Abstract
Endocrine-disrupting chemicals (EDCs) are exogenous substances that alter the structure or function of the endocrine system. 4-Tert-octylphenol (OP) is one of the most representative EDCs and has estrogenic effects. In this study, we examined the effects of ethinyl estradiol (EE) and OP on the pituitary gland, placenta, and uterus of pregnant rats. Expression levels of human chorionic gonadotropin (hCG), oxytocin (OT), and contraction-associated proteins (CAPs) were determined, and uterine contractile activity was measured by uterine contraction assay. EE and OP both increased mRNA expression of OT and hCG in the pituitary gland but not the placenta. Since OT and hCG control uterine contraction, we next examined CAP expression in the uterus. Expression of 15-hydroxyprostaglandin-dehydrogenase (PGDH) was upregulated by OP, whereas expression of other CAPs was unaffected. To clarify the effect of OP on uterine contraction in pregnant rats, uterine contraction assay was performed. The 17β-Estradiol (E2) did not affect contraction of primary uterine cells harvested from pregnant rats in a 3D collagen gel model. However, OP showed different effects from E2 by significantly reducing contraction activity. In summary, we demonstrated that OP interferes with regulation of OT and hCG in the pituitary gland as well as PGDH in the uterus, thereby reducing uterine contraction activity. This result differs from the action of endogenous E2. Collectively, these findings suggest that exposure to EDCs such as OP during pregnancycan reduce uterine contractile ability, which may result in contraction-associated adverse effects such as metratonia, bradytocia, and uterine leiomyomata.
Endocrine-disrupting chemicals (EDCs) are known as exogenous chemical substances or mixtures that alter the structure or function of the endocrine system as well as have adverse effects on the reproductive system in animals and humans [1]. Use of EDCs is widespread in paints, household articles, toiletries, pesticide formulations, and many other industrial and agricultural products. Chemicals previously defined as EDCs include dichlorodiphenyltrichloroethane (DDT), polychlorinated biphenyls (PCBs), bisphenol A (BPA), 4-tert-octylphenol (OP), nonylphenol, polybrominateddiphenyl ethers (PBDEs), and a variety of phthalates [2]. One of the most representative of these chemicals is OP, which is 5 to 20-fold more estrogenic than nonylphenol while 100 to 10000-fold less estrogenic than 17β-estradiol (E2) [3]. OP has been shown to reduce serum concentrations of luteinizing hormone (LH), follicle-stimulating hormone (FSH), and testosterone as well as stimulate prolactin production. Further, OP affects the secretion of reproductive hormones and has similar effects as endogenous E2. As estrogenic activity is associated with uterine contraction during pregnancy, we expect that OP may affect pregnancy maintenance and labor. A previous study has suggested that OP can stimulate uterine contraction in immature rats [4]. Stable uterine contractile activity is an essential factor for maintenance of pregnancy as well as appropriate timing of childbirth. On the other hand, inappropriate uterine contraction can lead to premature birth and even abortion, both of which are currently recognized as leading causes of perinatal death in developed nations. In addition to E2, many endocrine factors such as progesterone (P4), human chorionic gonadotropin (hCG), oxytocin (OT), and prostaglandin (PG) are associated with uterine contraction and maintenance of pregnancy [5,6]. Thus, these hormones must be in correct balance for successful pregnancy.
The uterus frequently contracts throughout the entire menstrual cycle. During pregnancy, contractility of the uterine smooth muscle is reduced by progesterone. Progesterone further inhibits myometrial contractility in vitro and myometrial gap junction formation for pregnancy maintenance. Progesterone also down-regulates PG production as well as the development of calcium channels and oxytocin receptors (OTR), which are both involved in myometrial contraction [7]. During the early stages of pregnancy, progesterone is produced by the corpus luteum, and this event is essential for supporting pregnancy and establishing the placenta. In most mammals, progesterone production by the corpus luteum is required for successful pregnancy. Corpus luteum formation and production of progesterone is regulated by hCG, which is produced by the placenta as well as partially by the pituitary gland during the beginning of pregnancy [8]. The hCG acts on hCG receptor, which is expressed in uterine spiral arteries, to promote angiogenesis [9]. This process serves as one of the major roles of hCG in the uterus by insuring adequate blood supply or nutrition to the placenta [10]. hCG also has an important function in trophoblast tissue of the placenta, promoting fusion of cytotrophoblast cells and their differentiation into syncytiotrophoblasts [11]. Lastly, hCG is a heterodimer with an α subunit identical to those of LH, FSH, and thyroid-stimulating hormone (TSH), in addition to a unique β subunit [12].
