Abstract
According to a high anti-osteoporotic efficacy of Sigma Anti-bonding Molecule Calcium Carbonate (SAC), repeated-dose toxicities of SAC were investigated to assess its feasibility as drug or functional food ingredient. Male ICR mice were given drinking water containing 0.006, 0.02 or 0.06% SAC for 4 weeks. SAC feeding decreased the body weights and feed and water consumptions of mice in a dose-dependent manner, especially, leading to severe emaciation and 70% death in 3 weeks in the high-dose (0.06%) group. Not only kidney and heart weights, but also the levels of blood urea nitrogen, creatinine, aspartate transaminase, and creatine phospokinase significantly increased after SAC administration, indicative of nephrotoxicity and cardiotoxicity. Such renal and cardiac toxicities were also confirmed by microscopic findings, exhibiting renal crystals and cardiac fibrosis, which may be due to the insoluble crystal formation and calcium overload, respectively. In conclusion, it is suggested that no observed adverse effect level of SAC is lower than 0.006% in mice, and that a long-term intake may cause serious adverse effects on renal and cardiac functions.
Osteoporosis is classified as 3 types; postmenopausal osteoporosis (Type I), age-related osteoporosis (Type II), and secondary osteoporosis (Type III). Osteoporosis is a general health problem in men and women with a calcium imbalance due to decreased intestinal calcium absorption, insufficient dietary calcium intake, as well as increased urinary calcium loss associated with estrogen deficiency during menopause [1]. The main underlying cause of fractures in osteoporosis is increased bone fragility as a result of bone loss. Postmenopausal osteoporosis typically affects women according to their estrogen deficiency after menopause [2,3]. Therefore, postmenopausal administration of estrogen strengthens bone mineral density (BMD), and thereby decreases the occurrence of fractures associated with osteoporosis [4].
Prevention and therapy of osteoporosis are generally based on the antiresorptive agents or stimulators of bone formation. Antiresorptive drugs include calcium, estrogen, calcitonin and bisphosphonates [5-8]. Plant estrogens, known as phytoestrogens, were recommended for prevention of bone resorption [9]. However, the effects of phytoestrogens are relatively weak and transient, whereas synthetic estrogens can cause adverse effects such as endometrial carcinoma, venous thrombosis, and pulmonary embolism [4].
We recently demonstrated that Sigma Anti-bonding Molecule Calcium Carbonate (SAC) harvested from oyster shells improved BMD of ovariectomized rats by preventing bone turnover at a very low concentration (0.0012%) in drinking water [10]. Since SAC has a weak bonding force with other molecules, radicals or atoms, it is believed that the calcium in the SAC can easily be absorbed in the intestines, into the cells, and particularly into bones. In the present study, we performed repeated-dose toxicities of SAC to assess its feasibility as a potential candidate for the therapy or prevention of osteoporosis.
SAC [also named Biotechnical Calcium (BT-CAL) and Activated Ionic Calcium (AICAL); http://ntsresearch.com & http://www.btcal.ca] was obtained from NTS Research & Inc. (Coquitlam, BC, Canada). Six-week-old male ICR mice (n=10/group) were obtained from Daehan Biolink (Eumseong, Korea). Mice were housed in a room with a temperature of 23±2℃, relative humidity of 55±5%, and an alternating 12-h light (300 lux)/dark cycle with free access to feed and water. The animals were fed a standard rodent chow and purified water ad libitum. All animal experiments were approved and carried out in accordance with the Institutional Animal Care and Use Committee of Laboratory Animal Research Center at Chungbuk National University, Korea (Approval No.: CBNUA-160-1001-01).
The test was also performed according to the "Guidelines for Toxicity Tests of Drugs and Related Materials" provided by KFDA (Korea Food and Drug Administration, 1999). After 1 week of adaptation period, mice were given drinking water containing 0.006, 0.02 or 0.06% SAC for 4 weeks. Clinical signs and mortality were examined every day throughout the experimental period, and body weights and feed and water consumptions were recorded at proper intervals. The animals were fasted after the final administration for 24 h and sacrificed for blood biochemical and histopathological examinations.
Blood biochemical analysis was performed using an automatic analyzer (INTEGRA 400, Roche, Germany) for alanine transaminase (ALT), aspartate transaminase (AST), creatine phosphokinase (CPK), blood urea nitrogen (BUN), creatinine, and calcium (Ca) focusing on the cardiotoxicity and nephrotoxicity according to the clinical signs and necropsy findings of dead mice. For microscopic examination, kidneys and heart were formalin-fixed, paraffin-embedded, and stained with hematoxylin-eosin or Masson's trichrome.
The results were presented as mean±SD, and the significance of difference was analyzed using one-way analysis of variance followed by Tukey's test at the level of P<0.05. The analyses utilized SPSS statistical software version 12.0 for Windows (SPSS Inc., Chicago, IL, USA).
