Abstract
Red ginseng and its extracts have been used as traditional medicines and functional foods in countries worldwide. The aim of this study was to examine the bioavailability of pectin lyase-modified red ginseng extracts (GS-E3D), and the effects of GS-E3D on adipogenesis of 3T3-L1 adipocytes, as well as on metabolic disorders such as hyperglycemia, dyslipidemia, and fatty liver in high-fat diet fed obese C57BL/6 mice. Mice were divided into 5 groups: normal diet group, high fat diet-vehicle group, high fat diet + 0.1 g/kg GS-E3D (0.1-GS-E3D), high fat diet + 0.3 g/kg (0.3-GS-E3D), high fat diet + 1.0 g/kg (1.0-GS-E3D). Treatment of GS-E3D reduced differentiation of 3T3-L1 adipocytes with low cytotoxicity. In the animal model, compared to the high fat diet control, serum glucose, total cholesterol, LDL-cholesterol, HDL-cholesterol, TG, and leptin level were reduced in treatment animals in a dose-dependent manner. In addition, we found that GS-E3D could decrease total hepatic lipid droplets. These results suggest that GS-E3D, as a dietary supplement, has beneficial effects on obesity and may have useful effects in health-care products.
Ginseng, the root of Panax ginseng C.A. Mayer, and its preparation, red ginseng, are common traditional medicines in Asian countries such as Korea, China, and Japan [1,2]. Red ginseng, which contains many functional chemicals and complexes, is manufactured through techniques utilizing repetitive steaming and drying cycles [3]. This processing results in the formation of additional beneficial compounds, known as ginsenosides, with various pharmacological effects [4] on immune response [5], diabetes [6,7], and Alzheimer's disease [8,9]. The ginsenosides of Panax ginseng are a group of steroidal saponins; over 50 of the compounds have been identified [10], which are generally divided into 2 classes: the protopanaxadiols, with study compounds Rb1, Rb2, Rb3, 20(S)-Rg3, 20(R)-Rg3, and Rd; and the protopanaxatriols, with study compounds Rg1, Re, Rf, Rg5, and Rk1.
Metabolic disorders, including obesity, are chronic worldwide, leading to various detrimental conditions in adults, such as hyperglycemia, dyslipidemia, and hypertension [11,12]. In particular, excessive dietary fatty acids and genetic causes of obesity induce increases in triglyceride (TG) accumulation in fat tissue and the reuptake of free fatty acids into the circulatory system, which contributes to TG accumulation in the liver through fatty acid binding and the action of transport proteins [13,14,15]. Effective control of blood glucose and lipid levels is necessary to regulate or prevent complications such as hyperglycemia and fatty liver [16,17].
Several studies have reported effects of fermentation and enzyme processing on ginseng (including red ginseng) and the development of ginsenosides [3,18,19,20]. Studies have also examined the effects of the resulting ginsenosides in various disease models such as metabolic disorders [21,22,23,24,25], cancer [26,27], immune response [1,28], skin care [29,30], pulmonary system [31], brain injury [32], depression [33], and Alzheimer's disease [34] in both in vitro and in vivo models [35]. Although many pharmaceutical effects of red ginseng and its fermented products have been postulated, the full range of applications of purified enzymes still remains unclear. Indeed, to date, the effects of commercial pectin lyase-modified red ginseng extracts on high fat diet-fed obese mice have not been reported.
Microbial pectin lyase is commonly used for its beneficial effect on various plant products such as carrot [36], and date syrup [37] in the production of functional compounds, but it has not been fully applied to ginseng. In this study, we enzyme-modified red ginseng extracts with microbial pectin lyase and observed its bioavailability in and effects on high fat diet-fed obese mice.
Dexamethasone, 3-isobutyl-1-methylxanthine (IBMX), insulin, formaldehyde, and Oil Red O solution were purchased from Sigma-Aldrich (MO, USA). Cell culture media, fetal bovine serum, and supplements were obtained from Thermo Scientific (UT, USA)
A dried mixture of whole red ginseng and red ginseng tail root (4:6 w/w) was extracted 3 times with 70% ethanol. The extracts were then concentrated to 6 Brix with a vacuum evaporator at 40℃. The extracts were incubated with 10% pectin lyase (EC 4.2.2.10, Novozyme, #33095, Bagsvaerd, Denmark), which was prepared from Aspergillus aculeatus and Aspergillus niger cultivates, at 50℃ for 5 days in a shaking incubator (150 rpm). To terminate the reaction, processed extracts were heated at 95℃ for 10 min, and then freeze-dried. The dried GS-E3D consisted of 120.2 mg/g crude saponin containing the following ginsenosides: 5.9 mg/g Rg1, 30.2 mg/g Rb1, 17.6 mg/g Rb2, 2.5 mg/g Rb3, 1.3 mg/g 20(S)-Rg3, 1.4 mg/g 20(R)-Rg3, 27.7 mg/g Rd, 12.6 mg/g Re, 1.5 mg/g Rg5, 0.8 mg/g Rk1, and 4.7 mg/g Rf (International Ginseng and Herb Research Institute, Geumsan, Korea).
To induce adipogenesis, 3T3-L1 preadipocytes were seeded into a 6-well plate at 2×105 cells per well and cultured until fully confluent. After 2 days in the medium, cells were incubated with a differentiation medium containing 1 µg/mL insulin, 1 µM dexamethasone, and 0.5 mM IBMX in standard culture medium for 2 days, followed by additional 48 h with standard culture medium containing insulin alone. The differentiation-maintenance medium was changed every 2 days. To investigate the effect of GS-E3D on differentiation in 3T3-L1, GS-E3D (at 0.1, 0.5, 1.0, 2.0, and 5.0 mg/mL) was added to the differentiation-induction medium. The assay protocol was conducted according to the colorimetric adipogenesis assay kit (Cayman Chemical Company, MI, USA). The absorbance was measured at 500 nm using a spectrophotometer (Infinite 200, Tecan Co, Männedorf, Switzerland) with 96-well plates. Lipid contents were calculated as a percent of control cells.
Specific-pathogen-free 6-week-old male C57BL/6J mice (18-23 g) were purchased from SAMTAKO Co. Ltd. (Osan, South Korea). The experiments were performed in accordance with the principles of and approval from the Ethics Committee of the Wonkwang University, Iksan, Korea (WKU12-47). All animals, which were maintained in a temperature-controlled room (temperature 22±2℃, humidity 50±5%) with a 12/12 h light/dark cycle, were acclimatized to the laboratory environment while housed in individual cages for 1 week before the experiment. Obesity was induced by a 60% fat calorie diet (high fat diet, or HFD), and mice were randomly divided into 5 groups (10 mice per group) after the first 3 weeks on high fat diet (AIN-93G, cat., #101556; Research Diet, Inc., NJ, USA). The study groups included: the normal diet group; the high fat diet-vehicle group; high fat diet+0.1 g/kg GS-E3D (0.1-GS-E3D); high fat diet+0.3 g/kg GS-E3D (0.3-GS-E3D); and high fat diet+1.0 g/kg GS-E3D (1.0-GS-E3D). Depending on the assigned group, placebo (water) or 3 doses of GS-E3D (0.1, 0.3, or 1.0 g/kg) were administered orally, twice daily for 4 weeks. At the end of the experiment, 4 h-fasted mice were anaesthetized using a mixture of xylazine/ketamine (1:3, v/v), and blood samples were collected and stored at -80℃ until their analyses (Figure 1).
After 4 weeks of treatment with and without GS-E3D, blood samples were collected and centrifuged at 3,000 rpm for 15 min at 4℃, and then levels of serum glucose, total cholesterol (T-CHO), high-density lipoprotein cholesterol (HDL-C), low-density lipoprotein cholesterol (LDL-C), triglyceride (TG), and leptin were measured by Green Cross Reference Lab (Yongin, Korea).
Body weight was measured once a week during the feeding period. Internal organs were dissected and weighed. All body, organ and fat was weighed to the nearest 0.01 g using an electronic balance (Sartorius, Göttingen, Germany). Fat tissue samples also were dissected, weighed, and then processed for further histological experiments.
Histological analysis was conducted following routine methods. Briefly, the dissected epididymal adipose tissues were fixed in 10% neutral buffered formalin for histological analysis and embedded in paraffin. The paraffin sections were cut at a thickness of 4 µm and stained with hematoxylin and eosin (H&E). Adipocyte sizes were measured in randomly chosen microscopic areas from individual animals using a Nikon microscope system (TE-2000, Tokyo, Japan). Average adipocyte size was calculated by dividing the each chosen microscopic area by total adipocyte cells in the area [38].