OT together with endorphin and adrenaline play major roles in regulating labor and birth. OT is a neuropeptide that is synthesized centrally in the paraventricular and supraoptic nuclei of the hypothalamus, after which it is released into the bloodstream via the posterior pituitary [13]. OT levels rise upon onset of labor, causing regular contractions of the uterus and abdominal muscles through the OTR [14]. Combined with high levels of E2, OT stimulates the release of PG hormones, which may play a role in ripening of the cervix [15,16]. PGs also mediate a variety of strong physiological effects, such as regulation of smooth muscle tissue contraction and relaxation [17]. Another PG, prostaglandin F2α (PGF2α), acts through prostaglandin-F2α receptor (FP) on the myometrium to up-regulate OTR and gap junctions, thereby promoting uterine contractions [18]. PGs are degraded by the metabolizing enzyme 15-hydroxyprostaglandin-dehydrogenase (PGDH). The above-mentioned genes, including OTR, FP, and PGDH, are collectively known as contraction-associated proteins (CAPs).
In the present study, we examined the effects of EE and OP on rat uterine contractions in pregnant rats. To explore the mechanism underlying EE and OP activities, expression levels of contraction-regulating hormones such as OT and hCG as well as genes encoding CAPs were analyzed. Contractility of the rat uterus was further evaluated using a three-dimensional (3D) collagen gel contraction assay.
Ethinyl estradiol (EE) and corn oil were purchased from Sigma Chemical Co. (St. Louis, MO, USA). 4-Tert-octylphenol (OP) was obtained from Fluka Chemie (Seoul, Republic of Korea).
Pregnant Sprague-Dawley (SD; 40 females) rats were purchased from SamTako (Osan, Republic of Korea) and handled at the Pusan National University Laboratory Animal Resources Center, which is accredited by the Korea FDA according to National Institutes of Health guidelines. Rats were housed in cages with a 12-h light/dark cycle and constant temperature of 23±1℃. Rats were fed a diet of soy-free pellets (SamTako, Osan, Korea). From gestational days (GD) 14 to 16, rats were treated daily with EE (500 µg/kg/d), OP (10, 100, or 500 mg/kg/d), or corn oil (5 mL/kg/d) as a vehicle control via gavage. Dosage was adjusted according to changes in body weight (BW). BW, clinical signs, and abnormal behaviors were recorded daily throughout the experimental period. All animals were immediately sacrificed using CO2 gas for preparation of tissue samples, which were stored in eppendorf tubes at -70℃.
Total RNA was extracted using Trizol reagent (Invitrogen Co., Carlsbad, CA, USA) according to the manufacturer's protocol. The concentration of total RNA was measured by a spectrophotometer. First-strand complementary DNA (cDNA) was prepared from total RNA (3 µg) by reverse transcription (RT) using M-MLV reverse transcriptase (Invitrogen Co.) and random primers (9-mers; TaKaRa Bio Inc., Shiga, Japan). Quantitative real-time PCR (Q-PCR) was performed with cDNA template (2 µL) and 2× Power SYBR Green (6 µL; TOYOBO) containing specific primers. Primer sequences for OT, OTR, FP, α-hCG, β-hCG, and PGDH are shown in Supplemental Table S1. Q-PCR was carried out for 40 cycles using the following parameters: denaturation at 95℃ for 15 s, followed by annealing and extension at 70℃ for 60 s. Fluorescence intensity was measured at the end of the extension phase of each cycle. The threshold value for the fluorescence intensity of all samples was set manually. The reaction cycle at which PCR products exceeded the fluorescence intensity threshold during exponential phase of PCR amplification was considered to be the threshold cycle (CT). Expression of the target gene was quantified relative to that of 1A and beta-actin, which are ubiquitous housekeeping genes, based on comparison of CTs at constant fluorescence intensity.
Uterine tissues were taken from pregnant SD rats, cut into pieces less than 3 mm in size, and digested with 0.2% collagenase (type I; Sigma Chemical Co.). The digested tissues were washed and filtered using 100-µm Spectra Mesh filters (Spectrumlabs Inc., Rancho Dominguez, CA, USA), and the isolated uterine cells were prepared for 3D cultures.
Collagen gels were prepared from rat tail type 1 collagen (Millipore, Bedford, MA, USA) at a final concentration of 1.5 mg/mL and then added to 12-well tissue culture dishes containing 50,000 uterine cells according to the technique described by Dallot et al. [19]. Briefly, cells in the collagen gel were allowed to equilibrate overnight in serum- and phenol red-free DMEM medium (Gibco BRL, Grand Island, NY, USA). The next day, the gel lattices were physically detached from the culture dishes, and E2 and OP were added. The lattices treated with 10% (v/v) FBS were used as positive controls, and unstimulated cells served as a negative control.
Gel images were captured using a Canon digital camera T1i (Canon Inc., Tokyo, Japan), and the area (cm2) of the lattices was measured using Image J software (version 1.45×; National Institutes of Health, Bethesda, MD, USA). For each condition, contractile activity was determined in triplicate, and each experiment was performed at least three times. Results were expressed as mean gel area (cm2)±standard error of the mean (SEM). The original area of the collagen gels was captured before treatment and used as an internal control. Percentages of increased or decreased gel areas were compared to that of unstimulated lattices.
