Abstract
The anti-obesity activities of Rapha diet® preparation containing silkworm pupa peptide, Garcinia cambogia, white bean extract, mango extract, raspberry extract, cocoa extract, and green tea extract were investigated in mice with dietary obesity. Male C57BL/6 mice were fed a high-fat diet (HFD) containing 3% Rapha diet® preparation for 8 weeks, and blood and tissue parameters of obesity were analyzed. The HFD markedly enhanced body weight gain by increasing the weights of epididymal, perirenal, and mesenteric adipose tissues. The increased body weight gain induced by HFD was significantly reduced by feeding Rapha diet® preparation, in which decreases in the weight of abdominal adipose tissue and the size of abdominal adipocytes were confirmed by microscopic examination. Long-term feeding of HFD increased blood triglycerides and cholesterol levels, leading to hepatic lipid accumulation. However, Rapha diet® preparation not only reversed the blood lipid levels, but also attenuated hepatic steatosis. The results indicate that Rapha diet® preparation could improve HFD-induced obesity by reducing both lipid accumulation and the size of adipocytes.
Obesity is a major public health problem in developed countries as well as in developing countries [1]. The prevalence of obesity is steadily increasing and has more than doubled since 1980. According to World Health Organization (WHO) global estimates from 2008, over 500 million adults are obese (http://www.who.int/mediacentre/factsheets/fs311/en). Obesity is becoming leading risk for global deaths. At least 2.8 million adults die each year as a result of being overweight or obese. With severe obesity, over 30 kg/m2 body mass index (BMI), mortality is substantially elevated by 50-150% [2]. In addition, obesity is strongly associated with the development of serious diseases such as type 2 diabetes mellitus, cardiovascular diseases, and certain cancers [3-5].
Obesity has many causes including excessive intake of calories, genetic factors, endocrine dysfunction, metabolic disorders, and stresses [6]. Among these causes, excessive energy intake is the most common risk factor of obesity. The major dietary fat, triglycerides (TG), provide the major source of energy. TG is transported in the blood as lipoproteins and stored in adipose tissues [7]. The major classes of blood lipoproteins are chylomicrons, very low-density lipoproteins (VLDL), intermediate-density lipoproteins (IDL), low-density lipoprotein (LDL), and high-density lipoproteins (HDL). Chylomicrons are produced in intestinal cells from dietary lipid, and VLDL is produced in the liver, mainly from dietary carbohydrates. IDL and LDL are produced in blood capillary by digestion of the TG or VLDL. HDL transfers an activator of lipoprotein lipase (LPL) to chylomicrons and VLDL. HDL also carries cholesterol from peripheral tissues to the liver. TG of chylomicrons and VLDL are hydrolyzed by LPL to fatty acid and glycerol. The fatty acids may be oxidized by various tissues. In adipose cells, the fatty acids are converted TG and stored.
To treat obesity, constant control of diet and exercise are required [8]. However, it is hard for obese patients to maintain regular exercising steadily. Synthetic appetite suppressants or fat absorption blockers have been developed as anti-obesity drugs, yet the efficacy and long-term safety level of the drugs were not fully evaluated [9,10]. Therefore, safe and efficient anti-obesity substances are still needed.
Silkworm (Bombyx mori) has been ingested for a long time in Asian countries. It is well known that silk peptides (SP) and silkworm pupa peptides (SPP) have a blood glucose-regulating effect [11-14]. Recently, we demonstrated that SPP suppresses adipogenesis in preadipocytes and fat accumulation in rats [15]. Notably, in spite of body weight-lowering effect, silk amino acids (SAA) enhanced physical stamina [16,17].
Garcinia cambogia is an edible native Southeastern Asian fruit, used as spices [18]. Garcinia cambogia contains hydroxycitric acid (HCA), a competitive inhibitor of adenosine triphosphate (ATP) citrate lyase [18-20]. HCA reduces the acetyl-CoA pool, thus limiting the biosynthesis of fatty acid and cholesterol.
White bean (Phaseolus vulgaris) contains a high level of pancreatic α-amylase inhibitor [21,22]. It has been shown that the long-term administration of the α-amylase inhibitor from white bean extract reduced blood glucose levels and body weight gain in rats.
