Abstract
Gangliosides are ubiquitous components of the membranes of mammalian cells that are thought to play important roles in various cell functions such as cell-cell interaction, cell adhesion, cell differentiation, growth control, and signaling. However, the role that gangliosides play in the immune rejection response after xenotransplantation is not yet clearly understood. In this study, the regulatory effects of human leukocytes on ganglioside expression in primary cultured micro-pig aortic endothelial cells (PAECs) were investigated. To determine the impact of human leukocytes on the expression of gangliosides in PAECs, we performed high-performance thin layer chromatography (HPTLC) in PAECs incubated with FBS, FBS containing human leukocytes, human serum containing human leukocytes, and FBS containing TNF-α. Both HPTLC and immunohistochemistry analyses revealed that PAECs incubated with FBS predominantly express the gangliosides GM3, GM1, and GD3. However, the expression of GM1 significantly decreased in PAECs incubated for 5 h with TNF-α (10 ng/mL), 10% human serum containing human leukocytes, and 10% FBS containing human leukocytes. Taken together, these results suggest that human leukocytes induced changes in the expression profile of ganglioside GM1 similar to those seen upon treatment of PAECs with TNF-α. This finding may be relevant for designing future therapeutic strategies intended to prolong xenograft survival.
Xenotransplantation of pig organs into human is regarded as an attractive strategy to meet the increasing organ demand for human transplantation [1-4]. However, in discordant species combinations, vascularized organ xenografts are engorged and discolored with loss of function within minutes to hours after transplantation. This type of rejection is called hyper-acute rejection (HAR) and has long been considered the primary barrier to xenotransplantation.
The endothelium is the major target in HAR. Nontranscriptional (type I) endothelial cell (EC) activation occurs upon antibody binding [5]. This activation is rapid and leads to EC retraction, expression of P-selectin (CD62P), secretion of platelet activating factor (PAF), and loss of the antithrombotic phenotype [5,6]. The observed EC retraction may be caused by rapid redistribution of platelet/endothelial cell adhesion molecule (PECAM-1/CD31) away from cell junctions because of α-Gal cross-linking [7]. If HAR is prevented, the vascularized grafts are generally lost due to acute vascular rejection (AVR) characterized by type II (transcriptional) EC activation, thrombosis with fibrin deposition, and cellular infiltration dominated by monocyte/macrophages, natural killer (NK) cells, and neutrophils. Neutrophils are the most abundant leukocyte circulating in the body, representing 50-70% of the total number of leukocytes, and are implicated in both HAR and AVR of xenografts [8,9]. However, the exact role of leukocytes in xenograft rejection has not yet been established. One of approach to inhibit AVR is to target glycosphingolipids, specifically gangliosides. Gangliosides are localized on the plasma membrane and have previously been shown to modulate epithelial cell immunosuppressive activity [10-12].
In this study, we investigated the regulatory roles of human leukocytes in the expression of gangliosides in primary cultures of micro-pig aortic endothelial cells (PAECs) using high-performance thin layer chromatography (HPTLC) and immunocytochemistry analyses.
Monoclonal antibodies (mAbs) specific for gangliosides GM3, GM2, GM1, GD3, GD1a, GD1b, GT1b and GQ1b (Seikagaku Co., Tokyo, Japan) were used in an initial screen of ganglioside expression in this study. PAECs were appreciably positive for the MAbs GMR6, GMB16, and GMR19, which correspond to gangliosides GM3, GM1, and GD3, respectively. Therefore, these 3 MAbs were used in further experiments. The production and characterization of these MAbs have previously been described [13-16].
