Abstract
Estrogens, a class of steroid hormones, regulate the growth, development, and physiology of the human reproductive system. Estrogens also involve in the neuroendocrine, skeletal, adipogenesis, and cardiovascular systems. Estrogen signaling pathways are selectively stimulated or inhibited depending on a balance between the activities of estrogen receptor (ER) α or ERβ in target organs. ERs belong to the steroid hormone superfamily of nuclear receptors, which act as transcription factors after binding to estrogen. The gene expression regulation by ERs is to modulate biological activities, such as reproductive organ development, bone modeling, cardiovascular system functioning, metabolism, and behavior in both females and males. Understanding of the general physiological roles of ERs has been gained when estrogen levels were ablated by ovariectomy and then replenished by treatment with exogenous estrogen. This technique is not sufficient to fully determine the exact function of estrogen signaling in general processes in living tissues. However, a transgenic mouse model has been useful to study gene-specific functions. ERα and ERβ have different biological functions, and knockout and transgenic animal models have distinct phenotypes. Analysis of ERα and ERβ function using knockout mouse models has identified the roles of estrogen signaling in general physiologic processes. Although transgenic mouse models do not always produce consistent results, they are the useful for studying the functions of these genes under specific pathological conditions.
Estrogen receptors (ERs) belong to the steroid hormone superfamily of nuclear receptors (NRs) [1,2]. Other types of steroid receptors among the NRs include the estrogen-related receptors (EER), progesterone receptors (PR), androgen receptors (AR), glucocorticoid, and mineral corticoid receptors. Steroid receptors (SRs), act as ligand-dependent transcription factors, and their activity is associated with the cell cycle [3]. Analyses of the association between steroid receptor activity and the cell cycle revealed that SRs, especially ERs, regulate cell proliferation [4]. Moreover, estrogen is an important sex hormone produced primarily in the ovaries in females and testes in males. This steroid hormone regulates the growth, development, and physiology of the reproductive system in humans [5]. Estrogen also affects the neuroendocrine, skeletal, adipogenic, and cardiovascular systems [6,7]. The biological functions of estrogen are mediated by binding to the ERs: estrogen receptor alpha (ERα) and estrogen receptor beta (ERβ). Estrogen signaling is selectively stimulated or inhibited depending upon a balance between ERα and ERβ activities in target organs. ERα was cloned from human breast cancer MCF-7 cells in 1986 and ERβ was cloned from rat prostate in 1996 [8].
ERα and ERβ are encoded by distinct genes located on different chromosomes. The human ERα gene is located on chromosome 6 while the ERβ gene is on chromosome 14 [9]. The full-length human ERα protein has 595 amino acids and a molecular size of 66 kDa while the full-length human ERβ protein has 530 amino acids and a molecular size of 54 kDa. Similar to other NRs, ERs have five domains with distinct functions [10]. The N-terminal of the A/B domains of ERs consist of activation function 1 (AF1), which contributes to the transcriptional activity of ERs and is an essential domain for interaction with co-regulators. AF1 is the least conserved region with only 30% identity between ERα and ERβ. Functional studies have shown that ERβ has low levels of AF1 activity. The A/B domains also contain amino acids that are targets of post-transcriptional modifications including splicing to stimulate AF1 activity [9]. The C domain encodes a centrally located DNA binding domain (DBD) essential for sequence-specific binging of ERs to DNA and regulating the expression of target genes [11]. The D domain, a hinge region, includes amino acid sequences that stimulate nuclear localization signaling and facilitate post-translational modification of ERs, resulting in the activation of ER signaling in cells. Finally, the E/F domain, located in the C-terminal region, contains a ligand-binding domain (LBD) that serves as an interaction site with co-regulators and ligand-dependent activation function 2 (AF2). AF1 and AF2 control the transcriptional regulatory activity of ERs because activation of ERs are stimulated during cellular responses to the environment [12]. The E/F domains of ERα and ERβ share a 53% sequence identity and affect cellular responses through ligand-dependent ER activation. The F domain also affects the activity of ERα and ERβ. The differences between the F domain of the ERs may contribute to the ability of ERs to selectively control transcriptional activities of specific target genes [10].
