Abstract
Although ginseng (genus Panax) leaf extract contains high concentrations of bioactive constituents, its effects have been reported in few preclinical studies, and information regarding its toxicity is not sufficient to allow for its clinical use. We evaluated the genotoxicity of UG0712, which is a powdered extract of ginseng leaves. UG0712 did not increase the number of revertant colonies in 4 histidine auxotrophic strains of Salmonella typhimurium (TA100, TA1535, TA98, and TA1537) or in a tryptophan auxotrophic strain of Escherichia coli (WP2uvrA(pKM101)) at any concentration evaluated, either in the absence or presence of the metabolic activation system. There was no significant increase in the number of metaphase cells with structural or numerical aberrations in the UG0712-treated groups compared to the concurrent vehicle control at any dose, regardless of the presence of the metabolic activation system. Oral administration of the extract at doses up to 2,000 mg/kg in male mice did not increase the frequency of micronucleated polychromatic erythrocytes in the bone marrow, and did not result in any significant clinical signs, body weight loss, gross findings, or mortality. These results suggest that UG0712 does not act as a mutagenic or genotoxic material at the concentrations evaluated.
Ginseng, especially ginseng root, is a commonly used traditional herbal medicine in East Asian countries including China, Korea, and Japan, for its tonic, adaptogenic, restorative, immunomodulatory, anti-inflammatory, anti-oxidant, anti-aging, and anti-cancer properties [1,2,3,4]. Ginseng is also one of the most commonly used dietary supplements in North America [4,5,6]. The study of ginseng has a long history [1]. The bioactive ingredients found in ginseng include more than 60 ginsenosides, such as Rb1, Rb2, Rb3, Rc, Rd, Re, Rg1, Rg2, and Rg3, as well as polysaccharides, oligopeptides, polyacetylenic alcohols, and fatty acids [1,7,8]. Ginsenosides, which are regarded as the major bioactive constituents of ginseng, have a four-ring, steroid-like structure with sugar moieties [9] and have been shown to produce multiple pharmacological effects [1]. Although the pharmacological effects of ginseng root are well known, its use is limited by its significant cost [4,10]. Other parts of the ginseng plant, such as the berry, stem, and leaf are much cheaper than the root, and the leaf can be harvested every year.
Recently, it has been reported that ginseng leaf contains numerous bioactive ingredients in high concentrations that are also found in ginseng root, such as ginsenosides, polysaccharides, triterpenoids, flavonoids, polyacetylenic alcohols, volatile oils, peptides, amino acids, and fatty acids [4,11,12]. The total ginsenoside content in the leaf of American ginseng (Panax quinquefolius) is significantly higher than that in the root and the berry [13]. The major ginsenosides found in ginseng leaf are Rd, Re, and Rb2 [13]. Ginseng leaf extract has been shown to exert pharmacological effects in the central nervous system (CNS), the cardiovascular system, and the immune system [4].
Although many studies have reported the therapeutic efficacy of ginseng leaf extract, there is little evidence in the scientific literature on its toxicity, and thus recommendations for the safe use of the extract are not well established. Therefore, in the present study, we report results from a series of standardized, Organisation for Economic Co-operation and Development (OECD)- and Korea Food and Drug Administration (KFDA) guideline-compliant in vitro and in vivo studies that were undertaken to investigate the genotoxicity of ginseng leaf extract UG0712. Assays included the bacterial reverse mutation test, the chromosome aberration test, and the bone marrow micronucleus test.
All positive controls and cofactor ingredients for the preparation of the S9 fraction were obtained from Sigma-Aldrich (St. Louis, MO, USA), and dimethyl sulfoxide (DMSO) was obtained from Merck (USA). The S9 fraction was prepared from male Sprague-Dawley rats treated with Aroclor 1254, which was obtained from Molecular Toxicology Inc. (USA).
UG0712 was prepared by blending partially hydrolyzed ginseng leaf extract in a manner that led to consistent content of ginsenosides Rd and Rg3. Briefly, ginseng leaf extract purchased from Hongju Biotech Co., Ltd. (Yanbian, China) was hydrolyzed under acidic conditions. UG0712 was standardized to contain greater than 5% ginsenoside Rd, greater than 10% ginsenoside Rg3 derivatives (Rg3, Rg5, and Rk1).
The appropriate amount of UG0712 for the highest dose was weighed and added to tubes containing pure DMSO or 0.5% DMSO for the bacterial reverse mutation test and the in vitro chromosome aberration test, respectively, and mixed with a vortexer until it was dissolved. The formulation was serially diluted with the appropriate DMSO vehicle to produce a series of lower doses that were separated by a geometric ratio of 2. The preparation of UG0712 formulations for injection in the in vivo micronucleus test was performed for each dose using water (Choongwae Pharma Corp.).