The methods used to extract and purify gangliosides have been previously described [17]. Briefly, the cells were homogenized in distilled water at 48℃ to extract total lipids, which were resuspended in chloroform/methanol (1:1, v/v), then lyophilized using N2 gas, and subsequently dissolved in chloroform/methanol/H2O (15:30:4, v/v/v). The dissolved total lipid samples were then applied to a DEAE Sephadex A25 column (Sigma, St. Louis, MO, USA). The column was subsequently washed to remove neutral lipids with chloroform/methanol/H2O (15:30:4, v/v/v), and acidic lipids were then eluted by adding chloroform/methanol/sodium acetate (15:30:4, v/v/v). The eluted samples were dried at 30℃ under N2 for 5 h, after which they were dissolved in chloroform/methanol (1:1, v/v) and alkalized in 12 N ammonium hydroxide. Next, the acidic lipid samples dissolved in chloroform/methanol (1:1, v/v) were applied to a Sep-Pak C18 cartridge column (Water, Ireland). The column was washed with H2O to remove non-hydrophobic species of lipids. Finally, the gangliosides were eluted with methanol, dried at 30℃ under N2 for 3 h, and stored at -80℃ until analysis.
HPTLC analysis of the gangliosides was conducted using a 10×10 cm thin-layer chromatography (TLC) 5651 plate (Merck, Darmstadt, Germany) as described in a previous study [15]. The purified gangliosides (600 µg protein/lane) were loaded onto TLC 5651 plates that were subsequently developed in chloroform/methanol/0.25% CaCl2 · H2O (50:40:10, v/v/v). The gangliosides were then stained with resorcinol, after which the densities of the ganglioside bands were quantified by HPTLC densitometry (Beta 4.0.3 from Scion Image, Frederick, MD, USA). The mixture of purified gangliosides GM3, GM2, GM1, GD3, GD1a, and GD1b (Matreya LLC, Pleasant Gap, PA, USA) were used as standards.
Tissues of SPF micro-pigs® used in this experiment were obtained from the Medi Kinetics Co (Pyongtaek, Korea). All animal experiments were conducted according to Standard Operation Procedures and were approved by the Institutional Animal Care and Use Committee of Wonkwang University, Korea (Approval No. WKU-IACUC: 11-14).
Fresh aortae were removed from the heart within 1 h of sacrifice and gently rinsed with sterile phosphate-buffered saline (PBS) after removing the connective and adipose tissue around the aorta. Aortae were fixed in buffered formalin (0.1 M sodium phosphate, 10% formalin, pH 7.4) for 24 h at 40℃. After an overnight rinse in 0.1 M sodium phosphate (pH 7.4) at 40℃, the aortae were dehydrated with graded ethanol and embedded in paraffin. Paraffin sections (4 µm thickness) were cut, deparaffinized with xylene, and rinsed thoroughly with absolute ethanol. Paraffin-embedded sections of the micro-pig aorta were stained with hematoxylin and eosin for histopathological evaluation.
The expression of gangliosides in the micro-pig aorta was determined by indirect immunofluorescence microscopy of frozen sections. Serial sections (4 µm thickness) cut with a cryostat microtome were thaw-mounted on albumin-coated glass slides. The mounted sections were then air-dried for 2 h, after which they were fixed with acetone at -20℃ for 5 min [17]. Sections were washed twice with PBS for 10 min and then incubated with 5% bovine serum albumin (BSA, Sigma, St. Louis, MO, USA) in PBS for 15 min at room temperature. Next, the sections were incubated with a mouse mAb against a specific ganglioside (GM3, GM2, GM1, GD3, GD1a, GD1b, GT1b and GQ1b; Seikagaku Co., Tokyo, Japan) diluted in PBS containing 5% BSA overnight at 4℃. They were then washed with cold PBS 4 times, after which they were incubated with fluorescein isothiocyanate (FITC)-conjugated goat anti-mouse IgM antibody (Sigma, St. Louis, MO, USA) diluted to 1:500 in PBS for 1 h, and then washed with PBS 5 times. To identify the nuclei, 1 µL/mL of a DNA-specific fluorescent dye (Hoechst 33342, Sigma) was added. The sections were sealed with a coverslip and observed under a fluorescence microscope (Model FV300, Olympus, Tokyo, Japan).