Mango is a rich source of various polyphenolic compounds [23]. Mango polyphenols, like other polyphenolic compounds, have a great antioxidative potential and they are expected to reduce degenerative diseases such as cancer, atherosclerosis, diabetes, and obesity [23,24].
Raspberry (Rubus idaeus) contains raspberry ketone, a major aromatic compound of red raspberry [25]. Raspberry ketone prevents and improves obesity and fatty liver by increasing norepinephrine-induced lipolysis in white adipocytes [25-27].
Cocoa can prevent high-fat diet-induced obesity by modulating lipid metabolism, especially by decreasing fatty acid synthesis and transport systems, and enhancement of a part of the thermogenesis mechanism in the liver and white adipose tissues [28].
Green tea contains a series of polyphenols known as catechins [29]. It is known that tea catechins modulate appetite and reduce food intake through the leptin receptor-independent pathway in rats [30]. Also, green tea components have been reported to possess various biological and pharmacological effects, such as lowering of plasma lipids and glucose levels [31].
In our repeated human trials (unpublished results), SPP enhanced physical stamina as shown in the animal studies [16,17], leading to increases in appetite and outdoor activities. Since the increased physical activity enhanced food intake and thereby attenuated body weight loss, we added extracts of Garcinia cambogia, white bean, mango, raspberry, cocoa, and green tea, possessing different mechanisms, to increase the anti-obesity effect and to control appetite. In the present study, we carried out an experiment to verify anti-obesity effects of Rapha diet® preparation containing the ingredients described above in an appropriate ratio, using a mouse model with dietary obesity.
Seven hundred mg Rapha diet® preparation contains 290mg (41.4%) SPP, 218mg (31.1%) Garcinia cambogia, 112 mg (16%) white bean extract, 21 mg (3%) mango extract, 20 mg (2.9%) raspberry extract, 20 mg (2.9%) cocoa extract, and 19 mg (2.7%) green tea extract.
Eight-week-old male C57BL/6 mice were obtained from Daehan Biolink (Eumseong, Korea). Mice were housed in a room with a temperature of 23±2℃, relative humidity of 55±5%, and an alternating 12-h light (300 lux)/dark cycle with free access to feed and water. All mice were fed a powdered basal diet (D12450B; Research Diets Inc., New Brunswick, USA) and tap water ad libitum for 1 week.
After 1 week of adaptation period, mice were divided into three groups: normal diet (control) group, HFD group, and HFD with Rapha diet® preparation (HFD+Rapha) group. Mice in HFD group were fed HFD (D12451; Research Diets Inc.) ad libitum for 8 weeks. Animals in HFD+Rapha group were fed HFD powder containing 3% (w/w) Rapha diet® preparation. The dose (3%) of Rapha diet® preparation was adopted from previous studies in animals [15] and in humans (unpublished results). Study protocols met the guidelines of the Institutional Animal Care and Use Committee (IACUC) of Laboratory Animal Center at Chungbuk National University, Korea.
Feed intake and body weights were recorded every week. Feed consumption was presented as mean value/day/mouse.
At the end of 8-week treatment, epididymal, perirenal, and mesenteric adipose tissues were weighed after sacrificing mice. Adipose tissue was fixed in 3% formaldehyde solution, and paraffin-embedded sections were stained with hematoxylin-eosin. Using a light microscope (×100 magnification), the mean size of 10 adipocytes was measured using an image analyzer.
Blood was collected from inferior vena cava after overnight fasting. Serum was analyzed for TG, total cholesterols, HDL, and LDL using a blood chemistry analyzer (model 7180; Hitachi Medical Co., Tokyo, Japan).
Liver tissue was fixed in 3% formaldehyde solution, and paraffin-embedded sections were stained with hematoxylin-eosin. Liver sections were examined for the lipid accumulation under a light microscope (×100 magnification).
The results are presented as mean±standard error, and the significance of difference was analyzed using one-2012-12-282012-12-28way analysis of variance followed by Turkey's test at the level of P<0.05. The analyses utilized SPSS statistical software version 12.0 for Windows (SPSS, Inc., Chicago, IL, USA).
The mean daily intake of normal diet was 3.64 g/mouse (Figure 1). The mean consumption of HFD markedly increased 1.5 folds. However, supplementation of Rapha diet® preparation remarkably attenuated the feed consumption to the control level.