The bacterial reverse mutation test was performed using the methods (pre-incubation) described in the OECD and KFDA guidelines [14,15] by Maron and Ames [16] with minor modification. The mutagenic potential of UG0712 was examined in the absence and presence of a metabolic activation system using histidine-requiring Salmonella typhimurium TA98, TA100, TA1535, and TA1537 strains and the tryptophan-requiring Escherichia coli WP2uvrA (pKM101) strain. All strains were purchased from Molecular Toxicology Inc. (Boone, NC, USA). The specific genotype characteristics of the S. typhimurium strains were evaluated, including their histidine requirement, the presence of the uvrB mutation, presence of R-factor, presence of the rfa mutation, and the number of spontaneous revertants. In the case of E. coli, the tryptophan requirement, the presence of the uvrA mutation, and the number of spontaneous revertants were tested according to the methods of Maron and Ames [16]. The viable cell counts in the bacterial cultures used in the test were greater than 1×109 cells per mL.
The dose range-finding test was carried out at 5 concentration levels (312.5, 625, 1,250, 2,500, and 5,000 µg/plate) in vehicle and strain-specific positive controls in the absence and presence of the metabolic activation system. The number of revertant colonies was reduced in the S. typhimurium TA98 strain in the absence of the metabolic activation system and in the S. typhimurium TA100 strain regardless of the presence of the metabolic activation system. However, turbidity and precipitation were not observed in any strain that was incubated with the UG0712 formulation for 48 h. Based on these results, the strain specific dose for the confirmatory test was selected and 2-fold serial dilutions were performed to yield 5 concentration levels. The number of revertant colonies was automatically counted using a colony counter (SINBIOSIS, UK). Results were expressed as the mean number of revertant colonies from triplicate plates per concentration (with standard deviation) and a ratio of the mean concurrent vehicle control value.
The in vitro chromosome aberration test was performed using the methods described in the OECD and KFDA guidelines [14,17] and based on published methods for cytogenetic tests [18,19,20]. The Chinese hamster lung (CHL/IU) cell line was purchased from the American Type Culture Collection (ATCC, USA); this cell line is the most frequently used line for the in vitro chromosome aberration test for regulatory submission owing to its high detection sensitivity.
A growth inhibition test (MTT assay) [21] was performed to determine the relative toxicity of UG0712 to the cells. In the growth inhibition test, the IC50 was calculated to be 100 µg/mL for the short-term (6-h) and continuous (24-h) treatments in the absence of the metabolic activation system, and calculated to be 800 µg/mL for the short-term (6-h) treatment in the presence of the metabolic activation system. Turbidity and precipitation were not observed at concentrations up to 5000 µg/mL in any treatment group at the beginning or the end of treatment. Using these results, the concentration range chosen for the confirmatory tests was designed with consideration of the cytotoxicity of UG0712. Treatment at each concentration was conducted in duplicate. The slides were prepared according to the hypotonic-methanol-glacial acetic acid-flame drying-giemsa stain method for metaphase plate analysis. At least 200 well-spread intact metaphase cells were scored for each concentration under 1000× magnification by using a differential interference microscope.
For the purposes of chromosome aberration identification, chromosome aberrations were classified into 2 groups: structural aberrations and numerical aberrations. Structural aberrations were subdivided into chromatid breaks (ctb), chromatid exchange (cte), chromosome breaks (csb), and chromosome exchange (cse). Numerical aberrations consisted of polyploidy (pol) only. Two types of aberration, chromatid and chromosome gap, were recorded, but not included in the calculation of the aberration rates. In the metaphase, because there were several gaps or cuttings, aberrations were recorded as fragments (frg). The criteria used to define chromosome aberrations were based on the report of Sofuni et al. [20]. If an appearance rate was less than 5%, between 5 and 10%, or higher than 10%, it was considered to be negative, equivocal, or positive, respectively.
The in vivo micronucleus test was performed using the methods described in the OECD and KFDA guidelines [14,22] and based on the published methods for cytogenetic tests [23,24]. Specific pathogen-free male mice (IcrTacSam:ICR, 27.86-30.44 g, approximately 7 weeks of age) were obtained from Samtako Bio Korea Inc. (Osan, Korea) and used after 9 days of acclimation. The animals were housed in polycarbonate cages with bedding. An ambient temperature of 21.7-23.6℃, a relative humidity of 47.7-59.9%, an air ventilation rate of 10-15 changes/h, a light intensity of 150-300 lux, and a photoperiod of 12 h were maintained throughout the study. A commercial pellet diet and water were provided ad libitum.