Primary cultures of PAECs were prepared using a modification of the method of Ohbayashi et al. [18]. The aorta was washed in Dulbecco's PBS (DPBS, Invitrogen, Carlsbad, CA) containing 1% antibiotic-antimycotic (GIBCO, Carlsbad, CA). After removing the connective and adipose tissue, the aorta was cut into 5- to 6-cm-long pieces that were opened longitudinally and laid endothelium side down onto tissue culture dishes coated with 130 U/mL collagenase (collagenase type I; Sigma, St. Louis, MO, USA) in DPBS for 20 min at 37℃. At the end of the incubation, the luminal surfaces of the tissue fragments were rinsed with 40 mL of Medium 199 (Sigma, St. Louis, MO, USA) containing 10% FBS (GIBCO, Carlsbad, CA). The cells were then centrifuged (200×g for 10 min). The cell pellet was resuspended in Medium 199 supplemented with 4500 mg/L glucose, L-glutamine, and sodium pyruvate (Sigma, St. Louis, MO, USA), 2.2 g/L sodium bicarbonate (Sigma, St. Louis, MO, USA), 1% antibiotic-antimycotic (GIBCO, Carlsbad, CA), and 10% FBS (GIBCO, Carlsbad, CA) and plated into 6-well tissue culture plates coated with 0.2% porcine gelatin (Sigma, St. Louis, MO, USA). Cultures were grown at 37℃ in 5% CO2/95% air. Confluent PAECs were routinely used for experiments between the first and fifth passage. Cultured cells were identified as endothelial by their morphology, and the presence of CD106 (anti-porcine E-selectin, Antigenix America Inc., Melville, NY, USA) and CD62E (anti-porcine VCAM-1; Vascular cell adhesion molecule-1, Antigenix America Inc.) evaluated by fluorescence microscope [19].
PBMCs were prepared from human fresh venous blood collected from healthy volunteers. After proper dilution in PBS containing 5% FBS and 2 mmol/L ethylenediaminetetraacetic acid (EDTA, Sigma, St. Louis, MO, USA), the blood was separated using Ficoll-Paque™ PLUS (GE Healthcare, Buckinghamshire, UK) gradient centrifugation. The leukocyte-containing buffy-coat interfaces were collected, washed twice with the above dilute solution, and finally resuspended in culture medium. The viability of isolated PBMCs always exceeded 95% as detected by trypan blue exclusion [20].
Cells were washed twice with PBS for 10 min, permeabilized with 0.25% Triton X-100 (Sigma, St. Louis, MO, USA) for 10 min at 37℃, and finally fixed in 4% paraformaldehyde in PBS for 30 min at room temperature. The samples were then incubated with 5% BSA in PBS for 15 min at room temperature, washed twice with PBS, and then incubated with mouse mAb diluted in PBS containing 5% BSA overnight at 4℃. Next, the samples were washed with cold PBS 4 times, incubated with FITC-conjugated goat anti-mouse IgM antibody (Sigma, St. Louis, MO, USA) diluted in PBS to 1:500 for 1 h, and then washed 5 times with PBS. To identify nuclei, 1 µL/mL of Hoechst 33342 (Sigma, St. Louis, MO, USA) was added. The sections were sealed with a coverslip and observed under a confocal scanning laser fluorescence microscope.
Histological analysis of the aorta, conducted using hematoxylin and eosin staining of micro-pig aorta sections (4 µm thickness), clearly revealed the endothelium, tunica media, and tunica adventitia (supplement 1).
Nuclear staining and confocal scanning laser fluorescence microscopy showed the expression patterns and localization of gangliosides in the micro-pig aorta. Micro-pig aortae stained with Hoechst 33342 to detect DNA and 8 MAbs specific for GM3, GM2, GM1, GD3, GD1a, GD1b, GT1b, and GQ1b were screened. The results suggest that GM3, GM1, and GD3, which correspond to the antibodies GMR6, GMB16, and GMR19, are the major gangliosides in the micro-pig aortal endothelium (Figure 1).
PAECs were isolated from micro-pig aortae by digestion with collagenase type I and used for primary culture. Isolated PAECs were identified as endothelial based on their morphology on GIEMSA staining (Figure 2A), and the presence of E-selectin/CD106 (FITC; green) and VCAM-1/CD62E (phycoerythrin; red), which are well known endothelial cell markers. The cells were also stained with Hoechst 33342 to detect DNA (Figure 2B).