All data were expressed as mean±standard error of the mean (SEM), and differences between groups were analyzed using one-way ANOVA (Duncan's multiple-range test). The effects of GS-E3D on body weight, food intake and water intake in experimental period were compared by two-way repeated measures analysis of variance (ANOVA) with Bonferroni's post-test analysis. All analyses were performed using SPSS 10.0 (SPSS Inc. USA). Each value was the mean of at least 3 separate experiments in each group. Statistical significance was set at P<0.05.
To determine the cytotoxic effects of GS-E3D on 3T3-L1 adipocyte cells, we performed a tetrazolium (MTT) assay. Treatment of GS-E3D did not inhibit 3T3-L1 cell viability at 5 mg/mL (>95%), but exposure of 10 mg/mL GS-E3D to cells showed high growth inhibition at 48 h (approximately<40%) (Figure 2). Therefore, we did not use a dose of 10 mg/mL GS-E3D for further experiments.
A 3T3-L1 differentiation assay was performed to investigate the effect of GS-E3D in 3T3-L1 cells using an Oil Red O staining-based assay kit (Cayman Chemical Company, MI, USA). Differentiated 3T3-L1 cells were treated every 48 h with GS-E3D at the indicated concentration, for 5 days. Based on quantification of lipid content, results showed that GS-E3D treatment at 0.1, 0.5, 1.0, 2.0 and 5.0 mg/mL in 3T3-L1 cells could inhibit lipid accumulation, as compared to untreated cells, in a dose-dependent manner (Figure 3). At 5.0 mg/mL, GS-E3D treatment showed a maximum inhibitory effect without cytotoxicity (Figure 2). These results suggest that GS-E3D may reduce adipogenesis and lipid accumulation in fat tissues.
The effects of GS-E3D on body weights are shown in Figure 4A. After 4 weeks of treatment with GS-E3D, the body weight of the high fat diet-vehicle group was significantly higher than that of the normal group. In contrast, the body weights of the GS-E3D groups were significantly lower, compared to the high fat diet-vehicle group. Although the body weight was significantly reduced in the GS-E3D group at 4 weeks after supplementation, there were no significant observed differences in body weight from 1 to 3 weeks. However, food intake and water intake did not significantly differ between each group (Figures 4B and C).
Figure 5 shows the effects of GS-E3D on blood glucose levels after 4 weeks. Blood glucose levels were measured at 4 weeks after twice-daily administration. Compared to the normal group, the high fat diet group had higher blood glucose levels. However, there were no significant changes in glucose levels by GS-E3D treatment.
Lipid levels (T-CHO, LDL-C, HDL-C, and TG) of experimental mice were analyzed (Figure 6). The control (high fat diet-fed mice) group showed high levels of T-CHO, LDL-C, and HDL-C when compared to the regular chow diet group (Figure 6A-C), but no significant increase was observed in TG concentration (Figure 6D). As shown Figure 6, T-CHO, LDL-C, HDL-C, and TG levels were significantly reduced in the GS-E3D 0.5 and GS-E3D 1.0 groups, compared to the control group. These results suggest that GS-E3D improved blood lipid profiles.
The results of leptin levels are shown in Figure 7. The serum leptin levels were significantly elevated in high fat diet-induced obese mice. In contrast, oral administration of GS-E3D significantly reduced serum leptin levels in a dose-dependent manner, compared to the control group.
As shown in Figure 8A-C, fat weights were significantly higher in the high fat diet-induced obese mice group than in the normal group. However, there were no significant differences in the fat weights between the control and GS-E3D groups. Although there was no effect of GS-E3D on fat weights, administration of GS-E3D reduced liver weight overall (Figure 8D). The liver weight of high fat diet-induced obese mice was significantly higher than that of the normal group. In contrast, the GS-E3D 1.0 group showed significantly reduced liver weight; however, the GS-E3D 0.1 and GS-E3D 0.3 groups showed no significant differences.
Figure 9 shows the effects on organ weight for each group. The organ weights were measured at 4 weeks. Significant increases in the weights of spleen and thymus were observed, but the weights of testis and kidney decreased on the high fat diet. In contrast, the administration of GS-E3Ds reduced the weights of spleen and increased the weight of testis and kidney in high fat diet-induced obese mice. Although the weight of thymus in both the control and GS-E3D groups were higher than the normal group, these phenomena were induced through reduction of immune function [39,40]. These results suggest that GS-E3D regulates the innate function of organs involved in metabolism and immunity.
The effects of GS-E3D on changes in adipocyte size in high fat diet obese mice are shown in Figure 10. High fat diet obese mice showed significantly larger adipocytes from epididymal adipose tissue (Figure 10B), but administration of GS-E3D markedly reduced the size of adipocytes, in a dose-dependent manner.
To determine ameliorative effects of GS-E3D on high fat diet-induced hepatic steatosis, we observed lipid droplet contents through an H&E stain in liver after 4 weeks (Figure 11). High fat diet mice had significantly increased steatosis scores, but administration of F-BS reduced the number of lipid droplets in a dose-dependent manner (Figure 11F).
Ginseng has been widely recognized for several thousand years as a valuable plant and useful medicine in the Asia-Pacific region, and in many countries such as Korea, China, Japan, and even Canada [7,25]. Typically, orally-administrated ginseng is broken down into various molecules, including ginsenosides, through exposure to gastric juices, digestive enzymes, and intestinal bacteria. Consequently, the absorption efficacy of ginseng's components may differ due to the varying resident microenvironments in the individuals consuming it [41]. Several groups have examined microflora in conjunction with enzymes in several studies involving red ginseng, with the aim of increasing efficacy [22,34,35,26,30,32]. Similarly, GS-E3D is produced from red ginseng extracts that were enzymatically modified with commercial pectin lyase (EC 4.2.2.10); however, in this study, we use tail root as a source of red ginseng and do not report on these methods.
Our aim was to investigate the effects and bioavailability of GS-E3D on high fat diet-fed obese mice. In addition, to develop a novel processing procedure of red ginseng extracts, we increased the amount of red ginseng tail root to exceed the amount of whole red ginseng (>1.5 fold, w/w) in extracts, as tail root contains more ginsenosides in red ginseng. In other hands, we should compared the effects of red ginseng extracts with G3-E3D but not observed significant differences between both extracts in experimental model as expected, unfortunately. However, GS-E3D and its processing procedures will helpful for absorption and utilization of effective molecules of red ginseng in other models.
We also observed ameliorative effects of GS-E3D on hepatic steatosis (Figure 11) and demonstrated histologic data with statistics. GS-E3D was significantly reduced lipid droplets in liver tissues but not recovered completely other phenomena such as observe of eosinophilic cytoplasm in H&E stained tissue sections. Indeed, lower food intake were observed in normal diet group at 3 weeks after first administration of GS-E3D or control, but it was unidentifiable things at the specific time couse. Therefore, these apparent symptom must be recognized and need further study.
In the present study, we found beneficial effects of GS-E3D for high fat diet-fed obese mice. Administration of GS-E3D resulted in improved blood glucose levels, lipid profiles, and leptin levels in high fat diet-fed obese mice. Moreover, GS-E3D reversed the progression of fatty liver by high fat diet-fed mice in the same animal model. Indeed, GS-E3D administration was found to be effective in reducing adipogenesis and lipid metabolism in high fat diet-induced obesity and liver steatosis models. The treatment decreased adipocyte differentiation, and ameliorated blood lipid parameters during a 4-week trial. Treatment significantly reduced the size of epididymal adipose tissue and conclusively ameliorated high fat diet-induced hepatic steatosis. These results suggest that GS-E3D as a dietary supplement may have beneficial effects on obese individuals, and may be useful as a health-care product. In the future, studies on the ginsenoside composition and the detailed mechanisms of processing techniques of red ginseng are required.
Acknowledgments
This work was supported in part by the "Food Functionality Evaluation Program" under the Ministry of Food, Agriculture, Forestry and Fisheries of South Korea.
References
1. Lee EJ, Song MJ, Kwon HS, Ji GE, Sung MK. Oral administration of fermented red ginseng suppressed ovalbumin-induced allergic responses in female BALB/c mice. Phytomedicine. 2012; 19(10):896–903. PMID: 22608480.


