Abstract
The effects of polarized-light therapy (PLT) on high-cholesterol diet (HCD)-induced hypercholesterolemia and atherosclerosis were investigated in comparison with that of lovastatin in rabbits. Hypercholesterolemia was induced by feeding male New Zealand white rabbits with 1% cholesterol in diet for 2 weeks and maintained with 0.5% cholesterol for 6 weeks, followed by normal diet for 2 weeks for recovery. Lovastatin (0.002% in diet) or daily 5-min or 20-min PLT on the outside surface of ears was started 2 weeks after induction of hypercholesterolemia. Hypercholesterolemic rabbits exhibited great increases in serum cholesterol and low-density lipoproteins (LDL) levels, and finally severe atheromatous plaques formation covering 57.5% of the arterial walls. Lovastatin markedly reduced both the cholesterol and LDL, but the reducing effect (47.5%) on atheroma formation was relatively low. By comparison, 5-min PLT preferentially decreased LDL, rather than cholesterol, and thereby potentially reduced the atheroma area to 42.2%. Notably, 20-min PLT was superior to lovastatin in reducing both the cholesterol and LDL levels as well as the atheromatous plaque formation (26.4%). In contrast to the increases in blood alanine transaminase and aspartate transaminase following lovastatin treatment, PLT did not cause hepatotoxicity. In addition, PLT decreased platelets and hematocrit level. The results indicate that PLT attenuates atherosclerosis not only by lowering blood cholesterol and LDL levels, but also by improving blood flow without adverse effects. Therefore, it is suggested that PLT could be a safe alternative therapy for the improvement of hypercholesterolemia and atherosclerosis.
In Korea, the patterns of disease have changed dramatically in parallel with rapid economic development, accompanied by Westernization of lifestyles [1]. Recently, according to the change in the pattern of food consumption, the incidence rate of metabolic syndromes such as obesity, stroke, atherosclerosis, hypertension, diabetes and cancer are considerably increasing [2,3]. Especially, hyperlipidemia is becoming the main cause of health problems [4].
The major risk factors of cardiovascular diseases are increasing levels of total cholesterol, triglycerides (TG) and low-density lipoproteins (LDL) accompanying decrease in high-density lipoproteins (HDL) [5,6]. It has been reported that increased consumption of cholesterol and triglycerides is closely associated with hypercholesterolemia, leading to atherosclerosis [7,8].
Hypercholesterolemia is an important risk factor of atherosclerosis [9,10]. LDL, major transporter of blood cholesterol, is oxidized in the endothelium of arterial walls and induces infiltration of circling monocytes following endothelial cell injuries. Oxidized LDL (OxLDL) stimulates vascular endothelial cells to produce monocyte-colony stimulating factor (M-CSF) and induces differentiation of infiltrated monocytes to macrophages [11]. Then, LDL binds to several scavenger receptors expressed on macrophages instead of LDL receptors, one of the superficial receptor of macrophages, disrupting feedback controlling mechanism of intracellular cholesterol level. Macrophages that contain highly enriched cholesterol become foam cells and form fatty streak namely atheromatous plaques, initial lesion of atherosclerosis [12].
Several drugs that are clinically used to treat for hyperlipidemia are classified as 3-hydroxy-3-methylglutanyl-coenzyme A (HMG-CoA) reductase inhibitor (statins), bile acid sequestrant (resin), nicotinic acid, fibrate and probucol [13,14]. Among them, statins inhibit HMG-CoA reductase, rate-limiting step enzyme in cholesterol biosynthesis, thereby reduce intracellular cholesterol level. Statins also increase the activity of LDL receptors on cell membrane, accelerating the metabolism of very low-density lipoproteins (VLDL) remnant and LDL [15]. Even though drugs with such mechanism have an advantage to lower blood cholesterol level remarkably, but they exert serious adverse effects like hepatomegaly and renal hypertrophy [16,17]. Thus, researches on the development of natural resources with a novel mechanism without side effects are required.
