cell Biochem Biotechnol. 1992; 1:151–217.
37. Dollahite JW, Pigeon RF, Camp BJ. The toxicity of gallic acid, pyrogallol, tannic acid, and Quercus havardi in the rabbit. Am J Vet Res. 1962; 23(97):1264–1267. PMID: 14028469.
38. Boyd EM, Bereczky K, Godi I. The acute toxicity of tannic acid administered intragastrically. Can Med Assoc J. 1965; 92(25):1292–1297. PMID: 14291458.
39. Toxicological Evaluation of some extraction solvents and certain other substances. FAO Nutrition Meetings Series, No. 48A. 1970.
40. Inert Reassessmen-t Tannin (CAS Reg. No. 1401-55-4). Office of prevention, pesticides and toxic substances, US Environmental Protection Agency;2006.
41. Eaton GJ, Johnson FN, Custer RP, Crane AR. The Icr:Ha(ICR) mouse: a current account of breeding, mutations, diseases and mortality. Lab Anim. 1980; 14(1):17–24. PMID: 7359882.


43. Takeno S, Hirano Y, Kitamura A, Sakai T. Comparative developmental toxicity and metabolism of nitrazepam in rats and mice. Toxicol Appl Pharmacol. 1993; 121(2):233–238. PMID: 8346540.


44. Xu X, Zhang L, Shao B, Sun X, Ho CT, Li S. Safety evaluation of meso-zeaxanthin. Food Control. 2013; 32(2):678–686.


45. Zhang Y, Li J, Wu Z, Liu E, Shi P, Han L, Guo L, Gao X, Wang T. Acute and long-term toxicity of mango leaves extract in mice and rats. Evid Based Complement Alternat Med. 2014; 2014:691574. PMID: 25246938.


46. Xiang F, Peng L, Yin Z, Jia R, Hu Z, Li Z, Ni X, Liang X, Li L, He C, Yin L, Su G, Lv C. Acute and subchronic toxicity as well as evaluation of safety pharmacology of Galla chinensis solution. J Ethnopharmacol. 2015; 162:181–190. PMID: 25540924.
47. Bissell DM, Gores GJ, Laskin DL, Hoofnagle JH. Drug-induced liver injury: mechanisms and test systems. Hepatology. 2001; 33(4):1009–1013. PMID: 11283870.


48. Kleiner DE, Brunt EM, Van Natta M, Behling C, Contos MJ, Cummings OW, Ferrell LD, Liu YC, Torbenson MS, Unalp-Arida A, Yeh M, McCullough AJ, Sanyal AJ. Nonalcoholic Steatohepatitis clinical research network. Design and validation of a histological scoring system for nonalcoholic fatty liver disease. Hepatology. 2005; 41(6):1313–1321. PMID: 15915461.


49. Giannini E, Botta F, Fasoli A, Ceppa P, Risso D, Lantieri PB, Celle G, Testa R. Progressive liver functional impairment is associated with an increase in AST/ALT ratio. Dig Dis Sci. 1999; 44(6):1249–1253. PMID: 10389705.
50. Sheth SG, Flamm SL, Gordon FD, Chopra S. AST/ALT ratio predicts cirrhosis in patients with chronic hepatitis C virus infection. Am J Gastroenterol. 1998; 93(1):44–48. PMID: 9448172.


51. Yoon SH, Park EJ, Oh KH, Chung YG, Kwon OJ. The effect of Lithospermi radix on benzo (a) pyrene-induced hepatotoxicity. J Korean Soc Food Sci Nutr. 1993; 22(2):144–148.
52. Hayes AW. Principles and methods of toxicology. New York: Raven Press;1982. p. 447–474.
53. Kim DH, Deung YK, Lee YM, Yoon YS, Kwon KR, Park DB, Park YK, Lee KJ. The liver protecting effect of pomegranate (Punica granatum) seed oil in mice treated with CCl4. Korean J Electron Microscopy. 2006; 36(3):173–182.
54. Michael B, Yano B, Sellers RS, Perry R, Morton D, Roome N, Johnson JK, Schafer K, Pitsch S. Evaluation of organ weights for rodent and non-rodent toxicity studies: a review of regulatory guidelines and a survey of current practices. Toxicol Pathol. 2007; 35(5):742–750. PMID: 17849357.


55. Singhal PC, Sharma P, Sanwal V, Prasad A, Kapasi A, Ranjan R, Franki N, Reddy K, Gibbons N. Morphine modulates proliferation of kidney fibroblasts. Kidney Int. 198; 53(2):350–357. PMID: 9461094.