To evaluate the effects of OP in the pituitary gland during pregnancy, rats were administered EE (500 µg/kg/d) and OP (10, 100, and 500 mg/kg/d) for 3d from GD14 to GD16. Since pregnancy is maintained by several hormones such as OT, hCG, and progesterone, expression levels of genes related to these hormones were measured in the pituitary gland. OT, a mammalian hormone generally produced by the hypothalamus, is critically associated with uterine contraction and thus commonly used to induce labor. However, peripheral synthesis of OT in other tissues, including the pituitary gland and uterus, has also been reported [20,21]. hCG is also a key hormone for maintaining pregnancy and is synthesized in both the pituitary gland and placenta during pregnancy. hCG stimulates the corpus luteum to secrete progesterone, which is called "the pregnancy hormone". Therefore, hCG can also be considered as important in pregnancy maintenance. For the initial experiment, expression levels of OT, α-hCG, and β-hCG were investigated in the pituitary gland, placenta, and uterus.
In the pituitary gland, transcription levels of OT significantly increased (up to 5.1- and 3.8-fold) at high concentrations of OP (500 mg/kg/d) and EE (500 µg/kg/d) (Figure 1). Transcription levels of common α-hCG subunit were altered by 500 mg/kg of OP, whereas the effect of EE was not significant. Transcription of hCG-specific subunit, β-hCG, was drastically up-regulated 13.4-fold by OP (500 mg/kg/d) and 10.2-fold by EE (500 µg/kg/d).
The placenta plays many roles during pregnancy by supporting transfer of nutrients to the fetus and by producing pregnancy maintenance hormones. During pregnancy, hCG is mainly present in the placenta. Unexpectedly, transcription levels of α- and β-hCG were unaffected by EE or OP in the placenta. These results indicate that expression of hCG was differentially regulated in a tissue-specific manner by EE and OP (Figure 2).
OP is an estrogenic EDC while E2 supposedly regulates uterine contraction [4]. In the present study, OP regulated expression of OT and hCG, which are also known to be related with uterine contraction. Therefore, we next examined the expression levels of CAPs including OT, OTR, FP, and PGDH, in the uterus. OT mRNA expression was not significantly regulated by either EE or OP in the uterus, although it was highly regulated in the pituitary gland.
OTR are expressed by the myometrium and endometrium of the uterus at the end of pregnancy. The OT system plays an important role as an inducer of uterine contraction during parturition as well as a signaling mediator of OT in the uterus. Similar to OT, expression of OTR was not regulated by either OP or EE.
PGF2α is a known inducer of labor and is another critical factor in uterine contraction. PGF2α signaling is mediated and regulated by expression of FP, the specific receptor for PGF2α [22]. PGDH catalyzes degradation of PGF2α and serves as a negative indicator of uterine contraction [23]. In the current study, mRNA expression levels of PGDH and FP were measured. PGDH transcription was dose-dependently up-regulated by OP administration but not EE. These results show that OP but not EE increased PGDH gene expression, thereby inhibiting uterine contraction. In contrast, FP expression was not regulated by EE or OP (Figure 3).
Since OP regulated expression of PGDH, which is a CAP, we next investigated the effect of OP on uterine contraction by 3D collagen gel contraction assay.
The areas of collagen lattices were measured at 24 and 48 h after treatment with E2 (10-7 M), OP (10-7 M), and 10% FBS, and contraction activity was normalized relative to the size of each gel before treatment. When the lattices were detached from the underlying plate, primary uterine cells contracted and reduced the areas of the 3D gels over 48h in serum-free DMEM, revealing their basal contractility (Figure 4). Contractile activity was first elevated by E2 and 10% FBS at 24 h, and these increases persisted for up to 48 h. E2 enhanced contractility by about 20% at 24 h and 80% at 48 h. Interestingly, OP showed no effect oncontraction of rat uterine cells, which was opposite that of E2.
EDCs are well known to have adverse effects on female reproductive organs, including the uterus. The main function of the uterus is to accept a fertilized ovum that then implants into the endometrium of the uterus and develops into a fetus until childbirth. During gestation, the uterus is relaxed so it can hold the fetus during development, which is controlled by P4. At the end of gestation, this muscular organ contracts to induce labor under the control of endogenous hormones such as E2, P4, OT, and PGF2α [24]. In the present study, we examined the effects of EE and OP on reproductive organs related to pregnancy in rats. First, we examined the expression levels of OT and hCG in the pituitary gland, which is a small organ located in the brain that secretes many hormones that function in the regulation of endocrine systems. The posterior pituitary gland consists mainly of neuronal projections (axons) of magnocellular neurosecretory cells extending from the supraoptic and paraventricular nuclei of the hypothalamus. These axons store and release neurohypophysial hormones such as OT and vasopressin into neurohypohyseal capillaries. In addition, gonadotrope cells of the anterior pituitary gland produce pituitary hCG [12]. There are four independent variants of hCG, each of which is produced by different cells. During pregnancy, hCG is mainly produced by placental villous