The dose range-finding test was performed using 5 dose levels (125, 250, 500, 1,000, and 2,000 mg/kg), the highest of which was the maximum dose for treatment up to 14 days, according to OECD guidelines. After administration, the mice did not show any clinical signs or behavioral alterations during the observation period. The frequencies of micronucleated polychromatic erythrocytes (MNPCEs) did not change for 72 h after dosing in the 2,000 mg/kg group. Based on these results, the in vivo micronucleus test was conducted at 3 dose levels (500, 1,000, and 2,000 mg/10 mL/kg). UG0712 was administered by a single oral gavage, whereas the positive control was administered intraperitoneally. All animals were sacrificed by cervical dislocation approximately 24 h after the administration. The micronucleus test was carried out according to the method described by Schmid [24]. Smears were allowed to dry, fixed with methanol, and stained with a 5% giemsa solution for 30 min. The stained slides were washed and allowed to air-dry. Slides were then examined under 1,000× magnification by observers who were blind to the treatments. A total of 2000 polychromatic erythrocytes (PCEs) were scored per animal by the same observer to determine the frequency of MNPCEs. The PCE/(PCE+NCE) ratio, an indicator of cytotoxicity, was calculated by counting 1000 total erythrocytes per animal. The results were judged as positive when there was a statistically significant and dose-related reproducible increase in the frequencies of micronucleated polychromatic erythrocytes for at least 1 dose level.
The table of Kastenbaun and Bowman [25] was used to determine if the frequencies of micronucleated polychromatic erythrocytes for individual dose groups significantly increased. The t-test and ANOVA were conducted on the differences in PCE/(PCE+NCE) ratio and body weight change, respectively. Statistical tests were regarded as significant when the P-value was less than 0.05.
The results of the bacterial reverse mutation test using UG0712 are shown in Figure 1 and Table 1. No contaminant colonies were observed on the sterility plates for the highest concentration of UG0712 and the metabolic activation system. The number of revertant colonies in all strains was not increased more than 2-fold compared to the concurrent vehicle control in the absence or presence of the metabolic activation system. However, indicators of growth inhibition, such as a decrease in the number of colonies compared to that for the concurrent vehicle control, was observed at the 625 µg/plate concentration in the S. typhimurium TA98 and TA100 strains in the absence of the metabolic activation system, and at the 1,250 µg/plate concentration in the S. typhimurium TA100 strain in the presence of the metabolic activation system. Turbidity and precipitation were not observed in any strain regardless of the presence of the metabolic activation system. The positive controls induced significant increases (defined as ≥4 times that of the concurrent vehicle control) in the number of revertant colonies, indicating that the test was valid.
The number of metaphase cells with structural and numerical aberrations is presented in Table 2. The number of aberrant metaphase cells at the concentrations of UG0712 tested ranged from 0.5 to 2.0% for the short-term (6-h) treatment in the absence or presence of the metabolic activation system, and from 0.5 to 1.5% for the continuous (22-h) treatment in the absence of the metabolic activation system. Therefore, the number of aberrant metaphase cells was not increased compared with that of the concurrent vehicle control at any concentration, regardless of the presence of the metabolic activation system. The number of aberrant metaphase cells in the positive control group in the absence or presence of the metabolic activation system was 15.5 and 15.5%, respectively, for the short-term treatment, and 15.0% the continuous treatment in the absence of the metabolic activation system, indicating that the test was valid.
No clinical signs or mortality related to UG0712 treatment was observed, and no significant body weight changes were noted during the observation period in any animal. Table 3 shows the frequencies of micronucleated polychromatic erythrocytes per 2000 polychromatic erythrocytes (PCEs) and the ratios of PCEs per 1000 erythrocytes (PCE/[PCE+NCE]), which is an indicator of cytotoxicity. The frequencies of MNPCEs were 0.40, 0.40, 0.60, and 0.60, respectively, in the vehicle control, 500, 1,000, and 2,000mg/kg treatment groups. Therefore, no statistically significant increase in the frequency of micronucleated polychromatic erythrocytes compared to the concurrent vehicle control group was observed at any dose of UG0712. The ratio of PCEs per 1000 erythrocytes (PCE/[PCE+NCE]) in the vehicle control, 500, 1,000, and 2,000 mg/kg treatment groups was 0.48, 0.44, 0.47, and 0.43, respectively, and there were no significant differences between the vehicle control group and the UG0712-treated groups. The frequency of micronucleated polychromatic erythrocytes and the ratio of PCE/(PCE+NCE) in the positive control group was 132.80 and 0.46, respectively, and the frequency of micronucleated polychromatic erythrocytes was significantly increased in comparison to that of the concurrent vehicle control group, indicating that the present study was performed under acceptable experimental conditions, and supporting the validity of the results.
Traditional herbal medicine has developed for several thousand years to maintain the equilibrium of the human body under diseases or stress in the east of Asial including China, Korea and Japan [1,26,27,28,29]. But on the contrary to its effectiveness, the toxicity of herbs used as the traditional medicine has become a major obstacle in clinical application. Traditionally, general toxicities of medicinal herbs are often introduced in ancient literatures. However, there is no information on its genetic studies based on the scientific demonstration.
