Abstract
Ginkgo biloba extract (EGb 761) exerts a neuroprotective effect against ischemic brain injury through an anti-apoptotic mechanism. Parvalbumin is a calcium buffering protein that plays an important role in modulating intracellular calcium concentration and regulating apoptotic cell death. The aim of this study was to investigate whether EGb 761 affects parvalbumin expression in cerebral ischemic injury. Adult male Sprague-Dawley rats were treated with vehicle or EGb 761 (100 mg/kg) prior to middle cerebral artery occlusion (MCAO) and cerebral cortex tissues were collected 24 h after MCAO. A proteomic approach revealed a reduction in parvalbumin expression in the vehicle-treated animals, whereas EGb 761 pretreatment attenuates the ischemic injury-induced decrease in parvalbumin expression. RT-PCR and Western blot analyses clearly confirmed the fact that EGb 761 prevents the injury-induced decrease in parvalbumin. Moreover, the results of immunohistochemical staining showed that the number of parvalbumin-positive cells was lower in vehicle-treated animals than in sham-operated animals, and EGb 761 averted this decrease. Thus, these results suggest that the maintenance of parvalbumin expression is associated with the neuroprotective function of EGb 761 against neuronal damage induced by ischemia.
Ginkgo biloba extract (EGb 761) is derived from the green leaves of Ginkgo biloba and used as a traditional medicine for various purposes [1]. It consists of flavones glycosides and terpene lactones and has an antioxidant effect in ischemic brain injury [2,3]. EGb 761 also provides pharmacologic benefits in Alzheimer's disease and vascular disorders of the brain [4-6], acts as a scavenger of reactive oxygen species (ROS), and reduces neural cell damage induced by ischemia [7,8]. Moreover, previous studies clearly reported the neuroprotective effect of EGb 761 during focal cerebral ischemia induced by middle cerebral artery occlusion (MCAO) [9,10].
Parvalbumin belongs to the family of EF-hand Ca2+-binding proteins, and acts as a calcium sensor protein or calcium buffer protein because its critical function is binding to Ca2+ molecules released into the cytoplasm [8,11,12]. Thus, parvalbumin plays an essential role in maintaining calcium homeostasis of the central nervous system by decreasing the intracellular Ca2+ concentration [12]. An increase in intracellular Ca2+ concentration leads to neuronal cell death and consequently central nervous system disorder [13]. Parvalbumin is thought to exert a neuroprotective effect by preventing increases in intracellular Ca2+. Although previous studies have attempted to elucidate the physiologic mechanism underlying the neuroprotective effect of EGb 761, more information is needed in order to fully define the complete neuroprotective mechanism. We hypothesized that EGb 761 may mediate parvalbumin expression in ischemic brain injury. However, little data is available regarding the effects of EGb 761 on parvalbumin expression levels in neuronal cell injury. We investigated the effect of EGb 761 on parvalbumin during cerebral ischemic injury.
All animal experiments were followed a protocol approved by the Institutional Animal Care and Use Committee (IACUC) at the Gyeongsang National University. Male Sprague-Dawley rats (210-230 g, n=48) were purchased from Samtako Co. (Animal Breeding Center, Osan, Korea). Animals were maintained on 12 h light/dark cycle with free access to food and water. Animals were randomly divided into following three groups: sham-operated group, vehicle-treated group, and EGb 761-treated group (n=16 per group). Animals were intraperitoneally administrated EGb 761 (100 mg/kg, Yuyu, Seoul, Korea) in EGb 761-treated group and vehicle in the vehicle-treated group at 1 h before the onset of MCAO [9].
Focal cerebral ischemia was induced by the method of MCAO [14]. Before MCAO operation, animals were anesthetized with sodium pentobarbital (30 mg/kg). a midline incision was made and The right common carotid, external carotid artery and internal carotid arteries were exposed. The external carotid artery was tied and cut. A 4/0 monofilament nylon with its tip rounded by heating over a flame was advanced from the external carotid artery into lumen of the internal carotid artery, thereby occluding blood flow through the middle cerebral artery. At 24 h after the onset of permanent occlusion, brain tissues were removed.