syncytiotrophoblast cells. The hCG can also be produced by cytotrophoblast cells, in which its free β-subunit is made by multiple primary nontrophoblastic malignancies. Lastly, hCG can be produced by gonadotrope cells of the anterior pituitary gland [25]. Although they have different biological functions, all hCG molecules share the same β-subunit amino acid sequence. hCG is a heterodimer composed of a common α-subunit and hCG-specific β-subunit. The α-subunit of hCG is common to hyperglycosylated hCG, pituitary hCG, LH, FSH, and TSH. Reports have shown that pituitary hCG has approximately half biological activity than that of placenta in promoting progesterone production in the placenta [26]. E2 is known to regulate established anterior pituitary gland hormones as well as stimulate the release of OT from the hypothalamus [27]. The present results show that administration of EE and OP upregulated OT and hCG in the pituitary gland of pregnant rats. These results suggest that EE as well as OP have a stimulatory effect on the production of OT and hCG in the pituitary gland as a type of positive feedback mechanism. However, in the placenta, OT and hCG production was not stimulated by OP or EE, suggesting production of OT and hCG is regulated in a tissue-specific manner.
Regulation of myometrial contractility is modulated the expression of genes encoding CAPs. OT is a main mediator of uterine contraction and labor, and OTR expression dramatically increases in the myometrium at term [22,28]. Bossmar et al. demonstrated that OT expression is detectable in the uterus during pregnancy in rats, and this does not differ according to gestational age [29]. However, regulation of CAPs by E2 remains controversial. Recent studies have revealed that E2 upregulates OTR expression in the uterus of pregnant and non-pregnant animals [30,31]. In a previous study in ovariectomized rats, OT expression significantly stimulated by E2 was reduced by P4, even though P4 alone did not alter OT expression [29]. However, in another study, EE treatment alone did not show any effect on parturition [32]. Further, E2 alone or in combination with OT was shown to have no significant effect, whereas it enhances trypsin-induced contraction [33]. Our results suggest that administration of EE and OP does not modulate expression of OT and OTR in the uterus of pregnant rats. It is possible that the uterus during pregnancy may be already saturated with endogenous E2 and is thus unresponsive to exogenous administration.
Another important pathway for uterine contraction is PGF2α signaling, and elevation of FP is associated with induction of uterine contraction [34]. In the present study, expression of PGF2α signaling-related factors, including PGDH and FP, was evaluated. PGDH oxidizes and inactivates PG at the 15-hydroxyl group [35]. During pre-term and term, expression of PGDH is reduced in the uterus and placenta, which indicates that PG produced in these tissues are biologically active, thereby inducing uterine contraction and labor [23].
The effects of E2 on PGDH and FP expression are not well understood. E2 has been shown to increase the activity of PGDH in the rat uterus [36]. Furthermore, antagonist of E2 during the early menstrual cycle reduces PGDH activity and increases PG production, suggesting that E2 inactivates PGDH [37]. However, in another study, E2 was shown to have no effect on PGDH activity in cultured placenta cells [38]. Dong et al. demonstrated that E2 significantly increases FP mRNA expression in the uterus of ovariectomized rats [39].
In the present study, the effects of EE and OP on PGF2α-related gene expression were different. PGDH expression was up-regulated by OP but not EE, whereas FP was not regulated by either EE or OP. These results suggest that OP and EE may have different effects on the regulation of PGDH expression in the uterus. To clarify the effects of OP on uterine contraction during pregnancy, we performed uterine contraction assay. Uterine contractile activity is traditionally estimated by measuring the isometric force of longitudinal uterine sections using tension transducers in organ baths. Since this method can be affected by many factors such as buffer composition and other experimental conditions, we employed 3D collagen gel contraction assay originally developed by Dallot et al. [29]. We demonstrated for the first time that E2 did not affect contraction of primary uterine cells harvested from pregnant rats using a 3D collagen gel model. In contrast, OP significantly reduced uterine contraction. These results were consistent with our previous study showing that OP and BPA reduce uterine cell contractility in immature rats [4].
In summary, we examined the effects of EE and OP on uterine contraction and its associated factors in pregnant rats. Our results demonstrate that OP interferes with regulation of OT and hCG in the pituitary glandas well as PGDH in the uterus, thereby reducing uterine contraction activity. This result is in contrast to the action of endogenous E2. These results further suggest that exposure to EDCs such as OP can reduce uterine contractile ability, which may cause contraction-associated adverse effects such as metratonia, bradytocia, and uterine leiomyomata.
References
1. Nilsson R. Endocrine modulators in the food chain and environment. Toxicol Pathol. 2000; 28(3):420–431. PMID: 10862560.