2. Lu JM, Yao Q, Chen C. Ginseng compounds: an update on their molecular mechanisms and medical applications. Curr Vasc Pharmacol. 2009; 7(3):293–302. PMID: 19601854.


3. Hong SY, Oh JH, Lee I. Simultaneous enrichment of deglycosylated ginsenosides and monacolin K in red ginseng by fermentation with Monascus pilosus. Biosci Biotechnol Biochem. 2011; 75(8):1490–1495. PMID: 21821946.
4. Shibata S. Chemistry and cancer preventing activities of ginseng saponins and some related triterpenoid compounds. J Korean Med Sci. 2001; 16:S28–S37. PMID: 11748374.


5. Park KH, Kim YS, Jeong JH. Inhibitory effects of ginseng extracts on histamine-release from rat's mast cell. Korean J Plant Res. 2011; 24(1):98–104.


6. Reeds DN, Patterson BW, Okunade A, Holloszy JO, Polonsky KS, Klein S. Ginseng and ginsenoside Re do not improve β-cell function or insulin sensitivity in overweight and obese subjects with impaired glucose tolerance or diabetes. Diabetes Care. 2011; 34(5):1071–1076. PMID: 21411505.


7. Attele AS, Zhou YP, Xie JT, Wu JA, Zhang L, Dey L, Pugh W, Rue PA, Polonsky KS, Yuan CS. Antidiabetic effects of Panax ginseng berry extract and the identification of an effective component. Diabetes. 2002; 51(6):1851–1858. PMID: 12031973.
8. Chen F, Eckman EA, Eckman CB. Reductions in levels of the Alzheimer\'s amyloid beta peptide after oral administration of ginsenosides. FASEB J. 2006; 20(8):1269–1271. PMID: 16636099.
9. Shieh PC, Tsao CW, Li JS, Wu HT, Wen YJ, Kou DH, Cheng JT. Role of pituitary adenylate cyclase-activating polypeptide (PACAP) in the action of ginsenoside Rh2 against beta-amyloid-induced inhibition of rat brain astrocytes. Neurosci Lett. 2008; 434(1):1–5. PMID: 18313848.