Ginseng has been used for several thousand years as a traditional herbal medicine to promote longevity, enhance resistance to diseases [1,26,27,28,29]. Ginseng root, which is harvested after several years' cultivation, is used more often than other parts of the ginseng plant such as berry, stem and leaf although ginseng leaf contains similar active ingredients with pharmacological effects and have larger amounts of the same active ingredients than its root [4,11]. The extracts of ginseng leaf have multifaceted pharmacological effects in the central nervous system and a broad range of beneficial effects including anti-fatigue, anti-hyperglycemic, anti-obesity, anti-cancer, anti-oxidant and anti-aging activities [4,30]. Ginseng berry extract has been reported to show better anti-hyperglycemic activity than ginseng root extract when used at the same concentration [31], and have a higher content of total ginsenosides than its root extract [13]. It was reported that American ginseng berry and leaf extract significantly reduced body weight in adult ob/ob and db/db mice, models of type 2 diabetes [32,33].
Although ginseng plants have been extensively studied for their therapeutic benefits, evidence-based information on the safety of ginseng leaf extract is limited. According to previous reports, the LD50 value of ginseng leaf extract was approximately 625 mg/kg when administered to mice by intraperitoneal injection [34], and ginseng leaf extract did not affect blood cell counts, hemoglobin levels, or renal function in a subacute toxicity study [4]. Zhang et al. demonstrated that panax ginseng has no genotoxicity by the comet assay using mouse peripheral lymphocytes [35]. Furthermore, they reported that total saponins from stem and leaf of panax ginseng inhibit cyclophosphamide-induced DNA damage and apoptosis in mouse bone marrow cells and peripheral lymphocyte cells, implying anti-clastogenic and anti-oxidant effects of panax ginseng [36].
To investigate the safety of UG0712 ginseng leaf extract, several safety tests were carried out in this study, because no single test can detect every genotoxic effect [37]. The most commonly used method for assessing the genotoxic potential of herbal medicines is the bacterial reverse mutation test in S. typhimurium and E. coli [38]. The objective of this test is to reveal the mutagenic potential of a test substance in a prokaryote organism and determine whether the reactive metabolite is a product of the metabolic activation system. The number of revertant colonies in S. typhimurium (TA98, TA100, TA1535, and TA1537) and E. coli (WP2uvrA) strains was not increased in comparison to the concurrent vehicle control in the absence or presence of the metabolic activation system. However, growth inhibition was observed in S. typhimurium TA98 and TA100 strains regardless of the presence of the metabolic activation system.
The in vitro chromosome aberration (CA) test is a widely used assessment of genotoxicity, because many birth defects and human genetic diseases are associated with abnormal chromosome complements. In the chromosome aberration test, the number of metaphase cells with structural and numerical aberrations was not increased compared with that of the concurrent vehicle control at any concentration, regardless of the presence of the metabolic activation system. Although the current preferred method of staining for the micronucleus (MN) assay is acridine orange staining [39], giemsa was chosen in this study because it is a permanent stain that does not fade when exposed to strong light during the scoring period. Giemsa stain was found to be acceptable by an international expert group that recently reviewed the in vivo rodent erythrocyte micronucleus assay in the International Workshop on Genotoxicity Test Procedures [23]. The time of peak micronuclei induction should be selected as the sacrifice time of the mutagens. The frequency of micronucleated polychromatic erythrocytes did not change at 24, 48, and 72 h after administration of 2,000 mg/kg in the dose range-finding test. Based on this information, mice were sacrificed approximately 24 h after dosing. In male mice, a single oral adminstration of UG0712 at doses up to 2,000 mg/kg did not induce an increase in the frequency of micronucleated polychromatic erythrocytes in the bone marrow.
UG0712 ginseng leaf extract did not cause a mutagenic response in 4 S. typhimurium strains or 1 E. coli strain, did not induce clastogenicity in the in vitro chromosome aberration test using Chinese hamster lung cells, and did not induce an increase in the frequency of micronucleated polychromatic erythrocytes in mouse bone marrow cells. No dose-related increase was observed in any of the parameters measured, suggesting that there were no adverse effects associated with UG0712 treatment. Our results suggest that UG0712 is safe for use in terms of genotoxicity.
References
1. Attele AS, Wu JA, Yuan CS. Ginseng pharmacology: multiple constituents and multiple actions. Biochem Pharmacol. 1999; 58(11):1685–1693. PMID: 10571242.
2. Cheng Y, Shen LH, Zhang JT. Anti-amnestic and anti-aging effects of ginsenoside Rg1 and Rb1 and its mechanism of action. Acta Pharmacol Sin. 2005; 26(2):143–149. PMID: 15663889.