Mice fed SAC exhibited hyperactivity, alert response, and gasping and panting symptoms in the medium (0.02%) and high (0.06%) doses of SAC. In addition, the mice with behavioral abnormalities showed oliguria at the final stage. A low dose (0.006%) of SAC slightly reduced the body weight gain, and a medium dose (0.02%) significantly decreased the body weights (Figure 1A). Especially, a high dose (0.06%) of SAC caused severe body weight loss, leading to moribund condition and 70% death in 3 weeks. SAC induced decrease in the feed and water consumptions (Figures 1B and 1C) in a similar manner to the change in the animals' body weights, which may be due to the bad physical conditions.
SAC administration remarkably increased the kidney and heart weights in a dose-dependent manner, without affecting the liver weight (Table 1). In blood biochemical analysis, blood calcium level increased in parallel with the SAC concentration in the drinking water (Table 2). Interestingly, SAC feeding markedly increased the blood levels of AST, CPK, BUN, and creatinine, but not that of ALT, indicative of specific toxicities to the kidneys and heart as inferred from the clinical signs and increased organ weights.
In microscopic findings, large brown crystals were found in the renal tubules of the mice treated with all doses of SAC (0.006-0.06%), compared with the normal features in control animals (Figure 2). In addition to the crystals, eosinophilic, amorphous, and proteinaceous casts filled in the dilated renal tubules in medium and high doses (0.02-0.06%; Figure 2B) were observed, which may be related to the oliguria. Notably, trichrome staining revealed fibrosis of the cardiac muscles (Figure 3), indicative of a long-term overload as inferred from gasping and panting symptoms.
Calcium is the most important ion for the activation of cells. However, intracellular calcium ion is strictly controlled in nanomolar concentrations, because disruption of calcium homeostasis triggers cytotoxicity through activation of Ca++-dependent enzymes and cell death programs [11]. In the present study, blood calcium level increased in parallel with the concentration of SAC in the drinking water. Notably, feeding only 0.0012% of SAC in drinking water was similar to 0.07% of sericinconjugated calcium in the improvement of osteoporosis in ovariectomized rats [10,12], indicating a higher absorption rate of SAC. Such a high absorptive property of SAC may be due to its weak bonding force with other molecules.
However, as aforementioned, free calcium ions are harmful to cells and tissues. In the present study, SAC formed insoluble renal crystals. Although the chemical nature of the SAC crystals remains to be clarified, it is not excluded that loosely bound calcium ions were released from SAC and formed insoluble conjugate with acids including oxalic acid in the urine. It is well known that toxic acids such as oxalic acid, formic acid, and uric acid can be produced from diet (e.g., plant oxalates), environmental contaminants (e.g., ethylene glycol), and metabolic procedures [13]. Although a portion of the absorbed calcium may entered the bone tissues and strengthened them [10], overloaded calcium ions might be cleared via glomerular filtration. However, it is assumed that insoluble calcium crystals blocked and injured the renal tubules, leading to severe oliguria.
Moreover, heart is the organ fatally affected by ionic balance [14,15]. Cardiac muscle contraction is triggered by influx of sodium ion, followed by calcium ion, into the cytosol of myocytes [11]. Therefore, calcium overload induces over-stimulation (increased amplitude) and delays returning time to resting state, leading to bradycardia [15]. In this study, mice fed high concentrations of SAC displayed gasping and panting signs. Therefore, it is explained that continued calcium overload caused over-contraction of cardiomyocytes, hypertrophy, and finally myocardial fibrosis.
From this 4-week repeated-dose toxicity study, no observed adverse effect level (NOAEL) of SAC was estimated to be lower than 0.006%, in which dose considerable renal and cardiac toxicities were observed. Based on the water volumes consumed by the mice, 0.006, 0.02, and 0.06% SAC in the water match 11.5, 38.3, and 115.0 mg/kg, respectively. According to the dose translation from animal to human studies [16], the doses used in this study can be extrapolated to 0.94, 3.13, and 9.4 mg/kg in humans that may cause similar adverse effects. Furthermore, Food and Drug Administration (FDA) and Korea FDA suggest 1/100 of animal dosage as tolerable daily intake (TDI) for safety in humans.
In this 4-week repeated-dose toxicity study, SAC was confirmed to induce serious nephrotoxicity and cardiotoxicity at very low doses. Notably, a guideline of Korea FDA for functional food ingredients requires 3-month repeated-dose toxicity data. Therefore, more long-term animal experiment as well as dose-range finding study for humans must be performed before clinical application.
Acknowledgments
This work was supported by Priority Research Centers Program through the National Research Foundation of Korea (NRF) funded by the Ministry of Education, Science and Technology (2009-0094034).
References
1. Kaplan B, Hirsch M. Current approach to fracture prevention in postmenopausal osteoporosis. Clin Exp Obstet Gynecol. 2004; 31(4):251–255. PMID: 15672957.
2. Li M, Shen Y, Wronski TJ. Time course of femoral neck osteopenia in ovariectomized rats. Bone. 1997; 20(1):55–61. PMID: 8988348.