2. Rudel RA, Perovich LJ. Endocrine disrupting chemicals in indoor and outdoor air. Atmos Environ (1994). 2009; 43(1):170–181. PMID: 20047015.


3. White R, Jobling S, Hoare SA, Sumpter JP, Parker MG. Environmentally persistent alkylphenolic compounds are estrogenic. Endocrinology. 1994; 135(1):175–182. PMID: 8013351.


4. An BS, Ahn HJ, Kang HS, Jung EM, Yang H, Hong EJ, Jeung EB. Effects of estrogen and estrogenic compounds, 4-tert-octylphenol, and bisphenol A on the uterine contraction and contraction-associated proteins in rats. Mol Cell Endocrinol. 2013; 375(1-2):27–34. PMID: 23664861.


5. Kota SK, Gayatri K, Jammula S, Kota SK, Krishna SV, Meher LK, Modi KD. Endocrinology of parturition. Indian J Endocrinol Metab. 2013; 17(1):50–59. PMID: 23776853.


6. Terranova PF. The female reproductive system. Medical Physiology. 2nd ed. Philadelphia: Lippincott, Williams & Wilkins;2003. p. 667–683.
7. White R, Jobling S, Hoare SA, Sumpter JP, Parker MG. Environmentally persistent alkylphenolic compounds are estrogenic. Endocrinology. 1994; 135(1):175–182. PMID: 8013351.


8. Hoermann R, Spoettl G, Moncayo R, Mann K. Evidence for the presence of human chorionic gonadotropin (hCG) and free beta-subunit of hCG in the human pituitary. J Clin Endocrinol Metab. 1990; 71(1):179–186. PMID: 1695224.
9. Zygmunt M, Herr F, Keller-Schoenwetter S, Kunzi-Rapp K, Münstedt K, Rao CV, Lang U, Preissner KT. Characterization of human chorionic gonadotropin as a novel angiogenic factor. J Clin Endocrinol Metab. 2002; 87(11):5290–5296. PMID: 12414904.


10. Shi QJ, Lei ZM, Rao CV, Lin J. Novel role of human chorionic gonadotropin in differentiation of human cytotrophoblasts. Endocrinology. 1993; 132(3):1387–1395. PMID: 7679981.


11. Cronier L, Bastide B, Hervé JC, Délèze J, Malassiné A. Gap junctional communication during human trophoblast differentiation: influence of human chorionic gonadotropin. Endocrinology. 1994; 135(1):402–408. PMID: 8013377.


