Abstract
Pain symptoms are a common complication of diabetic peripheral neuropathy or an inflammatory condition. In the most experiments, only one or two evident pain modalities are observed at diabetic peripheral neuropathy according to experimental conditions. Following diabetic peripheral neuropathy or inflammation, spinal glial activation may be considered as an important mediator in the development of pain. For this reason, the present study was aimed to address the induction of pain modalities and spinal glial expression after streptozotocin injection as compared with that of zymosan inflammation in the rat. Evaluation of pain behavior by either thermal or mechanical stimuli was performed at 3 weeks or 5 hours after either intravenous streptozotocin or zymosan. Degrees of pain were divided into 4 groups: severe, moderate, mild, and non-pain induction. On the mechanical allodynia test, zymosan evoked predominantly a severe type of pain, whereas streptozotocin induced a weak degree of pain (severe+moderate: 57.1%). Although zymosan did not evoke cold allodynia, streptozotocin evoked stronger pain behavior, compared with zymosan (severe+moderate: 50.0%). On the other hand, the high incidence of thermal hyperalgesia (severe+moderate: 90.0%) and mechanical hyperalgesia (severe+moderate: 85.7%) by streptozotocin was observed, as similar to that of zymosan. In the spinal cord, the increase of microglia and astrocyte was evident by streptozotocin, only microglia was activated by zymosan. Therefore, it is recommended that the selection of mechanical and thermal hyperalgesia is suitable for the evaluation of streptozotocin induced diabetic peripheral neuropathy. Moreover, spinal glial activation may be considered an important factor.
Diabetic peripheral neuropathy is one of the major complications associated with both type 1 and type 2 diabetes. Understanding the biochemical mechanisms underlying diabetic neuropathic pain and sensory disorders requires preclinical studies in animal models. The streptozotocin-induced diabetic rat, the most extensively studied animal model of type 1 diabetes, shares a number of features with human diabetic peripheral neuropathy [1]. However, diabetic rats have a limited life span and rarely show evidence of overt neuropathy, such as demyelination, axonal degeneration, fiber loss, or axonal regeneration in their peripheral nerves [2]. This makes diabetic rodents unsuitable for studying the contribution of these phenomena to advanced diabetic peripheral neuropathy to pain or to loss of sensory function.
In animal models, it is not possible to quantify spontaneous pain regarding human diabetic peripheral neuropathy. Despite these limitations, the assessment of behavioral responses to external stimuli in diabetic rats has led to identification of a number of abnormal sensation and pain in diabetes [2]. Several behavioral assays are described, which are routinely used to assess different aspects of neuropathic pain in streptozotocin-induced diabetic peripheral neuropathy, including mechanical allodynia (MA), cold allodynia (CA), mechanical hyperalgesia (MH) and thermal hyperalgesia (TH) [3]. In most experiments, only one type of pain (i.e. MA, TH and MH) is observed in the streptozotocin-induced diabetic peripheral neuropathy model on a limited basis [4-8, 9, 10]. In other case, two evident pain modalities (i.e., MA-TH, MH-CA and TH-CA) are used to study mechanisms of diabetic peripheral neuropathy and to evaluate potential therapies [11-14]. We assumed that limited experimental design may be originated from the low ratio of pain induction according to experimental conditions. Thus, the present study was aimed to address the induction of pain modalities in streptozotocin-induced diabetic peripheral neuropathy as compared with that of zymosan induced inflammatory pain model.
Until now, most studies aimed at understanding how pain amplification occurs in the spinal cord have focused exclusively on neurons. Accumulating data suggest that spinal glial activation can contribute to enhanced nociceptive responses in a state of clinical pain [15]. Therefore, we further evaluated a change of glia expression (i.e., microglia and astrocyte) in the spinal cord at in streptozotocin-induced diabetic peripheral neuropathy, as compared with the zymosan model.
Male Sprague-Dawley rats (7 weeks, 200-220 g, Dae Han Biolink Co., Eumsung, Korea) were housed in colony cages with free access to food and water and maintained in temperature and light controlled rooms (23±2℃, 12/12 hours light/dark cycle with lights on at 08:00) at least one week before experiment. All of the methods used in the present study were approved by the Institute of Animal Care and Use Committee at Chonbuk National University and conform to NIH guidelines (NIH publication No. 86-23, revised in 1985).
Experimental animals were briefly anesthetized with 3% isoflurane in a mixed N2O/O2 gas. Experimental diabetes was induced in rats (n=28) by a single injection of streptozotocin solution (50 mg/kg, i.v.) into the tail vein. Streptozotocin was prepared in saline (0.9% NaCl adjusted to pH 4.0 with hydrochloric acid) on ice; the solution was discarded if bubbling was noted. Control rats (n=5) for behavioral experiments received an equal volume of saline. We began checking blood sugar levels 48 hours after injections using a blood glucometer (Accu-check Active; Roche Diagnostics, Indiana, USA). Rats with values of >400 mg/dL were considered hyperglycemic and were included in the experimental group.