3. Ohta H, Makita K, Suda Y, Ikeda T, Masuzawa T, Nozawa S. Influence of oophorectomy on serum levels of sex steroids and bone metabolism and assessment of bone mineral density in lumbar trabecular bone by QCT-C value. J Bone Miner Res. 1992; 7(6):659–665. PMID: 1414484.


4. Riggs BL. Wyngaarden JB, editor. Osteoporosis. Textbook of Medicine. 1992. 19th ed. Philadelphia: Saunders;p. 1426–1430.
5. Fraser LA, Vogt KN, Adachi JD, Thabane L. Fracture risk associated with continuation versus discontinuation of bisphosphonates after 5 years of therapy in patients with primary osteoporosis: a systematic review and meta-analysis. Ther Clin Risk Manag. 2011; 7:157–166. PMID: 21691586.


6. Loke YK, Jeevanantham V, Singh S. Bisphosphonates and atrial fibrillation: systematic review and meta-analysis. Drug Saf. 2009; 32(3):219–228. PMID: 19338379.
7. Honig S. Osteoporosis - new treatments and updates. Bull NYU Hosp Jt Dis. 2010; 68(3):166–170. PMID: 20969546.
8. Muratore M, Quarta E, Grimaldi A, Calcagnile F, Quarta L. Clinical utility of clodronate in the prevention and management of osteoporosis in patients intolerant of oral bisphosphonates. Drug Des Devel Ther. 2011; 5:445–454.


9. Potter SM, Baum JA, Teng H, Stillman RJ, Shay NF, Erdman JW Jr. Soy protein and isoflavones: their effects on blood lipids and bone density in postmenopausal women. Am J Clin Nutr. 1998; 68(6 Suppl):1375S–1379S. PMID: 9848502.