In the absence of hormones, ERs remain in an inactivation form due to association with heat shock protein 90 (Hsp90). Hsp90 regulates various processes in eukaryotic cells including protein stabilization, binding affinity of receptors to ligands, and signaling cascades [13]. Hsp90 inhibits the degradation of unbound ERs and prevents inactivated ERs from binding ligand [14]. After binding to ligand, ERs are phosphorylated, form homo- or heterodimers, and then translocate into the nucleus [15]. ERs modulate the transcription of target genes by binding to estrogen response elements (EREs) in the DNA sequence [16]. Binding of ERs to EREs promotes DNA bending and looping, thereby permitting interaction with the transcriptional machinery and co-regulator proteins. These co-regulators include co-activators, co-repressors, co-integrators, histone acetyltransferases and deacetylases, and general transcriptional factors [12]. ER/co-regulator complexes act specifically on target genes in particular organs according to extracellular stimuli [17]. Analysis of ERα and ERβ tissue distribution suggests that ERs have high specificity on the target tissue [18]. ERα is highly expressed in the uterus, prostate stroma, ovarian theca cells, Leydig cells in testes, epididymis, breast, and liver [19]. ERβ is highly expressed in prostate epithelium, testes, ovarian granulosa cells, bone marrow, and brain [20]. As mentioned above, ERα and ERβ have different downstream transcriptional activities, resulting in their tissue-specific biological actions [21].
ERα and ERβ have different biological functions. Not surprisingly, eliminating the expression of either factor results in distinct phenotypes in mice. Analysis of ERα and ERβ using knockout mouse models has demonstrated the general roles of estrogen signaling. ERα (αERKO) and ERβ (βERKO) knockout mice have been used to examine the various roles of ER signaling [22,23].
Rodent reproductive physiology is associated with steroid hormones, including estrogen. Estrogen is basically known for its effect on the reproduction systems of both females and males [24]. Understanding of the general physiological roles of estrogen has resulted in ovariectomies to ablate estrogen production or restoring estrogen levels with exogenous E2 [25]. Currently, the exact function of estrogen signaling in biological processes is unclear. However, transgenic mice have been useful for discovering gene-specific ER functions [26]. Many experiments with ERKO mice were able to elucidate ERs function in specific pathological conditions, absence of ER expression. [27,28]. Surprisingly, both male and female αERKO mice are infertile, whereas fertility differs among βERKO mice according to gender. Female βERKO mice have reduced fertility while males have normal fertility [29].
Generally, maturation of the reproductive organs promoted by E2 is important for successful pregnancy and appropriate sexual behavior [30]. Infertility in αERKO female mice is due to a failure to respond to estrogen in the uterus, which is a central organ for reproduction and pregnancy [31]. ERα is essential for uterine maturation but not development [32]. Female αERKO mice have a decreased number of glands in the endometrium, whereas female βERKO mice have normal uterine and vaginal tissues despite their reduced fertility rates. Another reason for infertility in the knockout mice is abnormal ovarian function. The ovary is affected by changes in estrogen levels that induce cyclic ovulation [33]. Estrogen stimulates follicular responses to gonadtropins and activities of enzymes such as aromatase for steroidogenesis [34]. The synthesis and secretion to E2 is known for action of follicular stimulating hormone (FSH) on granulosa cells and luteinizing hormone (LH) on theca cell. Both FSH and LH maintain the menstrual cycle and affect ovulation [35]. An abnormal ovarian phenotype causes infertility in αERKO mice and reduced fertility in βERKO mice. αERKO mice appear incapable of ovulation as their follicles fail to differentiate. Ovarian dysregulation is also observed in βERKO mice including an increased number of unruptured follicles and reduced numbers of oocytes [36].
In mammals, the mammary gland is important for feeding offspring through the production milk. Development of the mammary gland involves ductal elongation from a nipple and formation of branches of the glands with fat pads. During the estrous cycle, mammary gland development proceeds in a manner similar to that observed during pregnancy through the actions of ERs and PRs [37]. Since αERKO female mice cannot ovulate, mammary glands of these mice do not develop beyond an immature state, and only contain rudimentary ducts characteristic of embryonic and fetal stages. Insufficient maturation of the mammary gland is caused by a loss of estrogen signaling due to the absence of ER expression. In female αERKO mice, the release of prolactin from the anterior pituitary gland is reduced and breast tissue for lactation is structurally impaired. However, the βERKO female have ability to nurse offspring since they develop normal mammary glands capable of lactation [38].