Mice fed HFD showed higher body weight gain than mice fed normal diet (Figure 2). Feeding Rapha diet® preparation decreased the body weight gain, leading to body weights of normal diet group at 8 weeks.
The body weights after overnight fasting were 30.95, 35.14, and 31.03 g in normal diet, HFD, and HFD+Rapha groups, respectively. Weights of epididymal, perirenal, and mesenteric fats markedly increased following HFD feeding, which were reverse by Rapha diet® preparation (Figures 3A and 3B). The size of epididymal adipocytes increased significantly in mice fed HFD compared with cells in the normal diet group (Figures 4A and 4B). However, Rapha diet® preparation near-fully inhibited the increases in the adipocytes from all 3 adipose tissues (Figures 4C and 4D).
HFD feeding increased serum TG, TC, and LDL levels, without affecting HDL value (Table 1). However, feeding Rapha diet® preparation inhibited the increases in TG, TC, and LDL, resulting in correction of LDL/HDL ratio: 0.088, 0.224, and 0.080 for normal diet, HFD, and HFD+Rapha groups, respectively.
Livers of mice fed HFD for 8 weeks revealed many lipid droplets. Such hepatic steatosis was attenuated by supplementation with Rapha diet® preparation (Figure 5).
The body weight gain of mice fed HFD markedly increased compared to normal diet. However, Rapha diet® preparation supplemented in the HFD for 8 weeks fully reduced the HFD-induced increase in body weight gain. It can be explained that the attenuated body weight gain comes from the decreased amount of fat tissues as well as the reduced size of adipocytes, which were confirmed from direct measurement of fat weights, and image analysis of adipocytes.
White adipose tissue is composed of adipocytes that play a central role in lipid homeostasis and the maintenance of energy balance [32]. Adipocytes store energy in the form of TG after food intake and release it in the form of free fatty acids during fasting period [33]. Excess white adipose tissue leads to obesity. The expansion of adipose tissue mass when excess food is ingested requires differentiation of adipocytes from precursor cells [34-36]. Adipocyte differentiation from adipose precursor cells has been shown to be arranged by the 2 interdependent transcription factors, peroxisome proliferator-activated receptor-γ (PPAR-γ) and CCAAT enhancer-binding proteins (C/EBP) [35]. SPP is known to suppress mRNA expression of adipogenesis-specific leptin and Acrp30 (adipocyte-derived cytokines), and to lower both PPAR-γ and Acrp30 synthesis [15]. Garcinia cambogia extract was reported to modulate multiple genes associated with adipogenesis as adipocyte protein aP2 (aP2), sterol regulatory element-binding factor 1c (SREBP1c), PPAR-γ2, and C/EBP-α [18]. Also, it has been shown that cocoa extract and green tea extract decreased the expression of PPAR-γ in adipose tissues [28,31]. Raspberry ketone has been reported to stimulate lipolysis in adipocytes as well [25-27]. The norepinephrine and epinephrine are known to stimulate lipolysis via β-adrenergic receptors [25-27]. Raspberry ketone increased norepinephrine-induced lipolysis not via a mechanism related to that of synephrine, but via an increase in the translocation of hormone-sensitive lipase (HSL), which catalyzes TG hydrolysis, from the cytosol to the lipid droplets in adipocytes [25-27]. The conversion of the translocation to its substrate on the surfaces of lipid droplets is a crucial step for TG hydrolysis. Raspberry ketone affects the lipid metabolism by secretion of adiponectin, one of the most important adipocytokines and a regulatory factor in obesity and insulin resistance, in adipocytes as well [25-27].