3. Shin HR, Kim JY, Yun TK, Morgan G, Vainio H. The cancer-preventive potential of Panax ginseng: a review of human and experimental evidence. Cancer Causes Control. 2000; 11(6):565–576. PMID: 10880039.
4. Wang H, Peng D, Xie J. Ginseng leaf-stem: bioactive constituents and pharmacological functions. Chin Med. 2009; 4:20. PMID: 19849852.


6. Wang Z, Zheng Q, Liu K, Li G, Zheng R. Ginsenoside Rh(2) enhances antitumour activity and decreases genotoxic effect of cyclophosphamide. Basic Clin Pharmacol Toxicol. 2006; 98(4):411–415. PMID: 16623867.


7. Lee FC. Facts About Ginseng: The Elixir of Life. Hollym International Corporation;1992.
8. Qi X, Ignatova S, Luo G, Liang Q, Jun FW, Wang Y, Sutherland I. Preparative isolation and purification of ginsenosides Rf, Re, Rd and Rb1 from the roots of Panax ginseng with a salt/containing solvent system and flow step-gradient by high performance counter-current chromatography coupled with an evaporative light scattering detector. J Chromatogr A. 2010; 1217(13):1995–2001. PMID: 20171644.


9. Raghavendran HR, Sathyanath R, Shin J, Kim HK, Han JM, Cho J, Son CG. Panax ginseng modulates cytokines in bone marrow toxicity and myelopoiesis: ginsenoside Rg1 partially supports myelopoiesis. PLoS One. 2012; 7(4):e33733. PMID: 22523542.