10. Choi SY, Park D, Yang G, Lee SH, Bae DK, Hwang SY, Lee PK, Kim YB, Kim IH, Kang HG. Effects of Sigma Anti-bonding Molecule Calcium Carbonate on bone turnover and calcium balance in ovariectomized rats. Lab Anim Res. 2011; 27(4):301–307. PMID: 22232638.


11. Klaassen CD. Casarett & Doull's Toxicology: The Basic Science of Poisons. 2001. 6th ed. New York: McGraw-Hill;p. 35–82.
12. Park D, Choi SY, Yang YH, Hwang SY, Yeon S, Lee JY, Kim IH, Kim YB, Choi EK, Kang HG. Anti-osteoporotic effects of sericincalcium conjugate in ovariectomized rats. J Biomed Res. 2011; 12:91–101.
13. Osweiler GD. Toxicology. 1996. Hoboken: Wiley-Blackwell.
14. Greiser M, Lederer WJ, Schotten U. Alterations of atrial Ca(2+) handling as cause and consequence of atrial fibrillation. Cardiovasc Res. 2011; 89(4):722–733. PMID: 21159669.


15. Kelly A, Elliott EB, Matsuda R, Kaneko N, Smith GL, Loughrey CM. The effect of K201 on isolated working rabbit heart mechanical function during pharmacologically induced Ca2+ overload. Br J Pharmacol. 2012; 165(4b):1068–1083. PMID: 21658026.
16. Reagan-Shaw S, Nihal M, Ahmad N. Dose translation from animal to human studies revisited. FASEB J. 2008; 22(3):659–661. PMID: 17942826.
Figure 1
Change in body weights (A) and daily feed (B) and water (C) consumptions during feeding Sigma Anti-bonding Molecule Calcium Carbonate (SAC). •, water alone; ▾, 0.006% SAC; ▪, 0.02% SAC; ♦, 0.06% SAC.

Figure 2
Representative findings of the kidneys. Note the brown crystals (arrows) in the renal tubules filled with eosinophilic contents in a mouse fed Sigma Anti-bonding Molecule Calcium Carbonate (0.02%) (B), compared with the normal features in a normal animal (A). Hematoxylin-eosin staining.

Figure 3
Representative findings of the hearts. Note the fibrosis (stained blue) in the cardiac muscles in a mouse fed Sigma Antibonding Molecule Calcium Carbonate (0.02%) (B), compared with the normal features in a normal animal (A). Masson's trichrome staining.
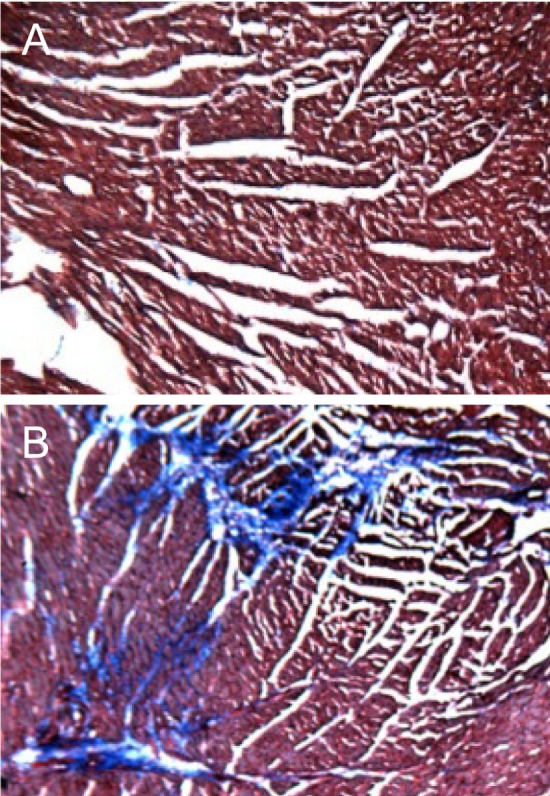