HPTLC analysis was conducted to analyze the expression of gangliosides in PAECs incubated for 5 h with 10% FBS, 10% FBS containing human leukocytes, 10% human serum containing human leukocytes, and 10% FBS containing TNF-α (10 ng/mL). The gangliosides GM3, GM1, and GD3 were readily detected in PAECs as in micro-pig aortal endothelium. However, the relative thicknesses of the bands corresponding to gangliosides obtained from PAECs cultured with 10% FBS containing human leukocytes, 10% human serum containing human leukocytes, and 10% FBS containing TNF-α (10 ng/mL) revealed that their expression differed from that seen upon culture with 10% FBS alone. As shown in Figure 3A, the expression of the gangliosides GM3 and GD3 was unchanged by the presence of human leukocytes or TNF-α, but ganglioside GM1 expression was obviously lower in PAECs incubated with 10% FBS containing human leukocytes, 10% human serum containing human leukocytes, and 10% FBS containing TNF-α (10 ng/mL). Taken together, these results suggest that human leukocytes induced expressional pattern changes in the ganglioside GM1 similar to those seen upon treatment of PAECs with TNF-α. The relative amounts of ganglioside expression in PAECs were measured by densitometry, as shown in Figure 3B.
Using immunohistochemical staining methods, the expressional patterns and localization of gangliosides in PAECs were investigated. Three MAbs, GMR6, GMB16, and GMR19, specific for gangliosides GM3, GM1, and GD3, respectively, were appreciably reactive in PAECs that were also stained with Hoechst 33342 to detect DNA (Figure 4A). However ganglioside GM1 expression was obviously lower in PAECs incubated with 10% FBS containing human leukocytes, 10% human serum containing human leukocytes, and 10% FBS containing TNF-α (10 ng/mL) (Figure 4B). These results are in accordance with the HPTLC findings (Figure 3A and B).
The endothelium is the first site of contact between a vascularized xenograft and the recipient's immune system. Gangliosides are ubiquitous components of the membranes of mammalian cells, and are thought to have roles in cell proliferation, adhesion, migration, differentiation, survival, and immunosuppressive activity [10,11,21-31] however, their role in the immune rejection response to xenotransplantation is not understood.
Morigi et al. showed that xenogeneic serum is a potent promoter of leukocyte adhesion and transmigration in porcine aortic endothelial cells under flow, and that complement deposited on porcine endothelium is responsible for the early adhesion and transmigration of leukocytes. They went on to demonstrate that these adhesive events are later regulated by endothelial activation of NF-κB dependent genes, and that TNF-α is one of the most potent inducers of endothelial cell adhesive properties [32].
Therefore, we studied the role of human leukocytes in the regulation of ganglioside expression in PAECs, as an in vitro model of a vascular xenograft. Hematoxylin and eosin staining of micro-pig aorta sections clearly showed the endothelium, tunica media and tunica adventitia (Supplement 1), and revealed that the gangliosides GM3, GM1 and GD3, which correspond to the mAbs GMR6, GMB16, and GMR19, are the major gnagliosides in micro-pig aortal endothelium (Figure 1). To determine the impact of human leukocytes on ganglioside expression in PAECs, these cells were isolated from micro-pig aortae (Figure 2). Isolated PAECs were identified as endothelial based on their morphology and the expression of VCAM-1/CD106 or E-selectin/CD62E, well-established endothelial cell markers (Figure 2B). Subsequent HPTLC analysis provided a profile of the gangliosides present in porcine aortic endothelium, which was appreciably reactive to the MAbs GMR6, GMB16, and GMR19, which correspond to gangliosides GM3, GM1, and GD3, respectively (Figure 3A). Finally to determine whether human leukocytes have an impact on the expression profiles of gangliosides in PAECs, we performed HPTLC in PAECs incubated for 5 h with 10% FBS, 10% FBS containing human leukocytes, 10% human serum containing human leukocytes, and 10% FBS containing TNF-α (10 ng/mL). Both HPTLC and immunohistochemistry analyses revealed that the expression of ganglioside GM1 was significantly lower in PAECs incubated for 5 h with 10% FBS containing human leukocytes, 10% human serum containing human leukocytes, and 10% FBS containing TNF-α (10 ng/mL) (Figure 3 and 4B).