Brains were cut into coronal slices of 2 mm in thickness. These slices were stained for 20 min in a 2% triphenyltetrazolium chloride (TTC; Sigma, St. Louis, MO, USA) and fixed in 10% formalin. The stained slices were photographed by a Nikon CoolPIX990 digital camera (Nikon, Tokyo, Japan) and measured for the ischemic lesion by Image-ProPlus 4.0 software (Media Cybernetics, Silver Spring, MD, USA). The ischemic lesion percentage of each slice was calculated by the ratio of the infarction area to the whole slice area.
The right cerebral cortices were dissolved in lysis buffer (8 M urea, 4% CHAPS, ampholytes, and 40 mM Tris-HCl). After centrifuge at 16,000 g for 20 min at 4℃, the total protein concentration was determined using the Bradford method (Bio-Rad, Hercules, CA, USA) according to the manufacturer's protocol. Briefly, prior to isoelectric focusing (IEF), the immobilized pH gradients (IPG, pH 4-7, pH 6-9, 17 cm, Bio-Rad) gel strips were re-hydrated in buffer (8 M urea, 2% CHAPS, 20 mM DTT, 0.5% IPG buffer, bromophenol blue) for 13 h. The protein samples (100 µg) were loaded on IPG strips. IEF was followed a multi-step protocol: 250 V (15 min), 10000 V (3 h), and then 10000 V to 50000 V using Protean IEF Cell (Bio-Rad). After equilibration of the isoelectric focusing strips, IPG strips were applied onto gradient gels (7.5~17.5%) and electrophoresed at 10 mA for 10 h using Protein-II XI electrophoresis equipment (Bio-Rad) at 10℃. The gels were fixed in a solution (12% acetic acid, 50% methanol) for 2 h, washed twice with 50% ethanol for 20 min, and then sensitized in 0.2% sodium thiosulfate for 1 min. The gels were washed with deionized water, incubated in a silver solution (0.2% silver nitrate, 0.75 mL/L formaldehyde) for 20 min. The gel was developed in a solution (0.2% sodium carbonate, 0.5 mL/L formaldehyde) and the reaction was stopped by adding 1% acetic acid. The silver stained gels were scanned by Agfar ARCUS 1200™ (Agfar-Gevaert, Mortsel, Belgium) and analyzed by a standard protocol for PDQuest software (Bio-Rad). The analyzed data were expressed as mean±S.E.M. Relative intensity of spots in each group was analyzed and compared by Student's t-test. Alteration of protein expression was identified in vehiclel- and EGb 761-treated animals. The gel pieces containing the desired protein spots were manually cut out from the gels and processed for MALDI-TOF.ed and distained. Detained and dehydrated gel pieces were incubated with trypsin-containing digestion buffer for 24 h at 37℃. The peptides were extracted and analyzed in a Voyager-DE™ STR biospectrometry workstation (Applied Biosystem, Forster city, CA, USA) for MALDI-TOF mass spectrometric analysis. the acquired peaks were identified using search programs MS-Fit and ProFound. SWISS-PROT and NCBI were used as the protein sequence databases.
RNA was extracted using Trizol reagent according to the manufacturer's instruction (Invitrogen, Carlsbad, CA, USA). Total RNA (1 µg) was reverse transcribed using superscript III first-strand system for RT-PCR (Invitrogen) based on the manufacturer's protocol. The primers sequences of parvalbumin are 5'-AAGAGTGCGGATGATGTGAAG-3' (forward primer) and 5'-AGCCATCAGCGTCTTTGTTT-3' (reverse primer). The primers sequences of actin are 5'-GGGTCAGAAGGACTCCTACG-3' (forward primer) and 5'-GGTCTCAAACATGATCTGGG-3' (reverse primer). The PCR reaction was carried out as followed: 5 min at 94℃, 30 sec at 94℃, 30 sec at 54℃, 1 min at 72℃ and 10 min at 72℃. The samples were amplified 30 cycles. PCR product was run in a 1% agarose gel and visualized under UV light.