It is well known that light with specific wavelength exerts influence on organisms, which has been reconsidered in terms of modern medicine [18]. Although sunlight had been believed to have beneficial effects on health in Egypt in B.C., it has been for the treatment of diseases in 1990s [19]. Especially, in 1960s, some studies showed that sunlight has a significant influence on feelings of daily life as well as physical and mental health [20]. Recently, it has been demonstrated that ultraviolet rays, far-infrared radiation, laser and visible lights including sunlight were applicable for disinfection and health improvement. For example, in late 1980s, Low-Level-Light Therapy (LLLT) using narrow-band (632.8 nm) light has been developed for the relief of pain and inflammation as well as for immune-boosting and wound-healing effects [21-26]. Moreover, various light therapies are being studied from a medical perspective [27].
Notably, several studies showed that Polarized-Light Therapy (PLT), using strained broad-band (400-760 nm) visible light through a polarizing lens, exhibited greater pain-relieving effects than LLLT with narrow-band wavelength [21-23,25,26]. Recently, it was confirmed that PLT improved blood circulation and blood pressure of hypertensive patients by reducing fibrinogen, platelets and blood viscosity, preventing aggregation of platelets, and delaying blood coagulation time (unpublished results). PLT displayed additional beneficial effects: i.e., decreasing blood cholesterol and triglycerides, improving immune function, relieving pain and inflammation, and facilitating wound healing in human studies (unpublished results).
Therefore, such results led us to investigate the improving effects of PLT on high-cholesterol diet (HCD)-induced hypercholesterolemia and atherosclerosis in comparison with lovastatin, a clinically available cholesterol-controlling statin, in rabbits.
The PLT equipment is consisted of a 60 W tungsten lamp and a lens coated with polarized film in a stainless tube with 6-cm internal diameter. The polarized film (Nucell Biomedics, Cheongju, Korea) was designed to block ultraviolet and infrared lights, permitting penetration of only 400-760 nm light, which was confirmed by prism analysis at Photonics Polymer Laboratory (Gwangju, Korea). The distance between light source and polarized-light lens was set at 10 cm to adjust the light intensity and energy density exposed to the skin to be 50 mW/cm2 and 60 J/cm2, respectively.
An anti-cholesterol reference drug Meverstin® containing lovastatin as an active ingredient was purchased from Choongwae Pharma Co. (Seoul, Korea). Cholesterol used to induce hypercholesterolemia and atherosclerosis was procured from Sigma-Aldrich (C75209; St. Louis, MO, USA).
Six-month-old male New Zealand white (NZW) rabbits (n=8/group) weighing 1.7-2.0 kg were from Daehan-Biolink (Eumseong, Korea), and subjected to the experiment after 1-week acclimation to the laboratory environment. The animals were housed in each cage with free access to feed and water under constant environmental conditions (22±2℃; 40-70% relative humidity; 12-hour light-dark cycle; 150-300 lux brightness). Animals were checked for abnormal sign every day and weighed once a week. All the animal experiments were conducted according to the Standard Operation Procedures, and approved by the Institutional Animal Care and Use Committee of Chungbuk National University, Korea.
Acute dietary hypercholesterolemia was induced by feeding animals with a powdered high-cholesterol diet (HCD) containing 1% cholesterol for 2 weeks, followed by 0.5% cholesterol for additional 6 weeks during maintenance period and normal diet without cholesterol for recovery period of 2 weeks.
After 2-week induction of hypercholesterolemia, the animals were grouped (n=8/group) according to their blood cholesterol levels to adjust similar mean values. To assess therapeutic efficacy of PLT against hypercholesterolemia and atherosclerosis, the rabbits were exposed to PLT light for 5 or 20 min on their outside surface of ear with 10 cm distance from lens every day (10:00-12:00) for 8 weeks. Separately, 0.002% lovastatin was supplemented in diet for the 8-week treatment period.
After 16-hour fasting at the ends of 2-week cholesterolemia induction and 10-week total experimental period (2-week induction, 6-week maintenance and 2-week recovery), blood sample was collected from auricular artery, and hematocrit, red blood cells (RBC), hemoglobin, mean corpuscular volume (MCV), mean corpuscular hemoglobin (MCH), mean corpuscular hemoglobin concentration (MCHC), white blood cells (WBC), differential counts (neutrophils, eosinophils, basophils, lymphocytes and monocytes) and platelets were measured by a hematology analyzer (Hemavet 850; CDC Technologies Inc., Dayton, USA).