Taken together, these results suggest that human leukocytes induce changes in the expression pattern of ganglioside GM1, similar to those seen upon treatment of PAECs with TNF-α. These findings may be relevant for designing future therapeutic strategies intended to prolong xenograft survival.
Figures and Tables
Figure 1
Immunofluorescence staining of micro-pig aorta sections. Sections were immunostained with GMR6 (anti-GM3 MAb), GMB28 (anti-GM2 MAb), GMB16 (anti-GM1 MAb), GMR19 (anti-GD3 MAb), GMR17 (anti-GD1a MAb), GGR12 (anti-GD1b MAb), GMR5 (anti-GT1b MAb), GMR13 (anti-GQ1b MAb), and fluorescein isothiocyanate (FITC)-labeled goat anti-mouse IgM antibody, and then stained with Hoechst 33342 for DNA. The bar represents 50 µm.
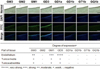
Figure 2
Characterization and GIEMSA staining of micro-pig aortic endothelial cells. Isolated micro-pig aortic endothelial cells (PAECs) were identified as endothelial cells (A) based on their morphology after Giemsa staining, and (B) by the presence of E-selectin/CD106 and VCAM-1/CD62E, well-known endothelial cell markers. The bar represents 50 µm.
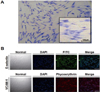
Figure 3
High-performance thin layer chromatography and quantitative analysis of ganglioside expression in micro-pig aortic endothelial cells. (A) Gangliosides isolated from micro-pig aortic endothelial cells (PAECs) were separated by high-performance thin layer chromatography (HPTLC) silica gel plates using chloroform:methanol:0.25% CaCl2 (50:40:10, v/v/v), and gangliosides were visualized using resorcinol solution. Lane 1 (M1) and 2 (M2), ganglioside standard markers; lane 3, 10% FBS; lane 4, 10% FBS containing human leukocytes; lane 5, 10% human serum containing human leukocytes; lane 6, 10% FBS containing TNF-α (10 ng/mL). The results are representative of at least 3 independent experiments. (B) Quantitative analysis of ganglioside expression was performed using with the Beta 4.0.3 densitometry program (Scion Image, Frederick, MD, USA). Data are expressed as mean±SD; n=3; ***P<0.001.
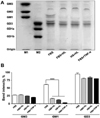
Figure 4
Immunofluorescence staining of micro-pig aortic endothelial cells. (A) Normal PAECs were immunostained with GMR6 (anti-GM3 MAb), GMB16 (anti-GM1 MAb), GMR19 (anti-GD3 MAb), and FITC-labeled goat anti-mouse IgM antibody and then stained with Hoechst 33342 to detect DNA. (B) Normal PAECs and PAECs-incubated with FBS containing human leukocytes, with leukocyte-containing 10% human serum, or with FBS containing TNF-α (10 ng/mL) were immunostained with GMB16 (anti-GM1 MAb), and FITC-labeled goat anti-mouse IgM antibody, and then stained with Hoechst 33342 to detect DNA. The bar represents 50 µm.
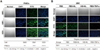
Acknowledgments
This work was supported by a research grant from the Ministry of Education, Science, and Technology (2010-0022316 and KCG 5401011), Korea.
References
1. Cozzi E, White DJ. Xenotransplantation. Curr Opin Nephrol Hypertens. 1996. 5(6):514–518.
2. Hancock WW. The past, present, and future of renal xenotransplantation. Kidney Int. 1997. 51(3):932–944.
3. Parker W, Saadi S, Lin SS, Holzknecht ZE, Bustos M, Platt JL. Transplantation of discordant xenografts: a challenge revisited. Immunol Today. 1996. 17(8):373–378.