10. Tanaka O, Kasai R. Saponins of ginseng and related plants. Fortschr Chem Org Naturst. 1984; 46:1–76. PMID: 6396179.


11. Glenny AM, O'Meara S, Melville A, Sheldon TA, Wilson C. The treatment and prevention of obesity: a systematic review of the literature. Int J Obes Relat Metab Disord. 1997; 21(9):715–737. PMID: 9376884.
12. Keck PE, McElroy SL. Bipolar disorder, obesity, and pharmacotherapy-associated weight gain. J Clin Psychiatry. 2003; 64(12):1426–1435. PMID: 14728103.


13. Atshaves BP, Martin GG, Hostetler HA, McIntosh AL, Kier AB, Schroeder F. Liver fatty acid-binding protein and obesity. J Nutr Biochem. 2010; 21(11):1015–1032. PMID: 20537520.


14. Farrell GC, Larter CZ. Nonalcoholic fatty liver disease: from steatosis to cirrhosis. Hepatology. 2006; 43:S99–S112. PMID: 16447287.


15. Park EJ, Lee JH, Yu GY, He G, Ali SR, Holzer RG, Osterreicher CH, Takahashi H, Karin M. Dietary and genetic obesity promote liver inflammation and tumorigenesis by enhancing IL-6 and TNF expression. Cell. 2010; 140(2):197–208. PMID: 20141834.


16. Warren RE. The stepwise approach to the management of type 2 diabetes. Diabetes Res Clin Pract. 2004; 65(Suppl 1):S3–S8. PMID: 15315864.


17. Fletcher B, Berra K, Ades P, Braun LT, Burke LE, Durstine JL, Fair JM, Fletcher GF, Goff D, Hayman LL, Hiatt WR, Miller NH, Krauss R, Kris-Etherton P, Stone N, Wilterdink J, Winston M. Council on Cardiovascular Nursing. Council on Arteriosclerosis, Thrombosis, and Vascular Biology. Council on Basic Cardiovascular Sciences. Council on Cardiovascular Disease in the Young. Council on Clinical Cardiology. Council on Epidemiology and Prevention. Council on Nutrition, Physical Activity, and Metabolism. Council on Stroke. Preventive Cardiovascular Nurses Association. Managing abnormal blood lipids: a collaborative approach. Circulation. 2005; 112(20):3184–3209. PMID: 16286609.
18. Ryu JS, Lee HJ, Bae SH, Kim SY, Park Y, Suh HJ, Jeong YH. The bioavailability of red ginseng extract fermented by Phellinus linteus. J Ginseng Res. 2013; 37(1):108–116. PMID: 23717164.


19. Sunwoo HH, Kim CT, Kim DY, Maeng JS, Cho CW, Lee SJ. Extraction of ginsenosides from fresh ginseng roots (Panax ginseng C.A. Meyer) using commercial enzymes and high hydrostatic pressure. Biotechnol Lett. 2013; 35(7):1017–1022. PMID: 23515895.
20. Quan LH, Min JW, Jin Y, Wang C, Kim YJ, Yang DC. Enzymatic biotransformation of ginsenoside Rb1 to compound K by recombinant β-glucosidase from Microbacterium esteraromaticum. J Agric Food Chem. 2012; 60(14):3776–3781. PMID: 22428991.


21. Kim SN, Lee JH, Shin H, Son SH, Kim YS. Effects of in vitro-digested ginsenosides on lipid accumulation in 3T3-L1 adipocytes. Planta Med. 2009; 75(6):596–601. PMID: 19204893.
22. Kim CM, Yi SJ, Cho IJ, Ku SK. Red-koji fermented red ginseng ameliorates high fat diet-induced metabolic disorders in mice. Nutrients. 2013; 5(11):4316–4332. PMID: 24177708.
23. Lee KJ, Lee SY, Ji GE. Diabetes-ameliorating effects of fermented red ginseng and causal effects on hormonal interactions: testing the hypothesis by multiple group path analysis. J Med Food. 2013; 16(5):383–395. PMID: 23675990.


24. Oh JS, Lee SR, Hwang KT, Ji GE. The anti-obesity effects of the dietary combination of fermented red ginseng with levan in high fat diet mouse model. Phytother Res. 2014; 28(4):617–622. PMID: 23873605.


25. Yuan HD, Quan HY, Jung MS, Kim SJ, Huang B, Kim do Y, Chung SH. Anti-Diabetic Effect of Pectinase-Processed Ginseng Radix (GINST) in High Fat Diet-Fed ICR Mice. J Ginseng Res. 2011; 35(3):308–314. PMID: 23717074.


26. Fu Y, Yin Z, Wu L, Yin C. Fermentation of ginseng extracts by Penicillium simplicissimum GS33 and anti-ovarian cancer activity of fermented products. World J Microbiol Biotechnol. 2014; 30(3):1019–1025. PMID: 24122127.


27. Jang SI, Lee YW, Cho CK, Yoo HS, Jang JH. Identification of Target Genes Involved in the Antiproliferative Effect of Enzyme-Modified Ginseng Extract in HepG2 Hepatocarcinoma Cell. Evid Based Complement Alternat Med. 2013; 2013:502568. PMID: 24174975.


28. Seo JY, Lee JH, Kim NW, Kim YJ, Chang SH, Ko NY, Her E, Yoo YH, Kim JW, Lee BY, Lee HY, Kim YM, Choi WS. Inhibitory effects of a fermented ginseng extract, BST204, on the expression of inducible nitric oxide synthase and nitric oxide production in lipopolysaccharide-activated murine macrophages. J Pharm Pharmacol. 2005; 57(7):911–918. PMID: 15969952.


29. Lee HS, Kim MR, Park Y, Park HJ, Chang UJ, Kim SY, Suh HJ. Fermenting red ginseng enhances its safety and efficacy as a novel skin care anti-aging ingredient: in vitro and animal study. J Med Food. 2012; 15(11):1015–1023. PMID: 23126662.