Lipid profiles including total cholesterol, LDL, HDL, triglycerides, fibrin-degradation products (FDP) and D-dimer were measured in serum obtained from centrifuged (3,000 rpm×20 min) blood. Parameters of hepatic function such as alanine transaminase (ALT), aspartate transaminase (AST), alkaline phosphatase (ALP) and total bilirubin were analyzed by blood chemistry analyzer (Hitachi-747; Hitachi Korea, Seoul, Korea).
The aortic arch (14 cm) was dissected from the aortic valve of the heart and washed with saline. The sample was dissected in 10 cm long from the orifice of carotid artery, and opened longitudinally. After removing fats and tissues adhering to the adventitia, the aorta was dehydrated with 100% propylene glycol for 10 min, and stained with 0.7% Sudan IV (in propylene glycol) for 10 min. Then, it was hydrated with 85% propylene glycol for 5 min, washed with distilled water, and photographed and analyzed with Digital Image Analyzer (Image Inside; Focus, Seoul, Korea) for red atheromatous plaques. The extent of lipid accumulation (atherosclerosis index; AI, %) was calculated as the percent Sudan-positive area to the total area of the aortic wall.
In comparison with normal animals, the body weight gain of HCD-fed animals slightly increased (Figure 1). Daily PLT for 5 or 20 min did not affected the body weight gain of HCD-fed rabbits, while treatment with lovastatin (0.002% in diet) slightly reduced the body weight gain, decreasing the final body weight below that of normal animals. Lovastatin also caused rough skin and hair of the animals, suggestive of a possibility of adverse effects.
Two-week feeding of 1% HCD caused hematological changes: increases in WBC (40-60%) and lymphocytes (38-92%) and decreases in basophils (44-78%) and platelets (22-30%), while hematocrit, RBC and RBC indices were not changed (Table 1).
Meanwhile, after 8-week feeding of HCD followed by 2-week recovery period, the number of WBC was maintained high, while basophils remained low. Notably, platelets increased after cease of HCD feeding. However, 5-min PLT attenuated the platelets increase, and 20-min PLT substantially inhibited the increases in hematocrit, WBC and platelets. Whereas, lovastatin did not exhibit any effects, except increasing lymphocytes and monocytes. Hence it is considered that PLT improves blood flow more effectively by alleviating hemoconcentration, inflammatory WBC and thrombosis-inducing platelets.
After 2-week feeding of 1% cholesterol, total cholesterol dramatically elevated to 1,720.0-1,736.7 mg/dL from 51.8 mg/dL of normal level (Table 2). Accordingly, LDL also increased to 651.1-684.2 mg/dL from 24.5 mg/dL in normal animals, although there was a slightly increase in HDL levels to 29.0-32.4 mg/dL from 18.0 mg/dL. These changes of lipoproteins induced increase in FDP (3.8-7.1 µg/dL), a metabolite of fibrin, although another fibrin metabolite D-dimer and triglycerides were not remarkably affected. ALP, an indicator of cholestasis slightly increased, without alterations in ALT and AST, parameters of hepatocytic damage.
In 10 weeks after 8-week feeding of HCD and 2-week recovery, blood cholesterol, LDL and FDP were recovered to 1,415.0mg/dL, 475.5mg/dL and 1.6 µg/dL, respectively. Lovastatin significantly reduced both the cholesterol and LDL levels to 855.0 and 280.1 mg/dL, respectively. In comparison, 5-min PLT lowered LDL level more preferably than cholesterol. Interestingly, 20-min PLT markedly decreased both the cholesterol and LDL levels comparable with lovastatin. More importantly, lovastatin significantly increased the blood ALT and AST values, indicative of hepatotoxicity, in contrast to the normal levels in PLT animals.
During 6-week feeding of 0.5% cholesterol diet, the blood cholesterol level maintained high (Figure 2), although LDL recovered gradually (Figure 3). Lovastatin significantly decreased the cholesterol level from 2-week treatment to reach 2-third level after 6-week feeding. Five-min PLT also reduced the blood cholesterol level after 4-week treatment, although the statistical significance was not achieved. Notably, 20-min PLT markedly lowered the cholesterol level even in higher degree than lovastatin. Similar effects of lavastatin and 20-min PLT were obtained on blood LDL level. Importantly, 5-min PLT also exerted a remarkable efficacy in LDL reduction.