4. Platt JL. The prospects for xenotransplantation of the kidney. Curr Opin Nephrol Hypertens. 1997. 6(3):284–291.
5. Bach FH, Robson SC, Winkler H, Ferran C, Stuhlmeier KM, Wrighton CJ, Hancock WW. Barriers to xenotransplantation. Nat Med. 1995. 1(9):869–873.
6. Platt JL, Vercellotti GM, Lindman BJ, Oegema TR Jr, Bach FH, Dalmasso AP. Release of heparan sulfate from endothelial cells. Implications for pathogenesis of hyperacute rejection. J Exp Med. 1990. 171(4):1363–1368.
7. Nasu K, Whyte A, Green SJ, Evans PC, Kilshaw PJ. A Alpha-galactosyl-mediated activation of porcine endothelial cells: studies on CD31 and VE-cadherin in adhesion and signaling. Transplantation. 1999. 68(6):861–867.
8. Leventhal JR, Matas AJ, Sun LH, Reif S, Bolman RM 3rd, Dalmasso AP, Platt JL. The immunopathology of cardiac xenograft rejection in the guinea pig-to-rat model. Transplantation. 1993. 56(1):1–8.
9. Mejía-Laguna JE, Martínez-Palomo A, López-Soriano F, García-Cornjeo M, Biro CE. Prolonged survival of kidney xenografts in leucopenic rabbits. Immunology. 1971. 21(6):879–882.
10. Bergelson LD, Dyatlovitskaya EV, Klyuchareva TE, Kryukova EV, Lemenovskaya AF, Matveeva VA, Sinitsyna EV. The role of glycosphingolipids in natural immunity. Gangliosides modulate the cytotoxicity of natural killer cells. Eur J Immunol. 1989. 19(11):1979–1983.
11. Grayson G, Ladisch S. Immunosuppression by human gangliosides. II. Carbohydrate structure and inhibition of human NK activity. Cell Immunol. 1992. 139(1):18–29.
12. Ladisch S, Gillard B, Wong C, Ulsh L. Shedding and immunoregulatory activity of YAC-1 lymphoma cell gangliosides. Cancer Res. 1983. 43(8):3808–3813.
13. Kanda N, Tamaki K. Ganglioside GQ1b enhances Ig production by human PBMCs. J Allergy Clin Immunol. 1998. 102(5):813–820.
14. Kotani M, Kawashima I, Ozawa H, Terashima T, Tai T. Differential distribution of major gangliosides in rat central nervous system detected by specific monoclonal antibodies. Glycobiology. 1993. 3(2):137–146.
15. Ladisch S, Gillard B. A solvent partition method for microscale ganglioside purification. Anal Biochem. 1985. 146(1):220–231.
16. Ozawa H, Kotani M, Kawashima I, Tai T. Generation of one set of monoclonal antibodies specific for b-pathway ganglio-series gangliosides. Biochim Biophys Acta. 1992. 1123(2):184–190.
17. Graus F, Cordon-Cardo C, Houghton AN, Melamed MR, Old LJ. Distribution of the ganglioside GD3 in the human nervous system detected by R24 mouse monoclonal antibody. Brain Res. 1984. 324(1):190–194.
18. Ohbayashi A, Hiraga T, Okubo M, Murase T, Matsushita H, Hara M. Characteristics of porcine coronary artery endothelial cells in culture: comparison with aortic endothelium. Biochem Biophys Res Commun. 1994. 202(1):504–511.
19. Vercellotti GM, Platt JL, Bach FH, Dalmasso AP. Neutrophil adhesion to xenogeneic endothelium via iC3b. J Immunol. 1991. 146(2):730–734.
20. Zhang X, Feng Z, Feng M, Wang H, Ban L. Adhesion of subsets of human blood mononuclear cells to porcine endothelial cells. Chinese Science Bulletin. 2000. 45(7):626–630.
21. Cho JH, Kim JS, Lee YC, Oh KB, Kwak DH, Kim WS, Hwang SS, Ko K, Chang KT, Choo YK. Differential expression patterns of gangliosides in the tissues and cells of NIH-mini pig kidneys. Animal Cells Syst. 2010. 14(2):83–89.