30. Hwang E, Sun ZW, Lee TH, Shin HS, Park SY, Lee DG, Cho BG, Sohn H, Kwon OW, Kim SY, Yi TH. Enzyme-processed Korean Red Ginseng extracts protects against skin damage induced by UVB irradiation in hairless mice. J Ginseng Res. 2013; 37(4):425–434. PMID: 24233239.


31. Rimar S, Lee-Mengel M, Gillis CN. Pulmonary protective and vasodilator effects of a standardized Panax ginseng preparation following artificial gastric digestion. Pulm Pharmacol. 1996; 9(4):205–209. PMID: 9160407.
32. Bae EA, Hyun YJ, Choo MK, Oh JK, Ryu JH, Kim DH. Protective effect of fermented red ginseng on a transient focal ischemic rats. Arch Pharm Res. 2004; 27(11):1136–1140. PMID: 15595417.


33. Lee KJ, Ji GE. The effect of fermented red ginseng on depression is mediated by lipids. Nutr Neurosci. 2014; 17(1):7–15. PMID: 24088416.


34. Kim J, Kim SH, Lee DS, Lee DJ, Kim SH, Chung S, Yang HO. Effects of fermented ginseng on memory impairment and β-amyloid reduction in Alzheimer's disease experimental models. J Ginseng Res. 2013; 37(1):100–107. PMID: 23717163.


35. Jin H, Seo JH, Uhm YK, Jung CY, Lee SK, Yim SV. Pharmacokinetic comparison of ginsenoside metabolite IH-901 from fermented and non-fermented ginseng in healthy Korean volunteers. J Ethnopharmacol. 2012; 139(2):664–667. PMID: 22178175.


36. Yu LJ, Rupasinghe HP. Improvement of cloud stability, yield and β-carotene content of carrot juice by process modification. Food Sci Technol Int. 2013; 19(5):399–406. PMID: 23729426.


37. Abbès F, Besbes S, Brahim B, Kchaou W, Attia H, Blecker C. Effect of concentration temperature on some bioactive compounds and antioxidant proprieties of date syrup. Food Sci Technol Int. 2013; 19(4):323–333. PMID: 23729415.


38. Kang YR, Lee HY, Kim JH, Moon DI, Seo MY, Park SH, Choi KH, Kim CR, Kim SH, Oh JH, Cho SW, Kim SY, Kim MG, Chae SW, Kim O, Oh HG. Anti-obesity and anti-diabetic effects of Yerba Mate (Ilex paraguariensis) in C57BL/6J mice fed a high-fat diet. Lab Anim Res. 2012; 28(1):23–29. PMID: 22474471.


39. Matsuzawa Y, Funalhashi T, Nakamura T. Molecular mechanism of metabolic syndrome X: contribution of adipocytokines adipocyte-derived bioactive substance. Ann J Clin Nutr. 1999; 52:426–430.
40. Nieman DC, Henson DA, Nehlsen-Cannarella SL, Ekkens M, Utter AC, Butterworth DE, Fagoaga OR. Influence of obesity on immune function. J Am Diet Assoc. 1999; 99(3):294–299. PMID: 10076580.