Feeding HCD with 1% cholesterol for 2 weeks, 0.5% cholesterol for 6 weeks and recovery for 2 weeks caused extensive atheromatous plaques covering 57.5% of the aortic arch and abdominal artery (Figure 4 and Table 3). The degree of atheromatous plaques formation was attenuated by treatment with lovastatin to 47.5%. However, the anti-atherosclerotic activity of PLT was superior to lovastatin: 5-min and 20-min PLT decreased the plaque area to 42.2% and 26.4%, respectively.
To investigate the PLT's effects on blood lipid profiles and change in vessel walls, HCD was fed to rabbits to induce hypercholesterolemia and ensuing atherosclerosis. Rabbits has been used to study atherosclerosis because their enzyme activity of cholesterol ester transfer proteins (CETP), a causative factor of arteriosclerosis in human, is three times higher than human, so that hypercholesterolemia can be induced in a short period and lesions such as foam cells and fatty streak be easily induced [28].
Lovastatin is a metabolic derivate of Aspergillus terreus and preferentially prescribed for hypercholesterolemia and cardiovascular diseases. Lovastatin, as HMG-CoA reductase inhibitor, suppress cholesterol synthesis and improves bile duct secretion [29-31]. Accordingly, lovastatin remarkably reduced cholesterol and LDL after 2-week feeding in the present study. Compare to lovastatin, 5-min PLT did not show any effects on cholesterol and LDL within 2 weeks. However, 5-min PLT remarkably reduced the LDL level, a key factor of atheroma formation, from 4-week therapy. Moreover, 20-min PLT markedly lowered both the cholesterol and LDL levels with higher efficacy than lovastatin.
It is of interest to note that 5-min PLT was more effective in the suppression of atheromatous lesion formation than lovastatin, in spite of the lower activity on cholesterol and LDL. Accordingly, 20-min PLT was much superior to lovastatin in the prevention of atherosclerosis, in which blood lipid levels were lowered to similar extents to lovastatin. Therefore, it is estimated that, in addition to the lipid-lowering activity, PLT might inhibit oxidation and accumulation in the aortic wall of LDL, although the exact mechanisms remain to be clarified. In addition, PLT decreased platelets and hematocrit level related to the improvement of blood flow. PLT also decreased WBC, while lovastatin increased lymphocytes and monocytes. Hence it is considered that PLT improves blood flow more effectively by alleviating hemoconcentration, inflammatory WBC and thrombosis-inducing platelets.
In blood biochemistry, ALT and AST significantly increased following lovastatin treatment. The hepatotoxicity is a critical factor limiting long-term use of high dose lovastatin. It was reported in previous studies that statins caused hepatic injuries with increases in ALT and AST as well as hepatobiliary dysfunction, myocardial degeneration, fetal malformations with skeletal defects presumably by disrupting lipid-metabolic processes [32,33]. PLT did not induce ALT and AST increases, and 20-min PLT rather lowered the HCD-induced increase in ALP, a parameter of cholestasis.
In the present study, it was demonstrated that PLT substantially attenuated atherosclerosis not only by lowering blood cholesterol and LDL levels, but also by improving blood flow without adverse effects such as hepatotoxicity. Therefore, it is suggested that PLT could be an alternative therapy for the improvement of hypercholesterolemia and prevention of atherosclerosis.
Figures and Tables
Figure 1
Change in body weight of high-cholesterol diet (HCD)-fed rabbits (n=8) treated with lovastatin or polarized-light therapy (PLT). Treatments were started 2 weeks following induction of hyperchoesterolemia. ○, normal; •, HCD; ▾, HCD+0.002% lovastatin; ▪, HCD+5 min PLT; ♦, HCD+20 min PLT.
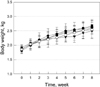
Figure 2
Time-course of blood cholesterol level in highcholesterol diet (HCD)-fed rabbits (n=8) treated with lovastatin or polarized-light therapy (PLT). Treatments were started 2 weeks following induction of hypercholesterolemia. ○, normal; •, HCD; ▾, HCD+0.002% lovastatin; ▪, HCD+5 min PLT; ♦, HCD+20 min PLT. *Significantly different from HCD alone, P<0.05.