56. Falconer IR, Hardy SJ, Humpage AR, Froscio SM, Tozer GJ, Hawkins PR. Hepatic and renal toxicity of the blue-green alga (cyanobacterium) Cylindrospermopsis raciborskii in male Swiss albino mice. Environ Toxicol. 1999; 14(1):143–150.
57. Horiguchi H, Oguma E, Kayama F, Sato M, Fukushima M. Dexamethasone prevents acute cadmium-induced hepatic injury but exacerbates kidney dysfunction in rabbits. Toxicol Appl Pharmacol. 2001; 174(3):225–234. PMID: 11485383.


58. Bonventre JV, Vaidya VS, Schmouder R, Feig P, Dieterle F. Next-generation biomarkers for detecting kidney toxicity. Nat Biotechnol. 2010; 28(5):436–440. PMID: 20458311.


Figure 1
MDA concentration in the serum of ICR mice treated with GEGR. The level of MDA was determined in the serum collected from the whole blood of ICR mice using a lipid peroxidation assay kit. Also, this kit could detect MDA at 0.1 nmole/mg to 20 nmole nmole/mg. Three samples were assayed in triplicate by MDA assay. Data are reported as the mean±SD. a, P<0.05 compared to the vehicle-treated group.
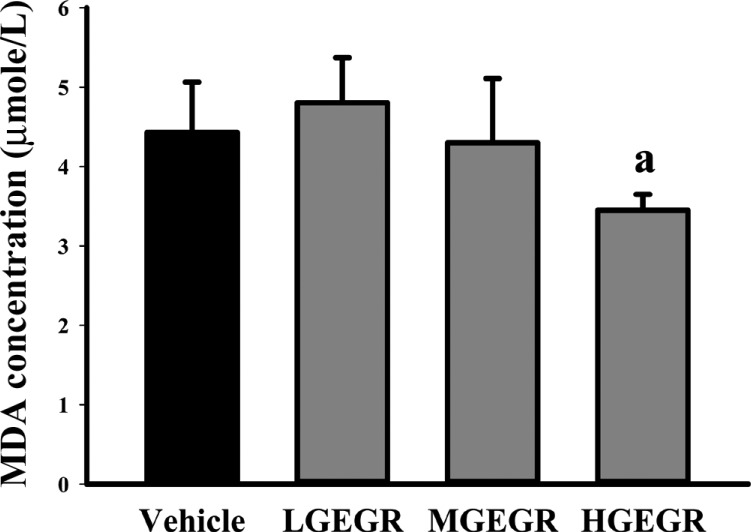
Figure 2
Effects of GEGR on liver toxicity in ICR mice. (A) Serum was collected from the abdominal veins of ICR mice and the serum concentrations of ALP, AST, ALT and LDH were then analyzed as described in the Materials and Methods. (B) Liver tissues were stained with H&E and cellular morphology was viewed at 400× magnification. The data shown