HFD increased blood lipids and caused hepatic steatosis, as confirmed by microscopic examination. Rapha diet® preparation reversed the increase in blood lipids and their accumulation in the liver. Interestingly, LDL, a major risk factor of atherosclerosis, was significantly decreased to the normal level by Rapha diet® preparation. It was proposed that silk protein hydrolysates have lipid-controlling effects owing to their up-regulating activities on the leptin and insulin secretion [11-15]. Garcinia cambogia contains HCA, a potent competitive inhibitor of ATP citrate lyase [18-20]. Therefore, HCA limits the availability of acetyl-CoA units required for fatty acid or cholesterol synthesis. Mango has been shown to lower insulin resistance, improving glucose tolerance and lipid profile, and to reduce adiposity associated with an HFD [24]. Raspberry ketone also was demonstrated to decrease hepatic TG content in animals fed an HFD [27]. Cocoa extract has been reported to produce extensive decreases in the expression of genes for cholesterol biosynthesis including squalene synthase, squalene epoxidase (monooxygenase), and 7-dehydrocholesterol reductase [28]. It was found that catechins contained in green tea decreased plasma levels of cholesterol, glucose, insulin, and leptin [29]. In addition, green tea catechins increased β-oxidation as determined by palmitic acid oxidation activity [29,31]. Such effects of green tea cathechins on lipid metabolism might be mediated by increased mRNA expressions of acyl-CoA oxidase and medium-chain acyl-CoA dehydro-genase, as demonstrated by increased lipid oxidation, β-oxidation, and expression of fatty acid translocase/CD36 mRNA in skeletal muscles [29,31]. Accordingly, inhibitory activity of Rapha diet® preparation on lipid accumulation might be due to its lipid-burning effect, in addition to anti-adipogenic potential.
The present study demonstrate that supplementation of Rapha diet® preparation attenuates body weight gain of HFD-fed animals not only by decreasing the accumulation of body fats and the size of adipocytes, but also by modulating lipid profiles. Notably, the body weight-controlling effect of Rapha diet® preparation (100%) was superior to SP (35%) or SPP (55%) alone [15]. Such higher effect may be achieved by inhibitory activities on the adipogenesis and lipid accumulation as well as additional appetite-controlling potentials of catechins in green tea [30] and HCA in Garcinia cambogia [37,38]. Since silk proteins enhance physical stamina [16,17], it is proposed that the Rapha diet® preparation could be a promising candidate for the control of dietary obesity without side effects such as energy deficiency-related fatigue, although sex difference in its effectiveness and detailed action mechanisms remain to be clarified.
Figures and Tables
Figure 1
Daily feed consumption (g/mouse) by mice fed normal diet, high-fat diet (HFD) alone or containing 3% Rapha diet® preparation for 8 weeks.
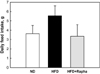
Figure 2
Change in body weights of mice during feeding normal diet (circle), high-fat diet (HFD) alone (triangle) or containing 3% Rapha diet® preparation (square) for 8 weeks. *Significantly different from normal diet (P<0.05). #Significantly different from HFD alone (P<0.05).
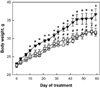
Figure 3
Absolute (A) and relative (B) weights of epididymal, perirenal, and mesentaric fats from mice fed normal diet (white), high-fat diet (HFD) alone (black) or containing 3% Rapha diet® preparation (grey) for 8 weeks. *Significantly different from normal diet (P<0.05). #Significantly different from HFD alone (P<0.05).
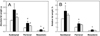
Figure 4
A-C: Representative findings of perirenal adipose tissue of mice fed normal diet (A), high-fat diet (HFD) alone (B) or containing 3% Rapha diet® preparation (C) for 8 weeks. D: Epididymal, perirenal, and mesentaric adipocyte sizes of mice fed normal diet (white), HFD alone (black) or containing 3% Rapha diet® preparation (grey). *Significantly different from normal diet (P<0.05). #Significantly different from HFD alone (P<0.05). Bar=100 µm.
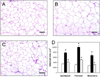
Acknowledgments
This work was supported by Priority Research Centers Program through the National Research Foundation of Korea (NRF) funded by the Ministry of Education, Science and Technology (2009-0094034).
References
1. Popkin BM, Doak CM. The obesity epidemic is a worldwide phenomenon. Nutr Rev. 1998. 56:106–114.
2. Sjöström LV. Mortality of severely obese subjects. Am J Clin Nutr. 1992. 55:2 Suppl. 516S–523S.
3. Lavie CJ, Milani RV, Ventura HO. Obesity and cardiovascular disease; risk factor, paradox, and impact of weight loss. J Am Coll Cardiol. 2009. 53:1925–1932.
4. Steppan CM, Bailey ST, Bhat S, Brown EJ, Banerjee RR, Wright CM, Patel HR, Ahima RS, Lazar MA. The hormone resistin links obesity to diabetes. Nature. 2001. 409(6818):307–312.