Western blot analysis were performed as previously described [10]. The proteins of right cerebral cortex were extracted by homogenization in buffer [1% Triton X-100, 1 mM EDTA in 1×PBS (pH 7.4)] containing 200 µM phenylmethylsulfonyl fluoride and 10 µM leupeptin. The lysates were centrifuged at 12,000 rpm for 20 min at 4℃. The supernatants were collected and the protein concentration of each lysate was determined using the bicinchoninic acid (BCA) kit (Pierce, Rockford, IL, USA). Total protein (30 µg) was run on to 10% SDS-polyacrylamide gel and subsequently transferred to a poly-vinylidene fluoride (PVDF) membranes (Millipore, Billerica, MA, USA) and membranes were blocked with 5% skim milk in 0.1% Tween-20 (TBST). After washing membranes with TBST, membranes were incubated with the following antibodies: a rabbit polyclonal anti-rat parvalbumin IgG (diluted 1:1000, Cell Signaling Technology, Beverly, MA, USA) and a mouse monoclonal anti-rat actin IgG (diluted 1:1000, Santa Cruz Biotechnology, Santa Cruz, CA, USA). The membrane was incubated with secondary antibody (1:5000, Pierce, Rockford, IL, USA) and the ECL Western blot analysis system (Amersham Pharmacia Biotech, Piscataway, NJ, USA) was used for detection according to the manufacturer's protocol.
Brains were fixed in 4% paraformaldehyde in 0.1M phosphate-buffered saline (PBS, pH 7.4) and embedded in paraffin. Three sections were selected in each group for the immunohistochemical staining. Sections were blocked with 1% normal goat serum in PBS for 1 h and incubated with a rabbit polyclonal anti-rat parvalbumin IgG (diluted 1:200, Cell Signaling Technology, Beverly, MA, USA) at 4℃ for 15 h. After washing with PBS, sections were incubated with biotin-conjugated goat anti-rabbit IgG (1:200 in PBS) for 1 h, followed by incubation with an avidin-biotin-peroxidase complex for 1 h from a Vector ABC Elite kit (Vector Laboratories Inc., Burlingame, CA, USA), incubated with DAB solution with 0.03% hydrogen peroxidase. Sections were counterstained with hematoxylin, dehydrated in graded alcohol. For the positive cell counting, three fields for cortical region were selected from right cerebral cortex. The total cell number and parvalbumin-positive cell number were obtained in each field. The index of positive cells was described as the percentage of the number of parvalbumin-positive cells to the total number of cells in each field.
All data are expressed as mean±S.E.M. The intensity analysis of Western blot analysis was carried out using SigmaGel 1.0 (Jandel Scientific, San Rafael, CA, USA) and SigmaPlot 4.0 (SPSS Inc., Point Richmond, CA, USA). The results in each group were compared by one-way analysis of variance (ANOVA) followed by Student's t-test. The difference for comparison was considered significant at *P<0.05.
We confirmed the neuroprotective effect of EGb 761 against ischemic brain injury using TTC staining (Figure 1). EGb 761 treatment significantly reduces infarct volume, compared with the vehicle-treated animals. Our previous study showed the EGb 761 regulates various proteins including peroxiredoxin-2, protein phosphatase 2A subunit B, and collapsing response mediator protein 2 in cerebral ischemic injury [15]. In this study, a proteomic approach additionally demonstrated that parvalbumin protein spots were differentially expressed in the cerebral cortices of vehicle- and EGb 761-treated animals in which brain injury had been induced by MCAO (Figure 2). The peptide mass of parvalbumin is 5/103 and the sequence of this protein is 40%. The intensity of parvalbumin protein spots were decreased in vehicle-treated animals, whereas this decrease was prevented in EGb-761-treated animals. The parvalbumin levels were 0.49±0.02 and 0.83±0.02 in the vehicle- and EGb 761-treated animals, respectively. RT-PCR and Western blot analyses clearly demonstrated differences in parvalbumin levels between the vehicle- and EGb 761-treated animals. Parvalbumin transcript levels were 0.28±0.03 and 0.87±0.02 in the vehicle- and EGb 761-treated animals during MCAO, respectively (Figure 3). Parvalbumin expression was significantly decreased in vehicle-treated animals, whereas EGb-761 treatment attenuated the ischemic injury-induced decrease in parvalbumin expression. Parvalbumin protein levels were 0.62±0.03 and 0.92±0.02 in the cerebral cortices of vehicle- and EGb 761-treated animals during MCAO, respectively (Figure 4). Immunohistochemical staining showed that the number of parvalbumin-positive cells was lower in vehicle-treated animals than in sham-operated animals, and EGb 761 prevented the injury-induced decrease in parvalbumin-positive cells (Figure 5). The number of parvalbumin positive cells significantly decreased in vehicle-treated animal, whereas EGb761 treatment attenuates the injury-induced decrease of parvalbumin positive cells. The index of positive cells were 7.58±1.05% and 25.36±1.35% in vehicle- and EGb 761-treated animals during MCAO (Figure 5).