10. Ceylan-Isik AF, Fliethman RM, Wold LE, Ren J. Herbal and traditional Chinese medicine for the treatment of cardiovascular complications in diabetes mellitus. Curr Diabetes Rev. 2008; 4(4):320–328. PMID: 18991600.


11. Hou JP. The chemical constituents of ginseng plants. Comp Med East West. 1977; 5(2):123–145. PMID: 608333.


12. Zhang QH, Wu CF, Duan L, Yang JY. Protective effects of total saponins from stem and leaf of Panax ginseng against cyclophosphamide-induced genotoxicity and apoptosis in mouse bone marrow cells and peripheral lymphocyte cells. Food Chem Toxicol. 2008; 46(1):293–302. PMID: 17904265.


13. Xie JT, Mehendale SR, Wang A, Han AH, Wu JA, Osinski J, Yuan CS. American ginseng leaf: ginsenoside analysis and hypoglycemic activity. Pharmacol Res. 2004; 49(2):113–117. PMID: 14643691.


14. KFDA. Testing Guidelines for Safety Evaluation of Drugs (Notification No. 2014-136) issued by the Korea Food and Drug Administration. 2014.
15. OECD. Guideline for Testing of Chemicals, Section 4, Health Effects, No. 471. 1997.
16. Maron DM, Ames BN. Revised methods for the Salmonella mutagenicity test. Mutat Res. 1983; 113(3-4):173–215. PMID: 6341825.


17. OECD. Guideline for Testing of Chemicals, Section 4, Health Effects, No. 473. 1997.
18. Ishidate M Jr. Data book of chromosomal aberration test in vitro. Elsevier;1998.
19. Ishidate M Jr, Odashima S. Chromosome tests with 134 compounds on Chinese hamster cells in vitro--a screening for chemical carcinogens. Mutat Res. 1977; 48(3-4):337–353. PMID: 876270.
20. Sofuni T, Matsuoka A, Sawada M, Ishidate M Jr, Zeiger E, Shelby MD. A comparison of chromosome aberration induction by 25 compounds tested by two Chinese hamster cell (CHL and CHO) systems in culture. Mutat Res. 1990; 241(2):175–213. PMID: 2345556.


21. Mosmann T. Rapid colorimetric assay for cellular growth and survival: application to proliferation and cytotoxicity assays. J Immunol Methods. 1983; 65(1-2):55–63. PMID: 6606682.


22. OECD. Guideline for Testing of Chemicals, Section 4, Health Effects, No. 474. 1997.
23. Hayashi M, MacGregor JT, Gatehouse DG, Adler ID, Blakey DH, Dertinger SD, Krishna G, Morita T, Russo A, Sutou S. In vivo rodent erythrocyte micronucleus assay. II. Some aspects of protocol design including repeated treatments, integration with toxicity testing, and automated scoring. Environ Mol Mutagen. 2000; 35(3):234–252. PMID: 10737958.


25. Kastenbaum MA, Bowman KO. Tables for determining the statistical significance of mutation frequencies. Mutat Res. 1970; 9(5):527–549. PMID: 5424720.