22. Lee DH, Koo DB, Ko K, Ko K, Kim SM, Jung JU, Ryu JS, Jin JW, Yang HJ, Do SI, Jung KY, Choo YK. Effects of daunorubicin on ganglioside expression and neuronal differentiation of mouse embryonic stem cells. Biochem Biophys Res Commun. 2007. 362(2):313–318.
23. Magnusson S, Strokan V, Svensson L, Månsson JE, Rydberg L, Breimer ME. Expression of carbohydrate xenoantigens on porcine peripheral nerve. Xenotransplantation. 2005. 12(1):49–58.
24. Phelps CJ, Koike C, Vaught TD, Boone J, Wells KD, Chen SH, Ball S, Specht SM, Polejaeva IA, Monahan JA, Jobst PM, Sharma SB, Lamborn AE, Garst AS, Moore M, Demetris AJ, Rudert WA, Bottino R, Bertera S, Trucco M, Starzl TE, Dai Y, Ayares DL. Production of alpha 1,3-galactosyltransferase-deficient pigs. Science. 2003. 299(5605):411–414.
25. Rogers CS, Hao Y, Rokhlina T, Samuel M, Stoltz DA, Li Y, Petroff E, Vermeer DW, Kabel AC, Yan Z, Spate L, Wax D, Murphy CN, Rieke A, Whitworth K, Linville ML, Korte SW, Engelhardt JF, Welsh MJ, Prather RS. Production of CFTR-null and CFTR-DeltaF508 heterozygous pigs by adeno-associated virus-mediated gene targeting and somatic cell nuclear transfer. J Clin Invest. 2008. 118(4):1571–1577.
26. Rogers CS, Stoltz DA, Meyerholz DK, Ostedgaard LS, Rokhlina T, Taft PJ, Rogan MP, Pezzulo AA, Karp PH, Itani OA, Kabel AC, Wohlford-Lenane CL, Davis GJ, Hanfland RA, Smith TL, Samuel M, Wax D, Murphy CN, Rieke A, Whitworth K, Uc A, Starner TD, Brogden KA, Shilyansky J, McCray PB Jr, Zabner J, Prather RS, Welsh MJ. Disruption of the CFTR gene produces a model of cystic fibrosis in newborn pigs. Science. 2008. 321(5897):1837–1841.
27. Rydberg L, Holgersson J, Samuelsson BE, Breimer ME. alpha-Gal epitopes in animal tissue glycoproteins and glycolipids. Subcell Biochem. 1999. 32:107–125.
28. Sachs DH, Leight G, Cone J, Schwarz S, Stuart L, Rosenberg S. Transplantation in miniature swine. I. Fixation of the major histocompatibility complex. Transplantation. 1976. 22(6):559–567.
29. Sun P, Wang XQ, Lopatka K, Bangash S, Paller AS. Ganglioside loss promotes survival primarily by activating integrin-linked kinase/Akt without phosphoinositide 3-OH kinase signaling. J Invest Dermatol. 2002. 119(1):107–117.
30. Sung CC, O'Toole EA, Lannutti BJ, Hunt J, O'Gorman M, Woodley DT, Paller AS. Integrin alpha 5 beta 1 expression is required for inhibition of keratinocyte migration by ganglioside GT1b. Exp Cell Res. 1998. 239(2):311–319.
31. Varki A, Cummings RD, Esko JD, Freeze HH, Stanley P, Marth JD, Bertozzi CR, Hart GW, Etzler ME. Symbol nomenclature for glycan representation. Proteomics. 2009. 9(24):5398–5399.
32. Morigi M, Zoja C, Colleoni S, Angioletti S, Imberti B, Donadelli R, Remuzzi A, Remuzzi G. Xenogeneic serum promotes leukocyte-endothelium interaction under flow through two temporally distinct pathways: role of complement and nuclear factor-kappaB. J Am Soc Nephrol. 1999. 10(10):2197–2207.