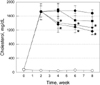
Figure 3
Time-course of blood low-density lipoproteins (LDL) level of high-cholesterol diet (HCD)-fed rabbits (n=8) treated with lovastatin or polarized-light therapy (PLT). Treatments were started 2 weeks following induction of hypercholesterolemia. ○, normal; •, HCD; ▾, HCD+0.002% lovastatin; ▪, HCD+5 min PLT; ♦, HCD+20 min PLT. *Significantly different from HCD alone, P<0.05.
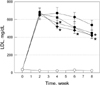
Figure 4
Representative findings of the aorta of high-cholesterol diet (HCD)-fed rabbits (n=8) treated with lovastatin (0.002%) or 5 min or 20 min polarized-light therapy (PLT). Note extensive atheromatous plaques induced by HCD and marked attenuation by 20 min PLT, in comparison with intact features in rabbits fed normal diet.
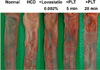
Table 1
Hematology of rabbits (n=8) fed a high-cholesterol (HCD) for 2 weeks followed by lovastatin treatment or polarized-light therapy (PLT) for 8 weeks (6-week maintenance and 2-week recovery periods)
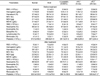
Acknowledgments
This work was supported by the research grant of the Chungbuk National University in 2011.
References
1. Moon SJ. Nutritional problems in Korea. Korean J Nutr. 1996. 29(4):371–380.
2. Huh KB. The present status of nutrition-related diseases and its countermeasures. Korean J Nutr. 1990. 23(3):197–207.
3. Lee HK. Pattern of disease incidence and nutrition in Korea. Korean J Nutr. 1996. 29(4):381–383.
4. Lee YC. Hypercholesterolemia in Korea and nutritional factors. Korean J Lipidol. 1991. 1(1):111–122.
5. Spady DK, Woollett LA, Dietschy JM. Regulation of plasma LDL-cholesterol levels by dietary cholesterol and fatty acids. Annu Rev Nutr. 1993. 13:355–381.
6. Natio HK. Atherogenesis: current topics on etiology and risk factors. Clin Chem. 1995. 41:132–133.
7. Sanders TA, Oakley FR, Miller GJ, Mitropoulos KA, Crook D, Oliver MF. Influence of n-6 versus n-3 polyunsaturated fatty acids in diets low in saturated fatty acids on plasma lipoproteins and hemostatic factors. Arterioscler Thromb Vasc Biol. 1997. 17(12):3449–3460.
8. Judd JT, Baer DJ, Clevidence BA, Muesing RA, Chen SC, Weststrate JA, Meijer GW, Wittes J, Lichtenstein AH, Vilella-Bach M, Schaefer EJ. Effects of margarine compared with those of butter on blood lipid profiles related to cardiovascular disease risk factors in normolipemic adults fed controlled diets. Am J Clin Nutr. 1998. 68(4):768–777.
9. Keys A. Coronary heart disease in seven countries. Circulation. 1970. 41:1–11.
10. Kannel WB, Castelli WP, Gordon T, McNamara PM. Serum cholesterol, lipoproteins, and the risk of coronary heart disease. The Framingham study. Ann Intern Med. 1971. 74(1):1–12.
11. Bae HY. The role of oxidized low density lipoprotein in atherogenesis. J Korean Diabetes Assoc. 1999. 23:7–11.
12. Glass CK, Witztum JL. Atherosclerosis. the road ahead. Cell. 2001. 104(4):503–516.
13. Shin SH. The choice of medicine. Diagnosis and Treatment in Hyperlipidemia. 2000. Haneuyhak, Seoul: Association of Hyperlipidemic Theraphy;315–331.
14. Blum A, Simsolo C, Hasin Y. 3-Hydroxy-3-methylglutaryl coenzyme a (HMG-CoA) reductase inhibitors (statins), atherosclerosis and coronary syndromes. Atherosclerosis. 2004. 175(1):1–5.
15. Larsen ML, Illingworth DR. Drug treatment of dyslipoproteinemia. Med Clin North Am. 1994. 78(1):225–245.
16. Cho JH, Lee NJ, Chai HY, Kim TM, Park JH, Kang JK, Kim YB, Hwang SY. Effect of Hwalgidan SJ-101 on atherosclerosis in hypercholesterolemic rabbits. Lab Anim Res. 2005. 21:149–158.