EGb 761 has a neuroprotective effect against beta-amyloid toxicity and oxidative stress [16,17]. Our previous study clearly showed the neuroprotective effect of EGb 761 against focal cerebral ischemia induced by MCAO [14]. EGb 761 exerts a neuroprotective effect in various neurological disorders such as ischemia, Alzheimer's, and depression [5,18,19]. Moreover, we demonstrated that EGb 761 prevents neuronal cells death from ischemic brain injury through the specific modulation of various proteins including peroxiredoxin-2, protein phosphatase 2A subunit B, collapsing response mediator protein 2, adenosylhomocyteinase, pyruvate kinase isoenzyme, and isocitrate dehydrogenase. This study additionally showed the decrease of parvalbumin expression during MCAO. Parvalbumin is a calcium buffering protein that counteracts neuronal cells damage and exerts a neuroprotective effect by buffering intracellular calcium [20]. The intracellular Ca2+ concentration increases in ischemic brain injury, and this increase in Ca2+ induces lethal effects such as free radical production, which ultimately lead to neuronal cell degeneration and death [13,21]. Because an excessive intracellular calcium concentration leads to the initiation of neuronal cell death, the regulation of calcium concentration is critical for ensuring the survival of neuronal cells [22,23].
The proteomic approach revealed in this study revealed that EGb 761 prevents the brain injury-induced decrease in parvalbumin expression during MCAO. The maintenance of parvalbumin levels may inhibit the excessive increase in the intracellular calcium concentration that is induced by ischemia. Calcium binding proteins play an important role in the destiny of neuronal cells because they act as calcium chelators and reduce intracellular calcium concentration [24,25]. The rise of intracellular calcium activates calcium-sensitive protein digesting enzymes and leads to cellular degradation. Moreover, amyloid-beta induces the disruption of neuronal Ca2+ homeostasis and the generation of ROS, while these events are blocked by EGb 761 [7]. Western blot analysis and immunohistochemical staining clearly confirmed that cerebral ischemic injury decreases parvalbumin expression, whereas EGb 761 attenuates this injury-induced decrease in parvalbumin expression. Our results demonstrate that EGb 761 modulates parvalbumin expression in ischemic brain injury and consequently contributes to the regulation of intracellular calcium levels. Although further research is required to explain the relationship between EGb 761 and parvalbumin, these results suggest that EGb 761 maintains parvalbumin levels, thereby maintaining intracellular calcium levels and attenuating neuronal cell death. In conclusion, our findings suggest that EGb 761 attenuates neuronal cell death by modulating the expression of parvalbumin.
Figures and Tables
Figure 1
TTC staining of brain slices in vehicle-treated, EGb 761-treated, and sham-operated animals. The ischemic area remained white, while the intact area was stained red. The percentage of ischemic lesion area was calculated as lesion area/whole brain section area. Data (n=4) are represented as mean±S.E.M. *P<0.05.