12. Pierce JG, Parsons TF. Glycoprotein hormones: structure and function. Annu Rev Biochem. 1981; 50:465–495. PMID: 6267989.


13. Jenkins JS, Nussey SS. The role of oxytocin: present concepts. Clin Endocrinol (Oxf). 1991; 34(6):515–525. PMID: 1889133.


14. Fuchs AR, Fuchs F, Husslein P, Soloff MS. Oxytocin receptors in the human uterus during pregnancy and parturition. Am J Obstet Gynecol. 1984; 150(6):734–741. PMID: 6093538.


15. Gimpl G, Fahrenholz F. The oxytocin receptor system: structure, function, and regulation. Physiol Rev. 2001; 81(2):629–683. PMID: 11274341.
16. Jagani N, Schulman H, Fleischer A, Mitchell J, Blattner P. Role of prostaglandin-induced cervical changes in labor induction. Obstet Gynecol. 1984; 63(2):225–229. PMID: 6582419.
17. Nelson RJ. An introduction to behavioral endocrinology.Sinauer Associates. 2005. p. 100.
18. Fuchs AR, Rollyson MK, Meyer M, Fields MJ, Minix JM, Randel RD. Oxytocin induces prostaglandin F2 alpha release in pregnant cows: influence of gestational age and oxytocin receptor concentrations. Biol Reprod. 1996; 54(3):647–653. PMID: 8835387.
19. Dallot E, Pouchelet M, Gouhier N, Cabrol D, Ferré F, Breuiller-Fouché M. Contraction of cultured human uterine smooth muscle cells after stimulation with endothelin-1. Biol Reprod. 2003; 68(3):937–942. PMID: 12604645.


20. Dave JR, Culp SG, Liu L, Tabakoff B, Hoffman PL. Regulation of vasopressin and oxytocin synthesis in anterior pituitary and peripheral tissues. Adv Alcohol Subst Abuse. 1988; 7(3-4):231–234. PMID: 3223431.
21. Lefebvre DL, Giaid A, Bennett H, Larivière R, Zingg HH. Oxytocin gene expression in rat uterus. Science. 1992; 256(5063):1553–1555. PMID: 1598587.


22. Engstrom T, Bratholm P, Vilhardt H, Christensen NJ. Effect of oxytocin receptor and beta2-adrenoceptor blockade on myometrial oxytocin receptors in parturient rats. Biol Reprod. 1999; 60(2):322–329. PMID: 9915997.
23. Challis JR, Sloboda DM, Alfaidy N, Lye SJ, Gibb W, Patel FA, Whittle WL, Newnham JP. Prostaglandins and mechanisms of preterm birth. Reproduction. 2002; 124(1):1–17.
24. Riemer RK, Heymann MA. Regulation of uterine smooth muscle function during gestation. Pediatr Res. 1998; 44(5):615–627. PMID: 9803440.


25. Kovalevskaya G, Genbacev O, Fisher SJ, Caceres E, O'Connor JF. Trophoblast origin of hCG isoforms: cytotrophoblasts are the primary source of choriocarcinoma-like hCG. Mol Cell Endocrinol. 2002; 194(1-2):147–155. PMID: 12242037.


26. Birken S, Maydelman Y, Gawinowicz MA, Pound A, Liu Y, Hartree AS. Isolation and characterization of human pituitary chorionic gonadotropin. Endocrinology. 1996; 137(4):1402–1411. PMID: 8625917.


27. Lischke A, Gamer M, Berger C, Grossmann A, Hauenstein K, Heinrichs M, Herpertz SC, Domes G. Oxytocin increases amygdala reactivity to threatening scenes in females. Psychoneuroendocrinology. 2012; 37(9):1431–1438. PMID: 22365820.


28. Kimura T, Takemura M, Nomura S, Nobunaga T, Kubota Y, Inoue T, Hashimoto K, Kumazawa I, Ito Y, Ohashi K, Koyama M, Azuma C, Kitamura Y, Saji F. Expression of oxytocin receptor in human pregnant myometrium. Endocrinology. 1996; 137(2):780–785. PMID: 8593830.


29. Bossmar T, Osman N, Zilahi E, Haj MA, Nowotny N, Conlon JM. Expression of the oxytocin gene, but not the vasopressin gene, in the rat uterus during pregnancy: influence of oestradiol and progesterone. J Endocrinol. 2007; 193(1):121–126. PMID: 17400809.