26. Ben-Hur E, Fulder S. Effect of Panax ginseng saponins and Eleutherococcus senticosus on survival of cultured mammalian cells after ionizing radiation. Am J Chin Med. 1981; 9(1):48–56. PMID: 7304498.


27. Keum YS, Han SS, Chun KS, Park KK, Park JH, Lee SK, Surh YJ. Inhibitory effects of the ginsenoside Rg3 on phorbol ester-induced cyclooxygenase-2 expression, NF-kappaB activation and tumor promotion. Mutat Res. 2003; 523-524:75–85. PMID: 12628505.
28. Liu WK, Xu SX, Che CT. Anti-proliferative effect of ginseng saponins on human prostate cancer cell line. Life Sci. 2000; 67(11):1297–1306. PMID: 10972198.


29. Ong YC, Yong EL. Panax (ginseng)--panacea or placebo? Molecular and cellular basis of its pharmacological activity. Ann Acad Med Singapore. 2000; 29(1):42–46. PMID: 10748963.
30. Mochizuki M, Yoo YC, Matsuzawa K, Sato K, Saiki I, Tono-oka S, Samukawa K, Azuma I. Inhibitory effect of tumor metastasis in mice by saponins, ginsenoside-Rb2, 20(R)- and 20(S)-ginsenoside-Rg3, of red ginseng. Biol Pharm Bull. 1995; 18(9):1197–1202. PMID: 8845804.


31. Dey L, Xie JT, Wang A, Wu J, Maleckar SA, Yuan CS. Anti-hyperglycemic effects of ginseng: comparison between root and berry. Phytomedicine. 2003; 10(6-7):600–605. PMID: 13678250.


32. Attele AS, Zhou YP, Xie JT, Wu JA, Zhang L, Dey L, Pugh W, Rue PA, Polonsky KS, Yuan CS. Antidiabetic effects of Panax ginseng berry extract and the identification of an effective component. Diabetes. 2002; 51(6):1851–1858. PMID: 12031973.


33. Xie JT, Zhou YP, Dey L, Attele AS, Wu JA, Gu M, Polonsky KS, Yuan CS. Ginseng berry reduces blood glucose and body weight in db/db mice. Phytomedicine. 2002; 9(3):254–258. PMID: 12046868.


34. Wang BX, Cui JC, Liu AJ. The action of ginsenosides extracted from the stems and leaves of Panax ginseng in promoting animal growth. Yao Xue Xue Bao. 1982; 17(12):899–904. PMID: 7183121.
35. EMEA/HMPC/107079, Guideline on the Assessment of Genotoxicity of Herbal Substances/Preparations. 2007.
36. Abdelmigid HM. Sivakumar Gowder, editor. New Trends in genotoxicity testing of herbal medicinal plants, New Insights into Toxicity and Drug Testing. 2013. (ISBN: 978-953-51-0946-4, InTech, DOI: 10.5772/54858. Available from: http://www.intechopen.com/books/new-insights-into-toxicity-and-drug-testing/new-trends-in-genotoxicity-testing-of-herbal-medicinal-plants).
37. Tinwell H, Ashby J. Comparison of acridine orange and Giemsa stains in several mouse bone marrow micronucleus assays--including a triple dose study. Mutagenesis. 1989; 4(6):476–481. PMID: 2695763.