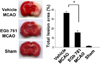
Figure 2
Parvalbumin protein spots identified by MALDI-TOF in the cerebral cortices of vehicle-treated, EGb 761-treated, and sham-operated animals. Circles indicate the protein spots. The intensity of the spots was measured using PDQuest software. The intensity ratio is defined as the spot intensity of vehicle- or EGb 761-treated animals to the spot intensity of the sham group. Data (n=4) are mean±S.E.M. *P<0.05. Mw and IP indicate molecular weight and isoelectric point, respectively.
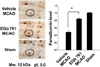
Figure 3
RT-PCR analysis of parvalbumin in the cerebral cortices of vehicle-treated, EGb 761-treated, and sham-operated animals. Each lane represents an individual experimental animal. The results of densitometric analysis are presented as the ratio of the intensity of parvalbumin to the intensity of actin. Data (n=4) are mean±S.E.M. *P<0.05.
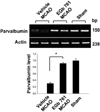
Figure 4
Western blot analysis of parvalbumin in the cerebral cortices of vehicle-treated, EGb 761-treated, and sham-operated animals. Each lane represents an individual experimental animal. The results of densitometric analysis are presented as the ratio of the intensity of parvalbumin to the intensity of actin. Molecular weight markers (kDa) are depicted on the left. Data (n=4) are mean±S.E.M. *P<0.05.
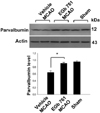
Figure 5
Immunostaining of parvalbumin in the cerebral cortices of vehicle-treated, EGb 761-treated, and sham-operated animals. Arrows indicate positive cells. The index of positive cells indicates the percentage of the number of parvalbumin-positive cells to the total number of cells. Scale bar=100 µm. Data (n=4) are represented as mean±S.E.M. *P<0.05.
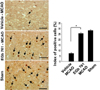
Acknowledgments
This work was partially supported by the National Research Foundation of Korea (NRF) grant funded by the Korea government (MEST) (No.2010-0007881) and partially supported by a grant from the Next-Generation BioGreen 21 Program (No. PJ008075), RDA, Republic of Korea.
References
1. Shah ZA, Nada SE, Dore S. Heme oxygenase 1, beneficial role in permanent ischemic stroke and in Gingko biloba (EGb 761) neuroprotection. Neuroscience. 2011. 180:248–255.
2. Seif-El-Nasr M, El-Fattah AA. Lipid peroxide, phospholipids, glutathione levels and superoxide dismutase activity in rat brain after ischaemia: effect of ginkgo biloba extract. Pharmacol Res. 1995. 32(5):273–278.
3. Calapai G, Crupi A, Firenzuoli F, Marciano MC, Squadrito F, Inferrera G, Parisi A, Rizzo A, Crisafulli C, Fiore A, Caputi AP. Neuroprotective effects of Ginkgo biloba extract in brain ischemia are mediated by inhibition of nitric oxide synthesis. Life Sci. 2000. 67(22):2673–2683.
4. Maurer K, Ihl R, Dierks T, Frolich L. Clinical efficacy of Ginkgo biloba special extract EGb 761 in dementia of the Alzheimer type. J Psychiatr Res. 1997. 31(6):645–655.
5. Oken BS, Storzbach DM, Kaye JA. The efficacy of Ginkgo biloba on cognitive function in Alzheimer disease. Arch Neurol. 1998. 55(11):1409–1415.
6. Kotil K, Uyar R, Bilge T, Ton T, Kucukhuseyin C, Koldas M, Atay F. Investigation of the dose-dependent antivasospasmic effect of Ginkgo biloba extract (EGb 761) in experimental subarachnoid hemorrhage. J Clin Neurosci. 2008. 15(12):1382–1386.
7. Shi C, Zhao L, Zhu B, Li Q, Yew DT, Yao Z, Xu J. Protective effects of Ginkgo biloba extract (EGb761) and its constituents quercetin and ginkgolide B against beta-amyloid peptide-induced toxicity in SH-SY5Y cells. Chem Biol Interact. 2009. 181(1):115–123.