41. Ryu JS, Lee HJ, Bae SH, Kim SY, Park Y, Suh HJ, Jeong YH. The bioavailability of red ginseng extract fermented by Phellinus linteus. J Ginseng Res. 2013; 37(1):108–116. PMID: 23717164.
Figure 1
Schematic diagram of the experimental protocol. Mice were acclimated for 1 week and induced obesity and normal chew mice model with high fat diet or normal diet for 3 weeks. In case of treatment with GS-E3D or placebo, they were orally administered for 4 weeks and sacrificed.
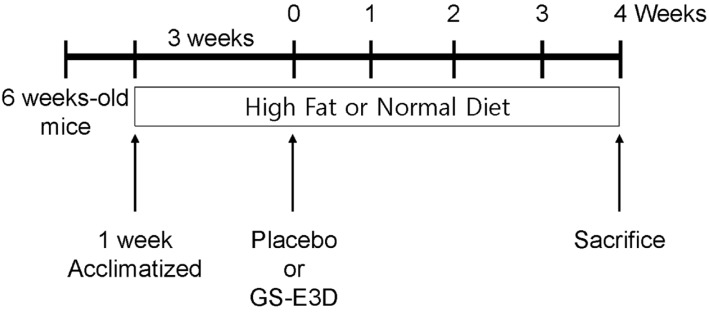
Figure 2
Cell viability of 3T3-L1 adipocytes against pectin lyase-modified red ginseng extracts (GS-E3D). 3T3-L1 cells (5×103 cells/well) were grown in 96-well plates and treated with different concentrations (0.1, 0.5, 1, 2 and 5 mg/mL) of GS-E3D. Differences were tested for significance by a one-way ANOVA (Duncan's multiple-range test). a,b,cValues in the row with different superscript letters are significantly different; P<0.05.
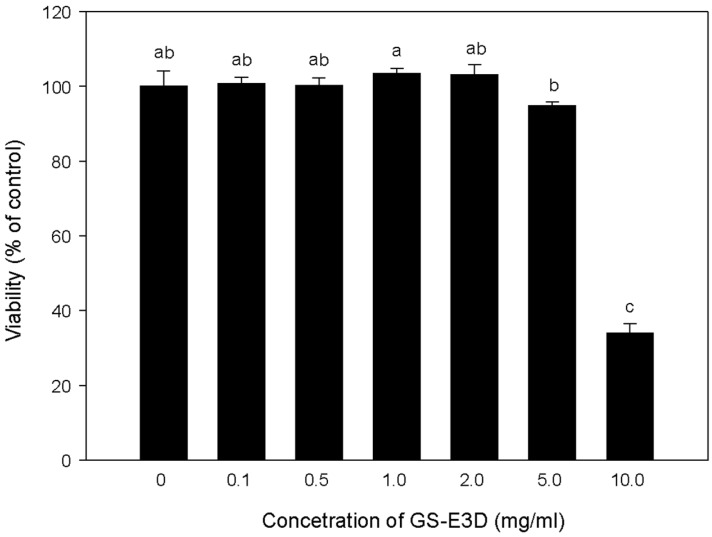
Figure 3
Effects of GS-E3D on adipocyte differentiation. Stained oil droplets with Oil Red O were dissolved with adipogensis assay kit and quantified by spectrophotometric analysis at 500 nm. a,b,c,d,eValues in the row with different superscript letters are significantly different; P<0.05.