Figure 1
UG0712 dose-response curve for revertant colonies in the presence of the metabolic activation system. Five test strains (S. typhimurium TA98, TA100, TA1535, and TA1537, and E. coli WP2uvrA) were exposed to UG0712 and incubated for 48 h. Data were expressed as the mean values of colonies from 3 plates for each concentration. * is growth inhibition.
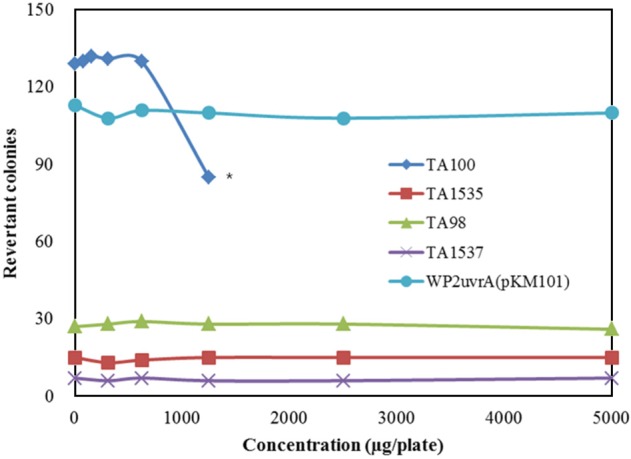
Table 1
Results of the bacterial reverse mutation test for UG0712 in the absence of the metabolic activation system
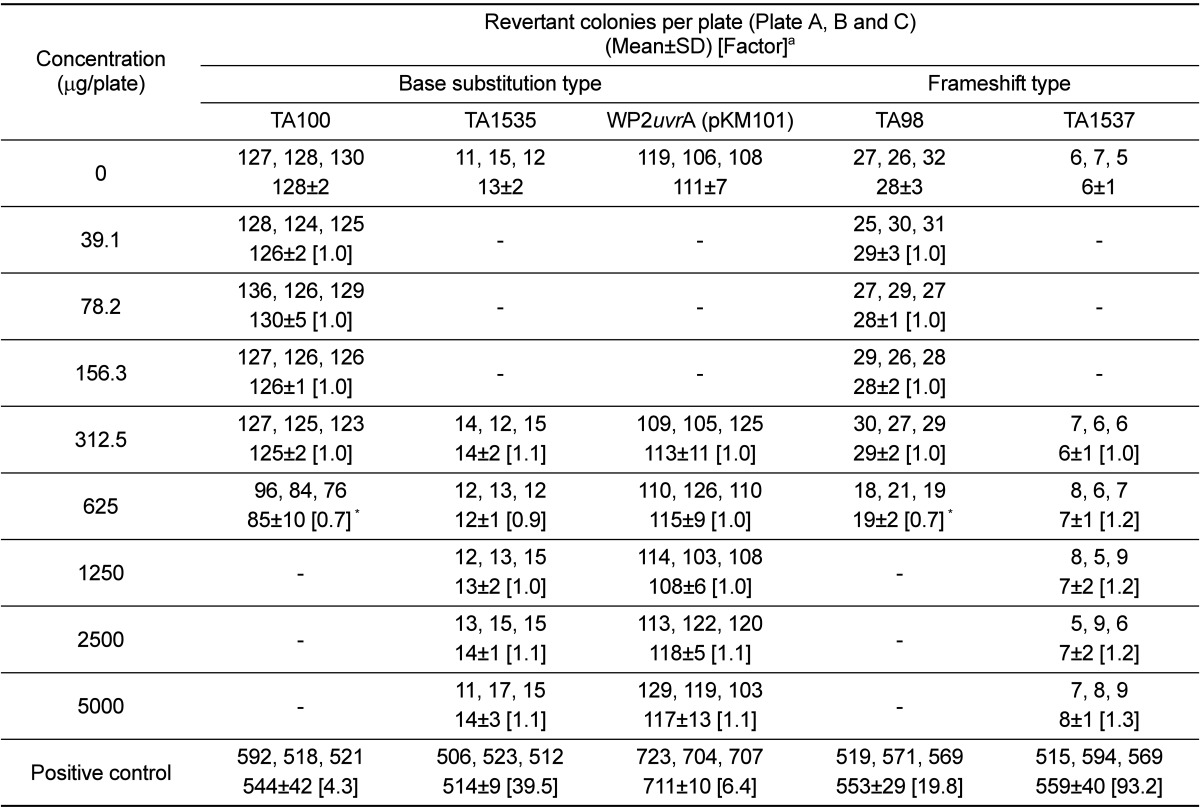