The balance of two steroid hormones, androgen and estrogen, plays an important role in male reproductive organs, and is essential for normal prostate development and prostatic homeostasis [39]. However, estrogen signaling also plays a role in maintaining the male reproductive system. ERα is expressed in prostatic tissues during fetal development. ERβ expression is initiated after ERα during adulthood. ERs regulate cell growth and differentiation in adult tissues [40]. Thus, an appropriate balance between ERα and ERβ is required for normal development of male reproductive tissues. [39]. In male αERKO mice, the testes are smaller than those in wild-type animals and the fertility rates are significantly reduced [41]. Low fertility in αERKO male mice is due to reduced sperm counts and low sperm quality. In contrast, the βERKO males have been shown to produce a sufficient number of sperm to maintain fertility. These findings imply that ERα is more important than ERβ for reproduction system development and sperm maturation in male mice.
Bone plays a role in the support of soft organs and maintains calcium level homeostasis in blood. This tissue is continuously remodeled to maintain a suitable length and density for the body. Calcium, vitamin D, and steroid hormones such as estrogen are critical for bone homeostasis [42,43]. These factors control gene expression in bone cells including osteoblasts, osteoclasts, and osteocytes [44]. In addition, various factors are regulated by estrogen during the formation the bone, which modulate osteoblast differentiation and the development of osteoporosis [45]. Estrogen is known to stimulate the release of growth factors such as insulin-like growth factor 1 (IGF-1) that influence bone resorption. ERKO mice have been very valuable for elucidating the association between mechanisms underlying bone functions and ER signaling [46]. ERα is expressed at low levels in bone. In αERKO mice, bone length and size are significantly decreased compare to normal mice. The bones of male αERKO mice also show a reduction in mineral density [47]. However, skeletons of βERKO mice are phenotypically identical to those of wild-type animals, indicating the low importance of ERα in osteoporosis progression [48].
The rate of cardiovascular system disease for women increases with age after menopause. Although the direct effect of estrogen on the cardiovascular system is unclear, reduced estrogen levels are associated with the disruption of lipid or glucose regulation [49]. The ability of estrogen to decrease cholesterol levels is due to the elimination of low-density lipoprotein (LDL) from blood circulation. In addition, various studies have recently demonstrated that estrogen affects blood vessel structure [5,50]. ERs are expressed in endothelial and smooth muscle cells of vascular tissues, suggesting that ER signaling involves in function of smooth muscle cells. Moreover, ERKO mice possess inherently vascular disease [51]. However, ERα is expressed in tissues involved in glucose and lipid metabolism such as the brain, adipose tissue, liver, and heart [52]. Imbalanced ERα/ERβ ratios may lead to the development of metabolic diseases [53]. αERKO mice are diabetic and insulin resistant. βERKO mice exhibited insulin responsibility and have not excessive fat in the body [54].
Estrogen is fundamental for regulating the growth and differentiation of axons and dendrites in the brain [55]. Functions performed by ERα and ERβ in the brain have been explored by studies of αERKO and βERKO mice. Interestingly, previous studies demonstrated differences in localization and expression levels of both ER isoforms. In addition, the results of these studies have shown that the function of ERα seems to be important for reproduction while that of ERβ may affect cognitive processes [5,6,56]. However, the level of ER expression in the brain rapidly changes in response to injury [57]. ERα primarily contributes in masculinization while ERβ contributes to the defeminization of sexual behavior [58]. ERKO females display aggressive behavior and depression. Additionally, estrogen signaling contributes to brain integrity and exerts different effects on behavior [59].
Estrogen regulates the growth, development, and physiology of the human reproductive system. This hormone also influences neuroendocrine, skeletal, adipose, and cardiovascular systems. Estrogen is an important sex hormone produced primarily by the ovaries in females and testes in males. The biological actions of estrogen are mediated by binding to the ERs in target organs. ERα and ERβ are transcriptional factors that regulate the expression of specific genes in different tissues on a ligand dependent manner. Analysis of ERα and ERβ functions using knockout mouse models has demonstrated the roles of estrogen signaling in different physiological processes. αERKO and βERKO mice are also valuable for examining the effect of ER signaling in specific target organs. Although transgenic mice do not always produce consistent results, these models are useful for evaluating the functions of genes under specific pathological conditions.
Figures and Tables
Figure 1
Mechanisms of estrogen receptor signaling pathway. Estrogen (E2) is able to bind to estrogen receptors (ERs). The ERs then dimerize and translocate into the nucleus. These complexes bind to estrogen response elements (EREs). ERs contain five domains with distinct functions. The A/B domain contains transcriptional activation function 1 (AF1). The C domain has the DNA binding domain and the D domain is a hinge region. The E/F domain encodes the AF2 region.