30. Murata T, Narita K, Honda K, Higuchi T. Changes of receptor mRNAs for oxytocin and estrogen during the estrous cycle in rat uterus. J Vet Med Sci. 2003; 65(6):707–712. PMID: 12867731.


31. Murata T, Murata E, Liu CX, Narita K, Honda K, Higuchi T. Oxytocin receptor gene expression in rat uterus: regulation by ovarian steroids. J Endocrinol. 2000; 166(1):45–52. PMID: 10856882.


32. Novy MJ, Walsh SW. Dexamethasone and estradiol treatment in pregnant rhesus macaques: effects on gestational length, maternal plasma hormones, and fetal growth. Am J Obstet Gynecol. 1983; 145(8):920–931. PMID: 6837680.


33. Aman M, Hirano K, Nishimura J, Nakano H, Kanaide H. Enhancement of trypsin-induced contraction by in vivo treatment with 17beta-estradiol and progesterone in rat myometrium. Br J Pharmacol. 2005; 146(3):425–434. PMID: 16056237.
34. Mesiano S, Welsh TN. Steroid hormone control of myometrial contractility and parturition. Semin Cell Dev Biol. 2007; 18(3):321–331. PMID: 17613262.


35. Parent M, Madore E, MacLaren LA, Fortier MA. 15-Hydroxyprostaglandin dehydrogenase in the bovine endometrium during the oestrous cycle and early pregnancy. Reproduction. 2006; 131(3):573–582. PMID: 16514200.


36. Matsuo M, Ensor CM, Tai HH. Characterization of the genomic structure and promoter of the mouse NAD+-dependent 15-hydroxyprostaglandin dehydrogenase gene. Biochem Biophys Res Commun. 1997; 235(3):582–586. PMID: 9207200.


37. Kelly RW, Linan C, Thong J, Yong EL, Baird DT. Prostaglandin inactivation is increased in endometrium after exposure to clomiphene. Prostaglandins Leukot Essent Fatty Acids. 1994; 50(5):235–238. PMID: 8066097.


38. Patel FA, Clifton VL, Chwalisz K, Challis JR. Steroid regulation of prostaglandin dehydrogenase activity and expression in human term placenta and chorio-decidua in relation to labor. J Clin Endocrinol Metab. 1999; 84(1):291–299. PMID: 9920098.


39. Dong YL, Yallampalli C. Pregnancy and exogenous steroid treatments modulate the expression of relaxant EP(2) and contractile FP receptors in the rat uterus. Biol Reprod. 2000; 62(3):533–539. PMID: 10684792.
Figure 1
Transcriptional regulation of hCG and OT by EE and OP in the pituitary gland.Transcription levels of OT, α-hCG, and β-hCG were analyzed by Q-PCR. Data were expressed as the mean±SD of at least six rats per group. Gene expression level was normalized to that of an internal control gene (1A). *P<0.05 compared to control group.