5. Calle EE, Kaaks R. Overweight, obesity and cancer: epidemiological evidence and proposed mechanisms. Nat Rev Cancer. 2004. 4(8):579–591.
6. Racette SB, Deusinger SS, Deusinger RH. Obesity: overview of prevalence, etiology, and treatment. Phys Ther. 2003. 83(3):276–288.
7. Smith C, Marks A, Lieberman M. Marks' Basic Medical Biochemistry: A Clinical Approach. 2004. 2nd ed. Baltimore: Lippincott Williams & Wilkins;579–681.
8. Curioni CC, Lourenço PM. Long-term weight loss after diet and exercise: a systematic review. Int J Obes (Lond). 2005. 29(10):1168–1174.
9. Cooke D, Bloom S. The obesity pipeline: current strategies in the development of anti-obesity drugs. Nat Rev Drug Discov. 2006. 5(11):919–931.
10. Ioannides-Demos LL, Proietto J, Tonkin AM, McNeil JJ. Safety of drug therapies used for weight loss and treatment of obesity. Drug Saf. 2006. 29(4):277–302.
11. Shin M, Park M, Youn M, Lee Y, Nam M, Park I, Jeong Y. Effects of silk protein hydrolysates on blood glucose and serum lipid in db/db diabetic mice. J Korean Soc Food Sci Nutr. 2006. 35:1343–1348.
12. Lee Y, Park M, Choi J, Kim J, Nam M, Jeong Y. Effects of silk protein hydrolysates on blood glucose level, serum insulin and leptin secretion in OLEFT rats. J Korean Soc Food Sci Nutr. 2007. 36:703–707.
13. Jung EY, Lee HS, Lee HJ, Kim JM, Lee KW, Suh HJ. Feeding silk protein hydrolysates to C57BL/KsJ-db/db mice improves blood glucose and lipid profiles. Nutr Res. 2010. 30(11):783–790.
14. Kim TM, Ryu JM, Seo IK, Lee KM, Yeon S, Kang S, Hwang SY, Kim YB. Effects of red ginseng powder and silk peptide on hypercholesterolemia and atherosclerosis in rabbits. Lab Anim Res. 2008. 24:67–75.
15. Lee SH, Park D, Yang G, Bae DK, Yang YH, Kim TK, Kim D, Kyung J, Yeon S, Koo KC, Lee JY, Hwang SY, Joo SS, Kim YB. Silk and silkworm pupa peptides suppress adipogenesis in preadipocytes and fat accumulation in rats fed a high-fat diet. Eur J Nutr. 2012. 51(8):1011–1019.
16. Shin S, Park D, Yeon S, Jeon JH, Kim TK, Joo SS, Lim WT, Lee JY, Kim YB. Stamina-enhancing effects of silk amino acid preparations in mice. Lab Anim Res. 2009. 25:127–134.
17. Shin S, Yeon S, Park D, Oh J, Kang H, Kim S, Joo SS, Lim WT, Lee JY, Choi KC, Kim KY, Kim SU, Kim JC, Kim YB. Silk amino acids improve physical stamina and male reproductive function of mice. Biol Pharm Bull. 2010. 33(2):273–278.
18. Kim KY, Lee HN, Kim YJ, Park T. Garcinia cambogia extract ameliorates visceral adiposity in C57BL/6J mice fed on a high-fat diet. Biosci Biotechnol Biochem. 2008. 72(7):1772–1780.
19. Hayamizu K, Hirakawa H, Oikawa D, Nakanishi T, Takagi T, Tachibana T, Furuse M. Effect of Garcinia cambogia extract on serum leptin and insulin in mice. Fitoterapia. 2003. 74(3):267–273.
20. Jena BS, Jayaprakasha GK, Singh RP, Sakariah KK. Chemistry and biochemistry of (-)-hydroxycitric acid from Garcinia. J Agric Food Chem. 2002. 50(1):10–22.
21. Fantini N, Cabras C, Lobina C, Colombo G, Gessa GL, Riva A, Donzelli F, Morazzoni P, Bombardelli E, Carai MA. Reducing effect of a Phaseolus vulgaris dry extract on food intake, body weight, and glycemia in rats. J Agric Food Chem. 2009. 57(19):9316–9323.