Inflammation was induced in rat (n=8) by a single subcutaneous injection (200 µL) into the plantar surface of the right hind paw of zymosan (Sigma, Missouri, USA) a from yeast cell wall suspended in sterile water (30 mg/mL concentration). Control rat (n=5) were similarly injected with sterile vehicle.
Measurement of pain behavioral tests was performed blindly either at 5 after zymosan injection or at 3 weeks after streptozotocin injection. Animals were acclimatized to the testing chambers for 1 hour at 1 day before and the day of behavioral testing. Each test was duplicated at least 5 min intervals.
To assess nociceptive responsiveness to hind paw application of mechanical stimuli, rats were placed in elevated individual clear Plexiglas observation wire mesh floor of the chamber (diameter 25 cm). Through the, a series of eight von Frey hair monofilaments (Stöelting, Illinois, USA), calibrated to produce incremental forces of 0.4, 0.6, 1, 2, 4, 8, 15 and 20 g, were applied to the middle of the middle and fourth toe of the hind paw for a maximum of 3 s, or until the animal displayed a nociceptive response comprised of paw lifting and/or shaking. Testing was initiated with the 2 g filament. The data collected using this up-down method [16] was then used to calculate the 50% mechanical paw withdrawal threshold (g). The mechanical threshold in normal animal was above 20 g. Thus, pain severity was divided into four groups: severe (<5 g), moderate (5-8 g), mild (8-15 g) and non-pain induction (>15 g).
Cold stimulation of the hind paw was carried out. A jet of 100 mL of acetone was applied to the middle of the plantar surface of the hind paw, with the aid of an insulin syringe and from a short distance (5 mm), through the wire mesh floor of the observation chamber. The frequency of shakes and/or licking of the hind paw, due to the cooling evoked by acetone's evaporation, were observed and this was used as an index of the nociceptive responsiveness to cold stimulation. The withdrawal frequency by acetone in normal animal was below 5 times. Thus, pain severity was divided into four groups: severe (>16 times), moderate (10-15 times), mild (5-10 times) and non-pain induction (<5 times).
Using thermal plantar tester (Ugo Basile, Commerio, Italy), radiant heat was applied to the plantar surface of the hindpaw until the rat lifted its paw. A photoelectric cell automatically turned the heat source off when the reflected light beam was interrupted (i.e., when the animal withdrew its paw) and the time at which this occurred was recorded as the paw withdrawal latency. Heating was terminated at a 20 second cutoff to prevent tissue damage if an animal failed to withdraw its paw prior to the cutoff. The thermal threshold in normal animal was above 20 second. Thus, pain severity was divided into four groups: severe (<8 sec), moderate (8-16 sec), mild (16-20 sec) and non-pain induction (>20 sec).
The nociceptive flexion reflex was quantified with an Analgesymeter (Ugo Basile, Commerio, Italy). This device generates a mechanical force that increases linearly with time. The force is applied to the dorsum of the rat's hindpaw, by a wedge-shape plunger. The nociceptive threshold is defined as the force (g) at which the rat withdraws its paw. The mechanical threshold in normal animal was above 200 g. Thus, pain severity was divided into four groups: severe (<90 g), moderate (90-130 g), mild (130-200 g) and non-pain induction (>200 g).
Finishing behavioral observation, rats were deeply anesthetized with 5% isoflurane and perfused transcardially with calcium-free Tyrode's solution followed by fixative containing 4% paraformaldehyde and 0.2% picric acid in 0.1 M phosphate buffered saline (PBS, pH 6.9). L4 spinal cord was removed immediately after perfusion, postfixed in the same fixative for 4 hours and then cryoprotected in PBS containing 30% sucrose (pH 7.4).
A series of frozen sections (40 µm) was cut using a cryostat (Microm, Walldorf, Germany). Spinal cord section was performed by prefloating protocol and thaw mounting protocol, respectively. Preblocking with 5% normal rabbit serum in 5% BSA and 0.3% triton X-100 in PBS, the sections were incubated in mouse anti-CD11b (Serotec, Oxford, UK, 1:10,000) or mouse anti-glial fibrillary acidic protein (GFAP, MerkMillipore, Massachusetts, USA, 1:10,000) at 4℃ overnight. The sections were subsequently incubated in cy3-conjugated secondary antibody (Jackson Immunoresearch, Pennsylvania, USA, 1:200) for 2 hour at room temperature and coverslipped.