Figure 2
Transcriptional regulation of hCG and OT by EE and OP in the placenta. Transcription levels of OT, α-hCG, and β-hCG were analyzed by Q-PCR. Data were expressed as the mean±SD of at least six rats per group. Gene expression level was normalized to that of an internal control gene (1A). *P<0.05 compared to control group.
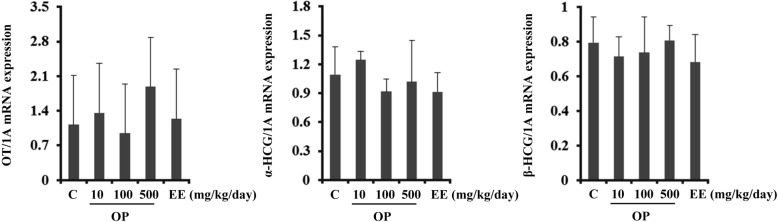
Figure 3
Transcriptional regulation of CAPs by EE and OP in the uterus. Transcription levels of OT, OTR, PGDH, and FP were analyzed by Q-PCR. Data were expressed as the mean±SD of at least six rats per group. Gene expression level was normalized to that of an internal control gene (β-actin). *P<0.05 compared to control group.
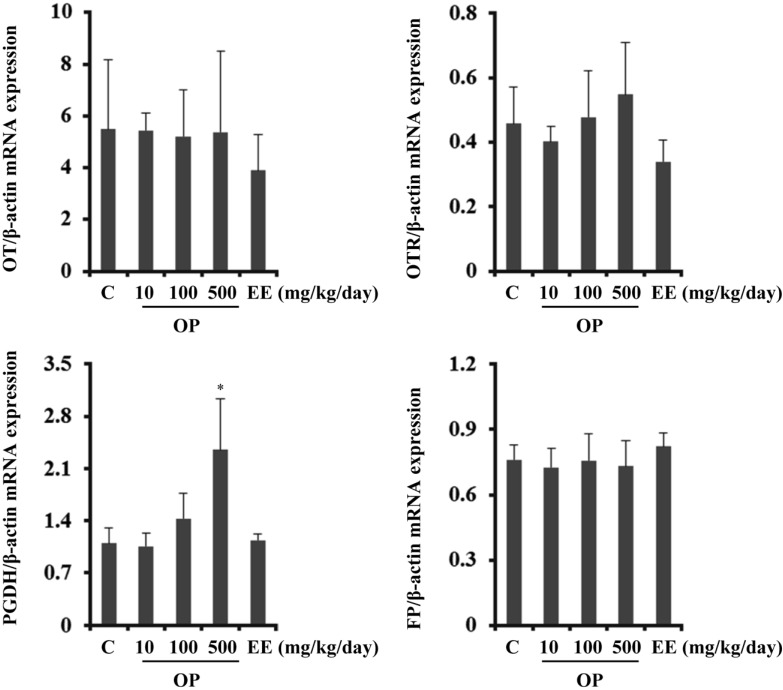
Figure 4
Uterine contraction differentially modulated by E2 and OP. Primary uterine cells from pregnant rats were mixed with a collagen solution and seeded in 12-well plates for 3D contraction assay. Collagen lattices were treated with E2 (10-7 M) and OP (10-7 M) for the indicated time periods. Images were captured and analyzed to measure contractile activity. 10% FBS were used as positive controls while unstimulated lattices were used as a negative control. *P<0.05 compared to control group at 24 h and #P<0.05 compared to control group at 48 h.
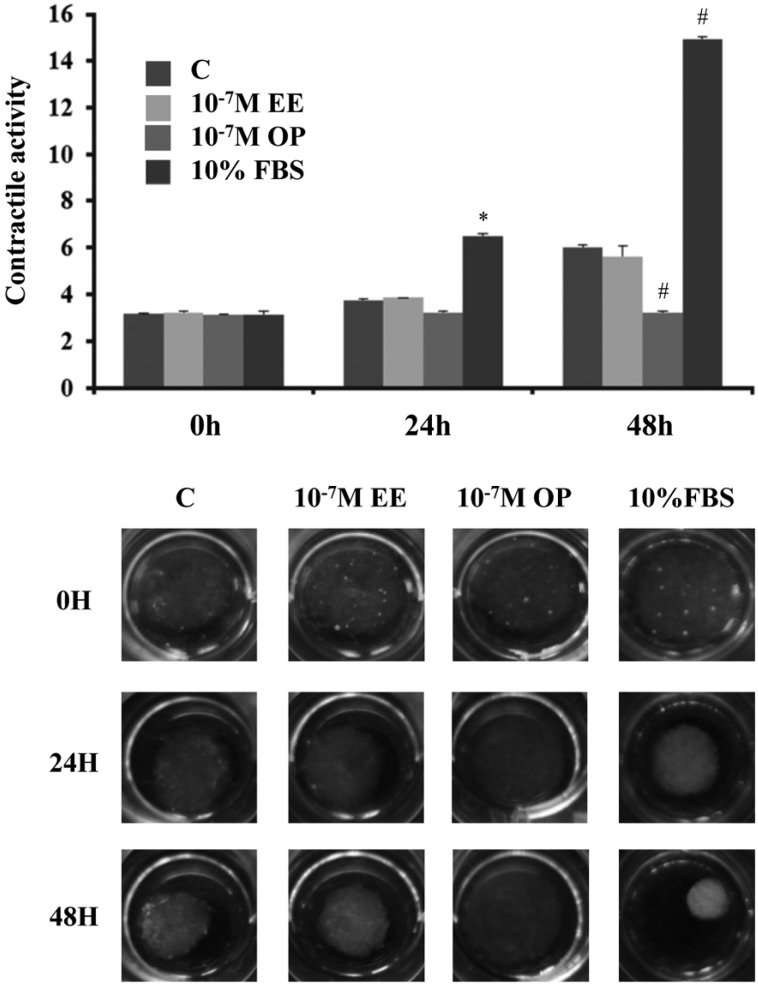