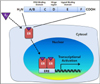
Acknowledgments
This work was supported by a National Research Foundation of Korea (NRF) grant (no. 2011-0015385) funded by the Ministry of Education, Science and Technology (MEST) of the South Korean government. In addition, this work was also supported by the Priority Research Centers Program (2011-0031403) through the NRF funded by the MEST.
References
1. Osz J, Brelivet Y, Peluso-Iltis C, Cura V, Eiler S, Ruff M, Bourguet W, Rochel N, Moras D. Structural basis for a molecular allosteric control mechanism of cofactor binding to nuclear receptors. Proc Natl Acad Sci U S A. 2012. 109(10):E588–E594.
2. Choi KC, Jeung EB. The biomarker and endocrine disruptors in mammals. J Reprod Dev. 2003. 49(5):337–345.
3. Weigel NL, Moore NL. Cyclins, cyclin dependent kinases, and regulation of steroid receptor action. Mol Cell Endocrinol. 2007. 265-266:157–161.
4. Lee HR, Hwang KA, Park MA, Yi BR, Jeung EB, Choi KC. Treatment with bisphenol A and methoxychlor results in the growth of human breast cancer cells and alteration of the expression of cell cycle-related genes, cyclin D1 and p21, via an estrogen receptor-dependent signaling pathway. Int J Mol Med. 2012. 29(5):883–890.
5. Swedenborg E, Power KA, Cai W, Pongratz I, Ruegg J. Regulation of estrogen receptor beta activity and implications in health and disease. Cell Mol Life Sci. 2009. 66(24):3873–3894.
6. Hughes ZA, Liu F, Marquis K, Muniz L, Pangalos MN, Ring RH, Whiteside GT, Brandon NJ. Estrogen receptor neurobiology and its potential for translation into broad spectrum therapeutics for CNS disorders. Curr Mol Pharmacol. 2009. 2(3):215–236.
7. Xiao J, Wang NL, Sun B, Cai GP. Estrogen receptor mediates the effects of pseudoprotodiocsin on adipogenesis in 3T3-L1 cells. Am J Physiol Cell Physiol. 2010. 299(1):C128–C138.
8. Welboren WJ, Sweep FC, Span PN, Stunnenberg HG. Genomic actions of estrogen receptor alpha: what are the targets and how are they regulated? Endocr Relat Cancer. 2009. 16(4):1073–1089.
9. Kong EH, Pike AC, Hubbard RE. Structure and mechanism of the oestrogen receptor. Biochem Soc Trans. 2003. 31(Pt 1):56–59.
10. Skafar DF, Zhao C. The multifunctional estrogen receptor-alpha F domain. Endocrine. 2008. 33(1):1–8.
11. Geserick C, Meyer HA, Haendler B. The role of DNA response elements as allosteric modulators of steroid receptor function. Mol Cell Endocrinol. 2005. 236(1-2):1–7.
12. Edwards DP. The role of coactivators and corepressors in the biology and mechanism of action of steroid hormone receptors. J Mammary Gland Biol Neoplasia. 2000. 5(3):307–324.
13. Sanchez ER. Chaperoning steroidal physiology: Lessons from mouse genetic models of Hsp90 and its cochaperones. Biochim Biophys Acta. 2012. 1823(3):722–729.
14. Beliakoff J, Whitesell L. Hsp90: an emerging target for breast cancer therapy. Anticancer Drugs. 2004. 15(7):651–662.
15. McDevitt MA, Glidewell-Kenney C, Jimenez MA, Ahearn PC, Weiss J, Jameson JL, Levine JE. New insights into the classical and non-classical actions of estrogen: evidence from estrogen receptor knock-out and knock-in mice. Mol Cell Endocrinol. 2008. 290(1-2):24–30.
16. Park MA, Hwang KA, Choi KC. Diverse animal models to examine potential role(s) and mechanism of endocrine disrupting chemicals on the tumor progression and prevention: Do they have tumorigenic or anti-tumorigenic property? Lab Anim Res. 2011. 27(4):265–273.
17. Zhao C, Dahlman-Wright K, Gustafsson JA. Estrogen signaling via estrogen receptor {beta}. J Biol Chem. 2010. 285(51):39575–39579.
18. Zhao C, Dahlman-Wright K, Gustafsson JA. Estrogen receptor beta: an overview and update. Nucl Recept Signal. 2008. 6:e003.
19. Lane PH. Estrogen receptors in the kidney: lessons from genetically altered mice. Gend Med. 2008. 5:Suppl A. S11–S18.