22. Barrett ML, Udani JK. A proprietary alpha-amylase inhibitor from white bean (Phaseolus vulgaris): a review of clinical studies on weight loss and glycemic control. Nutr J. 2011. 10:24.
23. Masibo M, He Q. Major mango polyphenols and their potential significance to human health. Compr Rev Food Sci Food Saf. 2008. 7:309–319.
24. Lucas EA, Li W, Peterson SK, Brown A, Kuvibidila S, Perkins-Veazie P, Clarke SL, Smith BJ. Mango modulates body fat and plasma glucose and lipids in mice fed a high-fat diet. Br J Nutr. 2011. 106(10):1495–1505.
25. Morimoto C, Satoh Y, Hara M, Inoue S, Tsujita T, Okuda H. Anti-obese action of raspberry ketone. Life Sci. 2005. 77(2):194–204.
26. Park KS. Raspberry ketone increases both lipolysis and fatty acid oxidation in 3T3-L1 adipocytes. Planta Med. 2010. 76(15):1654–1658.
27. Suh JH, Romain C, González-Barrio R, Cristol JP, Teissèdre PL, Crozier A, Rouanet JM. Raspberry juice consumption, oxidative stress and reduction of atherosclerosis risk factors in hypercholesterolemic golden Syrian hamsters. Food Funct. 2011. 2(7):400–405.
28. Matsui N, Ito R, Nishimura E, Yoshikawa M, Kato M, Kamei M, Shibata H, Matsumoto I, Abe K, Hashizume S. Ingested cocoa can prevent high-fat diet-induced obesity by regulating the expression of genes for fatty acid metabolism. Nutrition. 2005. 21(5):594–601.
29. Murase T, Nagasawa A, Suzuki J, Hase T, Tokimitsu I. Beneficial effects of tea catechins on diet-induced obesity: stimulation of lipid catabolism in the liver. Int J Obes Relat Metab Disord. 2002. 26(11):1459–1464.
30. Wolfram S, Wang Y, Thielecke F. Anti-obesity effects of green tea: from bedside to bench. Mol Nutr Food Res. 2006. 50(2):176–187.
31. Ashida H, Furuyashiki T, Nagayasu H, Bessho H, Sakakibara H, Hashimoto T, Kanazawa K. Anti-obesity actions of green tea: possible involvements in modulation of the glucose uptake system and suppression of the adipogenesis-related transcription factors. BioFactors. 2004. 22(1-4):135–140.
32. Fajas L, Auboeuf D, Raspé E, Schoonjans K, Lefebvre AM, Saladin R, Najib J, Laville M, Fruchart JC, Deeb S, Vidal-Puig A, Flier J, Briggs MR, Staels B, Vidal H, Auwerx J. The organization, promoter analysis, and expression of the human PPARgamma gene. J Biol Chem. 1997. 272(30):18779–18789.
33. Li J, Yu X, Pan W, Unger RH. Gene expression profile of rat adipose tissue at the onset of high-fat-diet obesity. Am J Physiol Endocrinol Metab. 2002. 282(6):E1334–E1341.
34. Wahli W, Braissant O, Desvercne B. Peroxisome proliferator activated receptors: transcriptional regulators of adipogenesis, lipid metabolism and more.... Chem Biol. 1995. 2(5):261–266.
35. Schoonjans K, Staels B, Auwerx J. The peroxisome proliferator activated receptors (PPARS) and their effects on lipid metabolism and adipocyte differentiation. Biochim Biophys Acta. 1996. 1302(2):93–109.
36. Mandrup S, Lane MD. Regulating adipogenesis. J Biol Chem. 1997. 272(9):5367–5370.
37. Preuss HG, Bagchi D, Bagchi M, Rao CV, Dey DK, Satyanarayana S. Effects of a natural extract of (-)-hydroxycitric acid (HCA-SX) and a combination of HCA-SX plus niacin-bound chromium and Gymnema sylvestre extract on weight loss. Diabetes Obes Metab. 2004. 6(3):171–180.
38. Hasani-Ranjbar S, Nayebi N, Larijani B, Abdollahi M. A systematic review of the efficacy and safety of herbal medicines used in the treatment of obesity. World J Gastroenterol. 2009. 15(25):3073–3085.