17. Hong SH, Chai HY, Kim TM, Lee NJ, Kim DK, Cho JH, Park JH, Kim YB, Kang JK, Hwang SY. Therapeutic effects of mulberry root-bark (Mori radicis Cortex) ethanol-extracts on atherosclerosis in hypercholesterolemic rabbits. Lab Anim Res. 2005. 21:273–279.
18. Hsiang YN, Todd ME, Bower RD. Determining light dose for photodynamic therapy of atherosclerotic lesions in the Yucatan miniswine. J Endovasc Surg. 1995. 2(4):365–371.
19. Hayashi J, Saito T, Aizawa K. Change in chemical composition of lipids accumulated in atheromas of rabbits following photodynamic therapy. Lasers Surg Med. 1997. 21(3):287–293.
20. Amemiya T, Nakajima H, Katoh T, Rakue H, Miyagi M, Ibukiyama C. Photodynamic therapy of atherosclerosis using YAG-OPO laser and Porfimer sodium, and comparison with using argon-dye laser. Jpn Circ J. 1999. 63(4):288–295.
21. Lymans'kyi IuP, Tamarova ZA, Hutliar SO, Bidkov IeH. The examination of the analgetic action of polarized light. Fiziol Zh. 2000. 46(6):105–111.
22. Lymans'kyi IuP, Tamarova ZA, Hutliar SO. Suppression of visceral pain by action of the low intensity polarized light on acupuncture antinociceptive points. Fiziol Zh. 2003. 49(5):43–51.
23. Samosiuk IZ, Kulikovich IuN, Tamarova ZA, Samosiuk NI, Kazhanova AK. Pain relief by low-intensity frequency-modulated millimeter waves acting on the acupuncture points. Vopr Kurortol Fizioter Lech Fiz Kult. 2000. 4:7–11.
24. Hafkamp AM, Havinga R, Sinaasappel M, Verkade HJ. Effective oral treatment of unconjugated hyperbilirubinemia in Gunn rats. Hepatology. 2005. 41(3):526–534.
25. Tamarova ZA, Lymans'kyi IuP, Hutliar SO. Comparative testing of analgesia induced by polarized light and analgetics. Fiziol Zh. 2005. 51(2):57–64.
26. Limansky YP, Tamarova ZA, Gulyar SA. Suppression of pain by exposure of acupuncture points to polarized light. Pain Res Manag. 2006. 11(1):49–57.
27. Rastad C, Ulfberg J, Lindberg P. Improvement in Fatigue, Sleepiness, and Health-Related Quality of Life with Bright Light Treatment in Persons with Seasonal Affective Disorder and Subsyndromal SAD. Depress Res Treat. 2011. 2011:543906.
28. Kobayashi H, Matsushita M, Oda K, Nishikimi N, Sakurai T, Komori K. Effects of atherosclerotic plaque on the enlargement of an experimental model of abdominal aortic aneurysm in rabbits. Eur J Vasc Endovasc Surg. 2004. 28(1):71–78.
29. Anistchow N, Chalatow S. Ueber experimentelle cholesterinstetase und die bedeutung fur die enstehung einiger pathdogischer prozesse. Zebtrabl Allg Pathol. 1913. 24:1–9.
30. McClelland GA, Stubbs RJ, Fix JA, Pogany SA, Zentner GM. Enhancement of 3-hydroxy-3-methylglutaryl-coenzyme A (HMGCoA) reductase inhibitor efficacy through administration of a controlled-porosity osmotic pump dosage form. Pharm Res. 1991. 8(7):873–876.
31. Yim JE, Choue RW, Kim YS. Effects of dietary counceling and HMG Co A reductase inhibitor treatment on serum lipid levels in hyperlipidemic patients. Korean J Lipidol. 1998. 8(1):61–76.
32. MacDonald JS, Gerson RJ, Kornbrust DJ, Kloss MW, Prahalada S, Berry PH, Alberts AW, Bokelman DL. Preclinical evaluation of lovastatin. Am J Cardiol. 1988. 62(15):16J–27J.
33. de Denus S, Spinler SA, Miller K, Peterson AM. Statins and liver toxicity: a meta-analysis. Pharmacotherapy. 2004. 24(5):584–591.