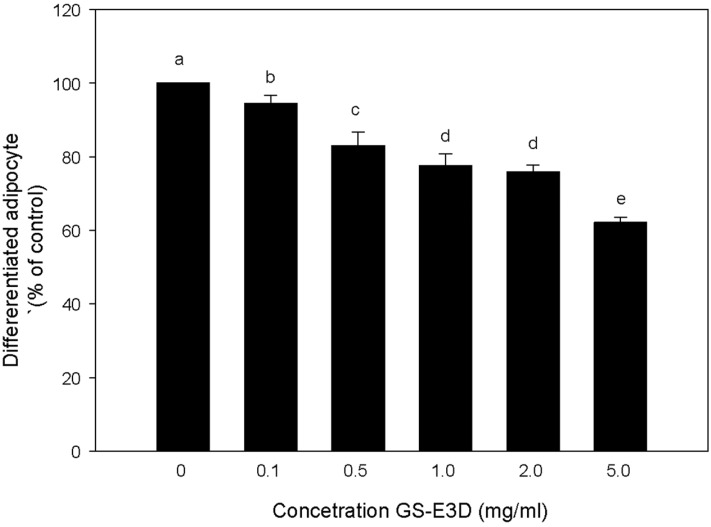
Figure 4
Effect of GS-E3D on the (A) body weight, (B) food and (C) water intake in the mice. *P<0.001 for high fat diet vs normal diet group. Data are shown as the mean±SE (n=8).
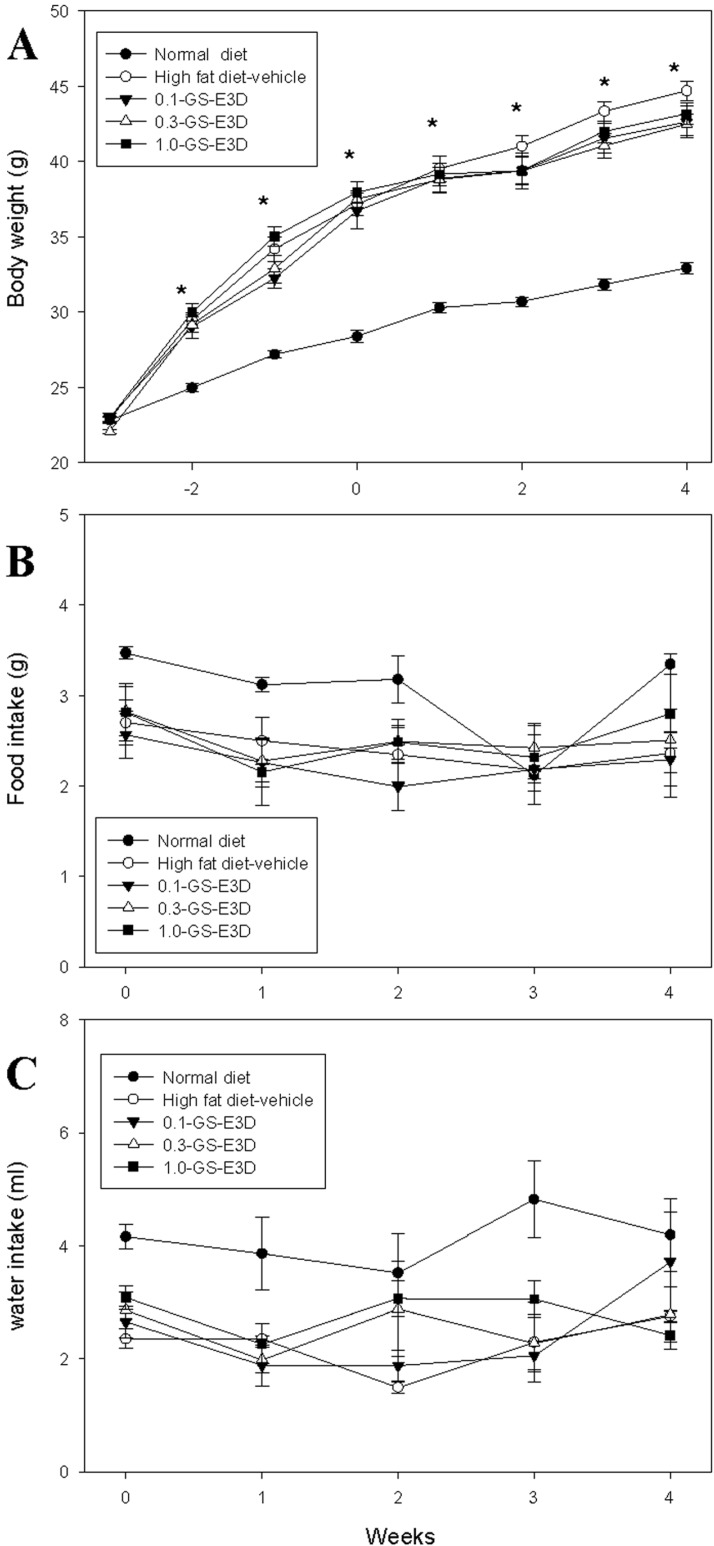
Figure 5
Effect of GS-E3D on changes in blood glucose in an obesity model induced by a high-fat diet. Data are shown as the mean±SE (n=8).
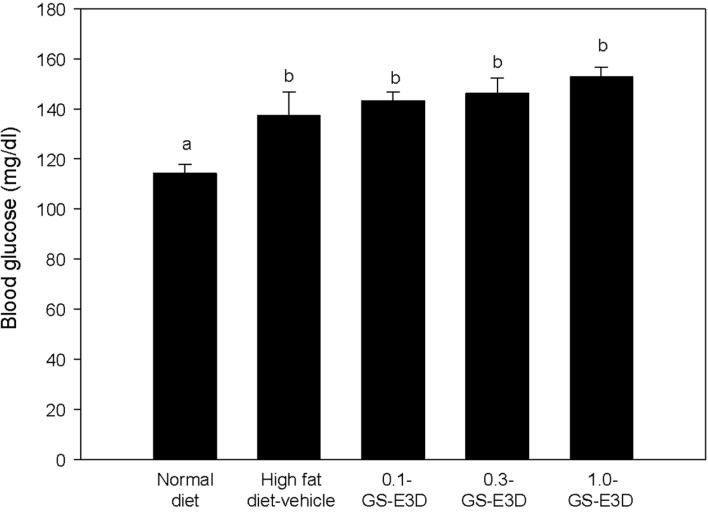
Figure 6
Effect of GS-E3D on changes in blood parameters in an obesity model induced by a high-fat diet. (A) total cholesterol (T-CHO), (B) low density lipoprotein (LDL-C) and (C) high density lipoprotein (HDL-C), (D) triglyceride (TG) in the whole blood of mice. a,b,cValues in the row with different superscripts are significantly different; P<0.05. Data are shown as the mean±SE (n=7).
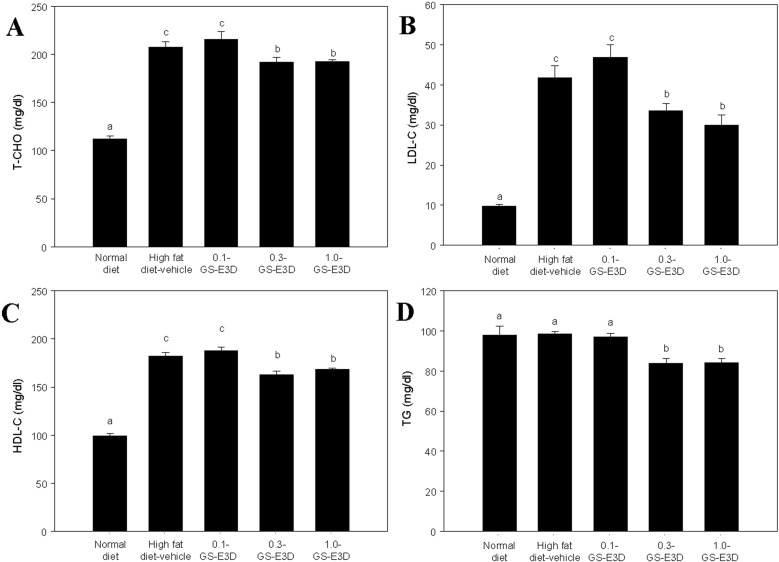
Figure 7
Effects of GS-E3D on the leptin level in a mouse obesity model induced by a high-fat diet. a,bValues in the row with different superscript letters are significantly different; P<0.05. Data are shown as mean±SE (n=7).
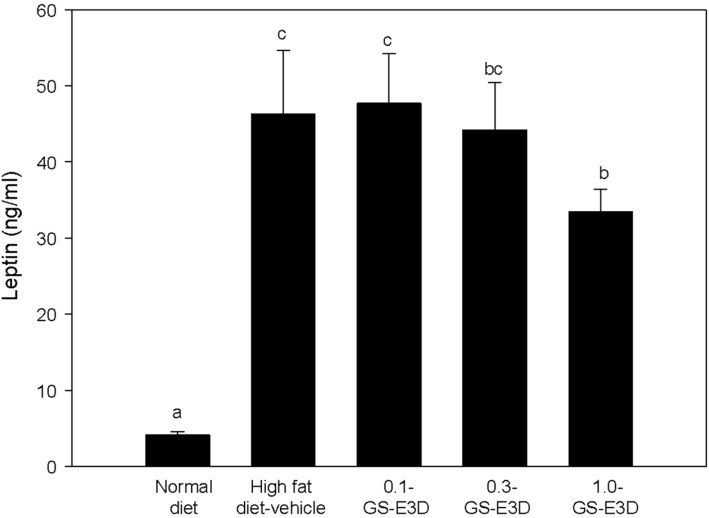
Figure 8
Effect of GS-E3D on the fat (epididymal fat, peritoneal fat, retroperitoneal fat, liver fat) weight in the mice. a,b,cValues in the row with different superscripts are significantly different; P<0.05. Data are shown as the mean±SE (n=8).