20. Weiser MJ, Foradori CD, Handa RJ. Estrogen receptor beta in the brain: from form to function. Brain Res Rev. 2008. 57(2):309–320.
21. Hwang KA, Park SH, Yi BR, Choi KC. Gene alterations of ovarian cancer cells expressing estrogen receptors by estrogen and bisphenol a using microarray analysis. Lab Anim Res. 2011. 27(2):99–107.
22. Chen M, Wolfe A, Wang X, Chang C, Yeh S, Radovick S. Generation and characterization of a complete null estrogen receptor alpha mouse using Cre/LoxP technology. Mol Cell Biochem. 2009. 321(1-2):145–153.
23. Jayachandran M, Preston CC, Hunter LW, Jahangir A, Owen WG, Korach KS, Miller VM. Loss of estrogen receptor beta decreases mitochondrial energetic potential and increases thrombogenicity of platelets in aged female mice. Age (Dordr). 2010. 32(1):109–121.
24. Singh SP, Wolfe A, Ng Y, DiVall SA, Buggs C, Levine JE, Wondisford FE, Radovick S. Impaired estrogen feedback and infertility in female mice with pituitary-specific deletion of estrogen receptor alpha (ESR1). Biol Reprod. 2009. 81(3):488–496.
25. Santollo J, Eckel LA. Effect of a putative ERalpha antagonist, MPP, on food intake in cycling and ovariectomized rats. Physiol Behav. 2009. 97(2):193–198.
26. Shao R. Understanding the mechanisms of human tubal ectopic pregnancies: new evidence from knockout mouse models. Hum Reprod. 2010. 25(3):584–587.
27. Bockamp E, Sprengel R, Eshkind L, Lehmann T, Braun JM, Emmrich F, Hengstler JG. Conditional transgenic mouse models: from the basics to genome-wide sets of knockouts and current studies of tissue regeneration. Regen Med. 2008. 3(2):217–235.
28. Sun J, Langer WJ, Devish K, Lane PH. Compensatory kidney growth in estrogen receptor-alpha null mice. Am J Physiol Renal Physiol. 2006. 290(2):F319–F323.
29. Chen M, Hsu I, Wolfe A, Radovick S, Huang K, Yu S, Chang C, Messing EM, Yeh S. Defects of prostate development and reproductive system in the estrogen receptor-alpha null male mice. Endocrinology. 2009. 150(1):251–259.
30. Choleris E, Clipperton AE, Phan A, Kavaliers M. Estrogen receptor beta agonists in neurobehavioral investigations. Curr Opin Investig Drugs. 2008. 9(7):760–773.
31. Wintermantel TM, Elzer J, Herbison AE, Fritzemeier KH, Schutz G. Genetic dissection of estrogen receptor signaling in vivo. Ernst Schering Found Symp Proc. 2006. (1):25–44.
32. Lee GS, Kim HJ, Jung YW, Choi KC, Jeung EB. Estrogen receptor alpha pathway is involved in the regulation of Calbindin-D9k in the uterus of immature rats. Toxicol Sci. 2005. 84(2):270–277.
33. An BS, Choi KC, Hong EJ, Jung YW, Manabe N, Jeung EB. Differential transcriptional and translational regulations of calbindin-D9k by steroid hormones and their receptors in the uterus of immature mice. J Reprod Dev. 2004. 50(4):445–453.
34. Lee S, Kang DW, Hudgins-Spivey S, Krust A, Lee EY, Koo Y, Cheon Y, Gye MC, Chambon P, Ko C. Theca-specific estrogen receptor-alpha knockout mice lose fertility prematurely. Endocrinology. 2009. 150(8):3855–3862.
35. Glidewell-Kenney C, Hurley LA, Pfaff L, Weiss J, Levine JE, Jameson JL. Nonclassical estrogen receptor alpha signaling mediates negative feedback in the female mouse reproductive axis. Proc Natl Acad Sci U S A. 2007. 104(19):8173–8177.
36. Drummond AE, Fuller PJ. The importance of ERbeta signalling in the ovary. J Endocrinol. 2010. 205(1):15–23.
37. Silberstein GB, Van Horn K, Hrabeta-Robinson E, Compton J. Estrogen-triggered delays in mammary gland gene expression during the estrous cycle: evidence for a novel timing system. J Endocrinol. 2006. 190(2):225–239.
38. Hewitt SC, Korach KS. Oestrogen receptor knockout mice: roles for oestrogen receptors alpha and beta in reproductive tissues. Reproduction. 2003. 125(2):143–149.