represent the means±SD of three replicates. Vehicle, vehicle-treated group; LGEGR, low dosage GEGR-treated group; MGEGR, middle dosage GEGR-treated group; HGEGR, high dosage GEGR-treated group. Arrow, hepatocytes; arrow head, Sinusoid.
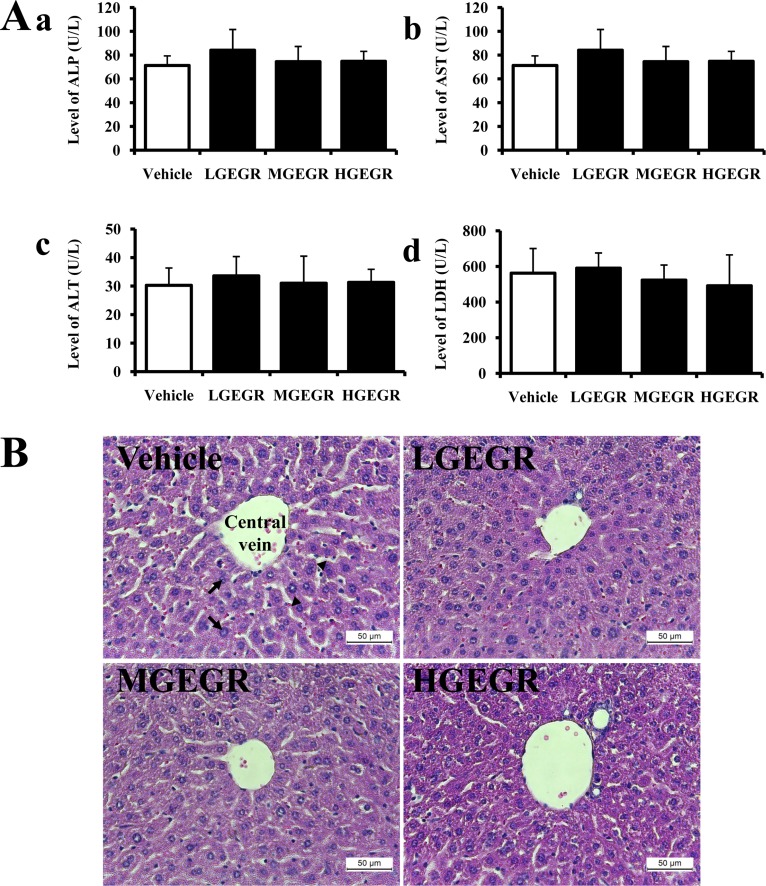
Figure 3
Effects of GEGR on the kidney toxicity in ICR mice. (A) Serum was collected from the abdominal veins of ICR mice and serum concentrations of BUN and CRE were then analyzed as described in the Materials and Methods. (B) Kidney tissues were stained with H&E, and cellular morphology was viewed at 400× magnification. The data shown represent the means±SD of three replicates. Vehicle, vehicle-treated group; LGEGR, low dosage GEGR-treated group; MGEGR, middle dosage GEGR-treated group; HGEGR, high dosage GEGR-treated group. Arrow, Distal convoluted tubules; arrow head, Proximal convoluted tubules; RC, Renal corpuscle.
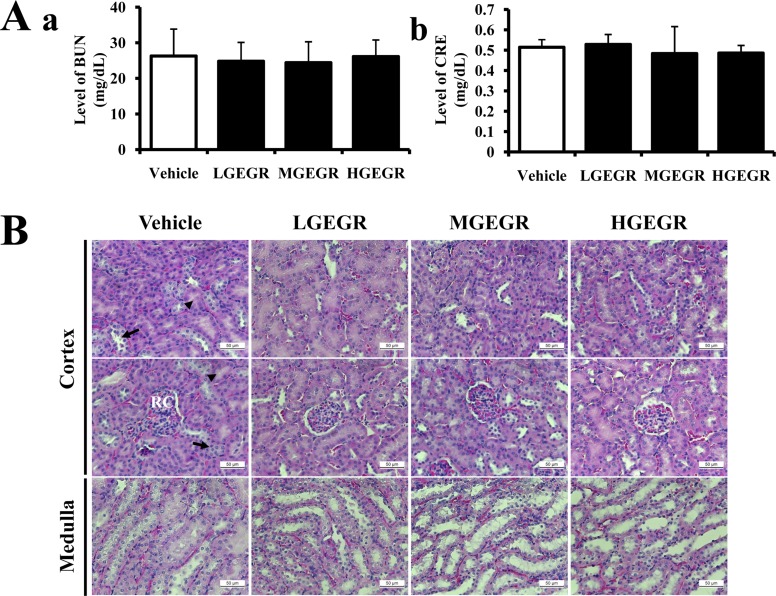
Table 1
Distribution of three functional components in GEGR
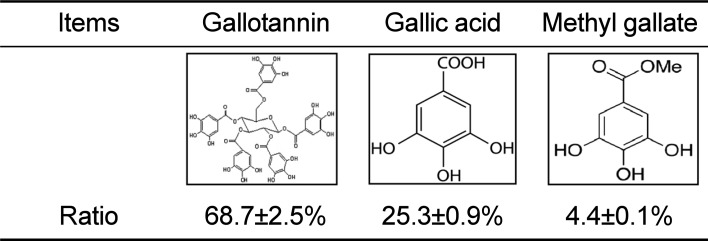
Items | Gallotannin | Gallic acid | Methyl gallate |
---|---|---|---|
![]() |
![]() |
![]() |
|
Ratio | 68.7±2.5% | 25.3±0.9% | 4.4±0.1% |
Table 2
Alteration in the weights of body and organs of ICR mice treated with GEGR
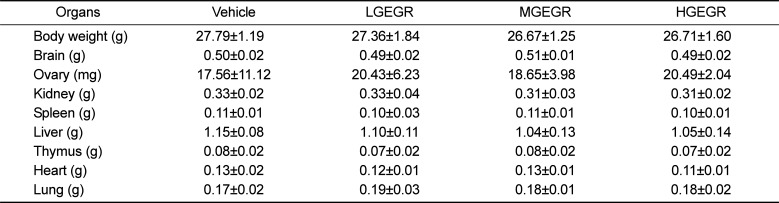
Table 3
Alteration in the urine biochemical parameters of ICR mice treated with GEGR
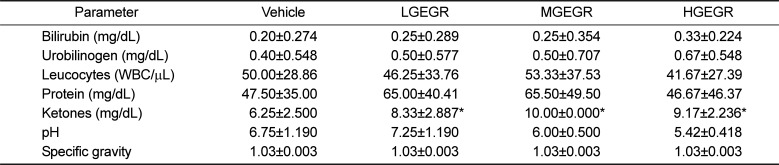