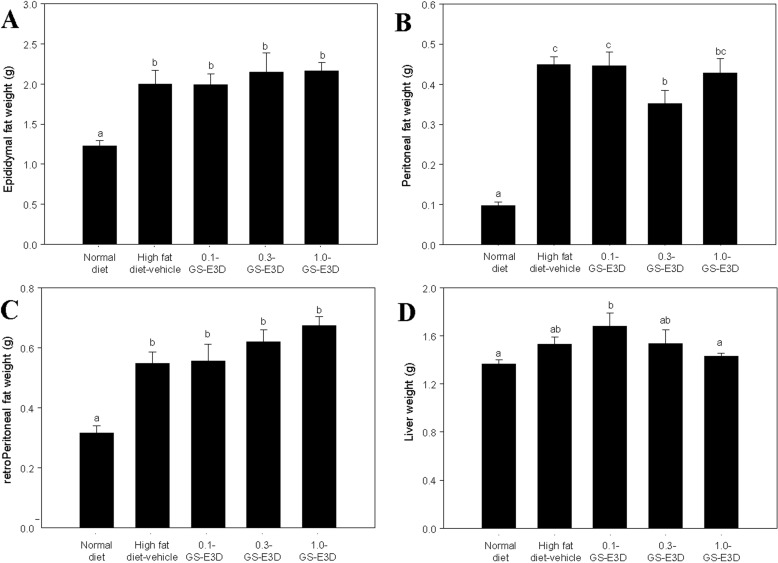
Figure 9
Effect of GS-E3D on the absolute organ weights of spleen, thymus, testis and kidney. Data are shown as the mean±SE (n=8).
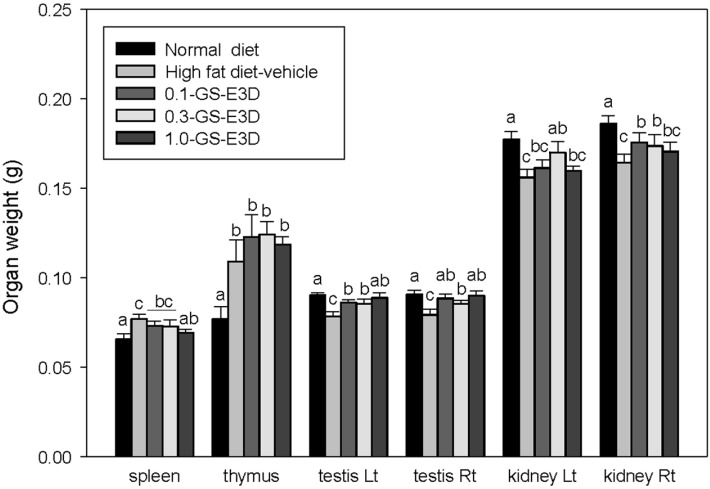
Figure 10
Effects of GS-E3D on histological adipocyte size in a mouse obesity model induced by a high-fat diet. (A) Normal group, (B) high fat diet-vehicle group, (C) high fat diet+ GS-E3D 0.1 g/kg, (D) high fat diet+GS-E3D 0.3 g/kg, (E) high fat diet+GS-E3D 1.0 g/kg, (F) quantitative analysis (400× magnification). a,b,c,dValues in the row with different superscript letters are significantly different; P<0.05. Data are shown as mean±SE (n=7).
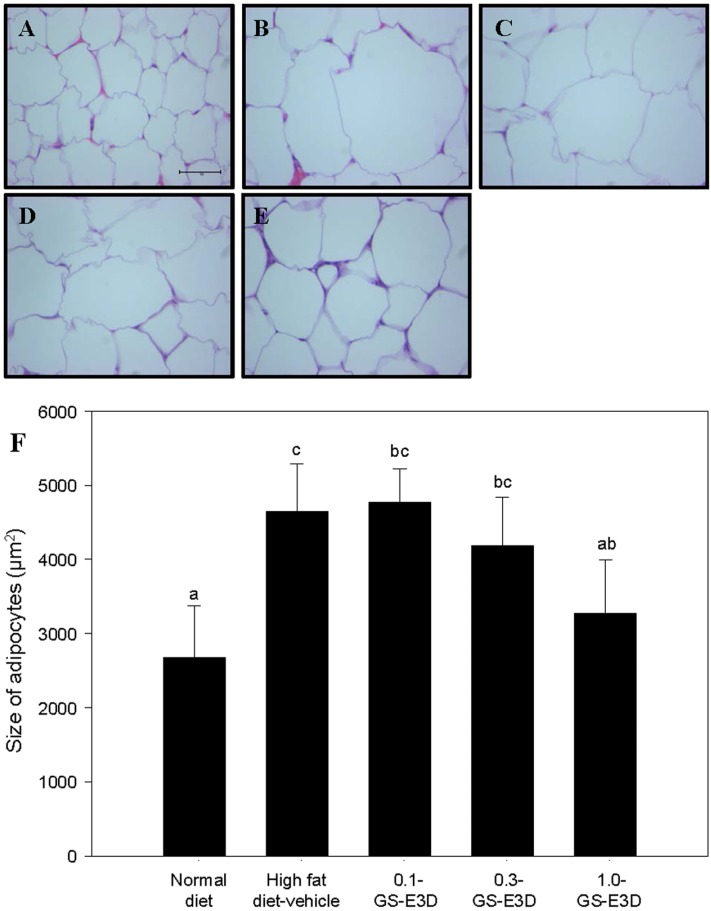
Figure 11
Effects of GS-E3D on histological hepatic steatosis in a mouse obesity model induced by a high-fat diet. (A) Normal group, (B) high fat diet-vehicle group, (C) high fat diet+GS-E3D 0.1 g/kg, (D) high fat diet+GS-E3D 0.3 g/kg, (E) high fat diet+GS-E3D 1.0 g/kg, (F) steatosis grade score. (400× magnification). Scale bar=100 µµm. a,b,c,dValues in the row with different superscript letters are significantly different; P<0.05. Data are shown as mean±SE (n=8).
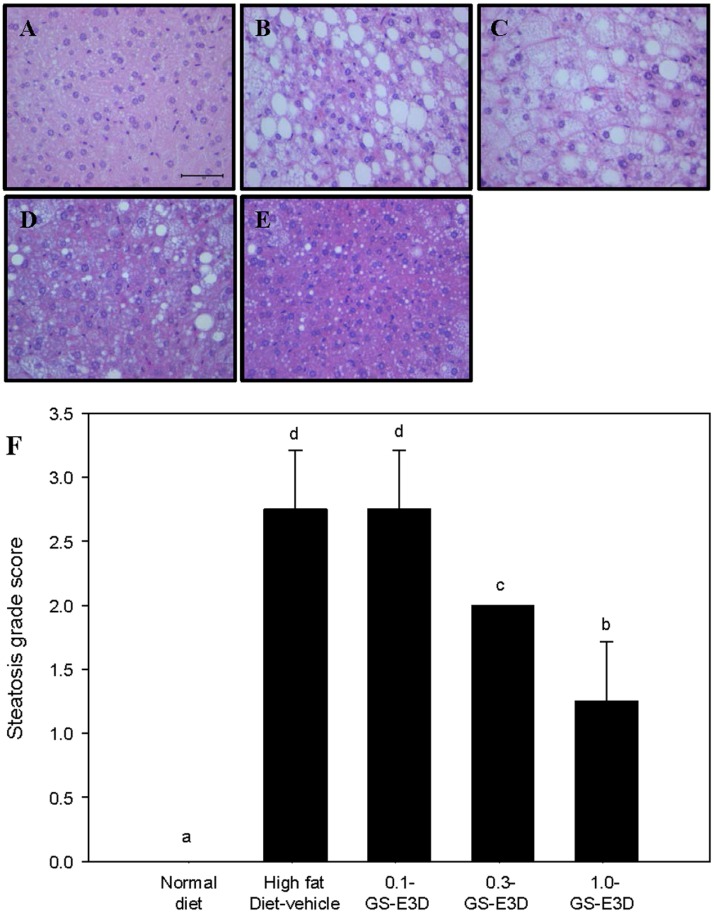