39. McPherson SJ, Ellem SJ, Risbridger GP. Estrogen-regulated development and differentiation of the prostate. Differentiation. 2008. 76(6):660–670.
40. Raskin K, de Gendt K, Duittoz A, Liere P, Verhoeven G, Tronche F, Mhaouty-Kodja S. Conditional inactivation of androgen receptor gene in the nervous system: effects on male behavioral and neuroendocrine responses. J Neurosci. 2009. 29(14):4461–4470.
41. Lee KH, Park JH, Bunick D, Lubahn DB, Bahr JM. Morphological comparison of the testis and efferent ductules between wild-type and estrogen receptor alpha knockout mice during postnatal development. J Anat. 2009. 214(6):916–925.
42. Imai Y, Kondoh S, Kouzmenko A, Kato S. Regulation of bone metabolism by nuclear receptors. Mol Cell Endocrinol. 2009. 310(1-2):3–10.
43. Li BY, Tong J, Zhang ZL. [Exogenous estrogen improved calcium homeostasis and skeletal mineralization in vitamin D receptor gene knockout female mice.]. Sheng Li Xue Bao. 2006. 58(6):573–576.
44. Venken K, Callewaert F, Boonen S, Vanderschueren D. Sex hormones, their receptors and bone health. Osteoporos Int. 2008. 19(11):1517–1525.
45. Auld KL, Berasi SP, Liu Y, Cain M, Zhang Y, Huard C, Fukayama S, Zhang J, Choe S, Zhong W, Bhat BM, Bhat RA, Brown EL, Martinez RV. Estrogen-related receptor α regulates osteoblast differentiation via Wnt/β-catenin signaling. J Mol Endocrinol. 2012. 48(2):177–191.
46. Vico L, Vanacker JM. Sex hormones and their receptors in bone homeostasis: insights from genetically modified mouse models. Osteoporos Int. 2010. 21(3):365–372.
47. Chilibeck PD, Cornish SM. Effect of estrogenic compounds (estrogen or phytoestrogens) combined with exercise on bone and muscle mass in older individuals. Appl Physiol Nutr Metab. 2008. 33(1):200–212.
48. Syed FA, Fraser DG, Spelsberg TC, Rosen CJ, Krust A, Chambon P, Jameson JL, Khosla S. Effects of loss of classical estrogen response element signaling on bone in male mice. Endocrinology. 2007. 148(4):1902–1910.
49. Knowlton AA, Lee AR. Estrogen and the cardiovascular system. Pharmacol Ther. 2012. 135(1):54–70.
50. Kim KH, Moriarty K, Bender JR. Vascular cell signaling by membrane estrogen receptors. Steroids. 2008. 73(9-10):864–869.
51. Luksha L, Kublickiene K. The role of estrogen receptor subtypes for vascular maintenance. Gynecol Endocrinol. 2009. 25(2):82–95.
52. Barros RP, Gustafsson JA. Estrogen receptors and the metabolic network. Cell Metab. 2011. 14(3):289–299.
53. Riant E, Waget A, Cogo H, Arnal JF, Burcelin R, Gourdy P. Estrogens protect against high-fat diet-induced insulin resistance and glucose intolerance in mice. Endocrinology. 2009. 150(5):2109–2117.
54. Faulds MH, Zhao C, Dahlman-Wright K, Gustafsson JA. The diversity of sex steroid action: regulation of metabolism by estrogen signaling. J Endocrinol. 2012. 212(1):3–12.
55. Kalita K, Szymczak S. [Estrogen receptors in the brain]. Neurol Neurochir Pol. 2003. 37:Suppl 3. 63–78.
56. Hill RA, Boon WC. Estrogens, brain, and behavior: lessons from knockout mouse models. Semin Reprod Med. 2009. 27(3):218–228.
57. Walf AA, Koonce C, Manley K, Frye CA. Proestrous compared to diestrous wildtype, but not estrogen receptor beta knockout, mice have better performance in the spontaneous alternation and object recognition tasks and reduced anxiety-like behavior in the elevated plus and mirror maze. Behav Brain Res. 2009. 196(2):254–260.
58. Kudwa AE, Michopoulos V, Gatewood JD, Rissman EF. Roles of estrogen receptors alpha and beta in differentiation of mouse sexual behavior. Neuroscience. 2006. 138(3):921–928.
59. Crews D, Fuller T, Mirasol EG, Pfaff DW, Ogawa S. Postnatal environment affects behavior of adult transgenic mice. Exp Biol Med (Maywood). 2004. 229(9):935–939.