8. Chard PS, Bleakman D, Christakos S, Fullmer CS, Miller RJ. Calcium buffering properties of calbindin D28k and parvalbumin in rat sensory neurones. J Physiol. 1993. 472:341–357.
9. Lee EJ, Chen HY, Wu TS, Chen TY, Ayoub IA, Maynard KI. Acute administration of Ginkgo biloba extract (EGb 761) affords neuroprotection against permanent and transient focal cerebral ischemia in Sprague-Dawley rats. J Neurosci Res. 2002. 68(5):636–645.
10. Koh PO. ngko biloba extract (EGb 761) prevents increase of Bad-Bcl-XL interaction following cerebral ischemia. Am J Chin Med. 2009. 37(5):867–876.
11. Van Den Bosch L, Schwaller B, Vleminckx V, Meijers B, Stork S, Ruehlicke T, Van Houtte E, Klaassen H, Celio MR, Missiaen L, Robberecht W, Berchtold MW. Protective effect of parvalbumin on excitotoxic motor neuron death. Exp Neurol. 2002. 174(2):150–161.
12. Girard F, Meszar Z, Marti C, Davis FP, Celio M. Gene expression analysis in the parvalbumin-immunoreactive PV1 nucleus of the mouse lateral hypothalamus. Eur J Neurosci. 2011. 34(12):1934–1943.
13. Starkov AA, Chinopoulos C, Fiskum G. Mitochondrial calcium and oxidative stress as mediators of ischemic brain injury. Cell Calcium. 2004. 36(3-4):257–264.
14. Longa EZ, Weinstein PR, Carlson S, Cummins R. Reversible middle cerebral artery occlusion without craniectomy in rats. Stroke. 1989. 20(1):84–91.
15. Koh PO. Identification of proteins differentially expressed in cerebral cortexes of Ginkgo biloba extract (EGb761)-treated rats in a middle cerebral artery occlusion model--a proteomics approach. Am J Chin Med. 2011. 39(2):315–324.
16. Bastianetto S, Ramassamy C, Dore S, Christen Y, Poirier J, Quirion R. The Ginkgo biloba extract (EGb 761) protects hippocampal neurons against cell death induced by beta-amyloid. Eur J Neurosci. 2000. 12(6):1882–1890.
17. Luo Y, Smith JV, Paramasivam V, Burdick A, Curry KJ, Buford JP, Khan I, Netzer WJ, Xu H, Butko P. Inhibition of amyloid-beta aggregation and caspase-3 activation by the Ginkgo biloba extract EGb761. Proc Natl Acad Sci USA. 2002. 99(19):12197–12202.
18. Pierre S, Jamme I, Droy-Lefaix MT, Nouvelot A, Maixent JM. Ginkgo biloba extract (EGb 761) protects Na,K-ATPase activity during cerebral ischemia in mice. Neuroreport. 1999. 10(1):47–51.
19. Rojas P, Serrano-Garcia N, Medina-Campos ON, Pedraza-Chaverri J, Ogren SO, Rojas C. Antidepressant-like effect of a Ginkgo biloba extract (EGb761) in the mouse forced swimming test: role of oxidative stress. Neurochem Int. 2011. 59(5):628–636.
20. Nitsch C, Scotti A, Sommacal A, Kalt G. GABAergic hippocampal neurons resistant to ischemia-induced neuronal death contain the Ca2(+)-binding protein parvalbumin. Neurosci Lett. 1989. 105(3):263–268.
21. Hayashi T, Abe K. Ischemic neuronal cell death and organellae damage. Neurol Res. 2004. 26(8):827–834.
22. Carafoli E. Intracellular calcium homeostasis. Annu Rev Biochem. 1987. 56:395–433.
23. Miller RJ. The control of neuronal Ca2+ homeostasis. Prog Neurobiol. 1991. 37(3):255–285.
24. Heizmann CW, Braun K. Changes in Ca(2+)-binding proteins in human neurodegenerative disorders. Trends Neurosci. 1992. 15(7):259–264.
25. Siesjo BK. Historical overview. Calcium, ischemia, and death of brain cells. Ann N Y Acad Sci. 1988. 522:638–661.