Spinal tissues were scanned with ECLIPSE 80i (Nikon, Japan) fluorescent microscope, and images of individual sections were digitized with 4096 grayscale levels using a cooled CCD camera (CoolSnap ES model, Nihon Roper, Tokyo, Japan) connected to a computer-assisted image analysis system (Metamorph, Universal Imaging Co., Pennsylvania, USA).
Following intravenous injection of streptozotocin, the concentration of blood glucose was markedly increased on day 3 (403.5±10.5 mg/dL) and remained stable through the 3 week follow-up period. In contrast, saline-injected animals maintained normal blood glucose levels (95.1±3.4 mg/dL) that did not differ from baseline levels (data not shown).
Evaluation of pain behavior by either thermal or mechanical stimuli was performed at 3 weeks or 5 hours after either streptozotocin or zymosan injection. The control group for streptozotocin and zymosan did not evoke any behaviors of pain avoidance (data not shown). The pain severity, according to pain modality after streptozotocin or zymosan injection, was divided into 4 groups: severe, moderate, mild, and non-pain induction, as described in the material and method section.
We found that the allodynia pattern between streptozotocin and zymosan was significantly different. At the MA test, zymosan injection evoked severe pain (100%) exclusively, whereas streptozotocin induced weak degree of pain (severe: 7.1%, moderate: 50.0%, mild: 28.6%, non-pain: 14.3%) (Figure 1A). Although zymosan-injection produced weak severity of the CA (mild: 25%, non-pain: 75%), streptozotocin evoked stronger pain behavior, as compared with zymosan (severe: 7.1%, moderate: 42.9%, mild: 35.7%, non-pain: 14.3%) (Figure 1B).
On the other hand, the induction rate of MH and TH was similar between streptozotocin and zymosan. Moreover, all animals show MH and TH, without exception, as compared with that of allodynia. Streptozotocin evoked TH (severe: 65.0%, moderate: 25.0%, mild: 10%) (Figure 2A). In addition, zymosan evoked the same pattern of TH (severe: 50.0%, moderate: 50.0%) (Figure 2A). Interestingly, it was notable that the induction rate of MH (severe type) by streptozotocin was higher than that of zymosan (35.7% vs 12.5%, respectively) (Figure 2B).
Using immunostaining for CD11b or GFAP, we show a pattern of microglia or astrocyte proliferation after streptozotocin or zymosan inflammation. Microglial activation was predominant in superficial dorsal horn, as well as lamina III-IV, after streptozotocin (Figure 3C) or zymosan (Figure 3B) injection compared with the control rat (Figure 3A). Although astrocyte proliferation was not significant in the zymosan injection group (Figure 3E) as compared with the control group (Figure 3D), streptozotocin dramatically increased astrocyte immunoreactivity (Figure 3F).
In this study, we demonstrated that streptozotocin induced diabetic peripheral neuropathy produced several types of pain symptoms that included two types of allodynia (MA and CA) and two types of hyperalgesia (TH and MH); there were similar with that of zymosan-induced inflammation. The pain modality, based on pain severity, was divided into 4 groups: severe, moderate, mild, and non-pain induction, the induction ratio was significantly different from that of the zymosan-induced inflammation. To evaluate underlying pain mechanisms on a preclinical basis, the pain severity should be located at least at the moderate level. From this consideration, pain induction ratio of TH (90.0%) and MH (85.7%) was higher than that of MA (57.1%) and CA (50.0%) after streptozotocin injection. On the other hand, zymosan-induced inflammation produced high induction ratio of MA (100%), TH (100%) and MH (75.0%), whereas CA (0%) was not evident.
In the streptozotocin-induced diabetic peripheral neuropathy study, some experiments are unfortunately performed to evaluate the drug effect relating to specific mechanisms using only the MA test [4-6]. From our findings, only half (57.1%) of the streptozotocin injected animals show MA. Therefore, it is possible that a limited effect against MA introduces some misunderstanding relating to overall therapeutic effect for diabetic peripheral neuropathy phenomenon. When it is evaluated by only one pain modality, such as MH [7,8] or TH [9,10], it may result in the mistake of hasty generalization. Although the use of 2 types of pain modalities, such as CA-TH [11], MA-TH [12,13,17], MH-CA [14] is more desirable rather than use of one modality, our finding indicated that a recommendable parameter is MH-TH, as performed in a laboratory [7].
Recent studies emphasize the role of non-neuronal cells, particularly microglia in the development of neuronal hypersensitivity. diabetic peripheral neuropathy is characterized by microglial activation that is unwanted and considered to contribute to or to even cause MA, TH and spontaneous pain [15]. In support, the present study observed that spinal microglia was significantly increased by streptozotocin injection. A recent study further revealed that activated microglia may be involved in the development of diabetic peripheral neuropathy (CA and TH) and minocycline (a selective inhibitor of microglial activation) exerts its effect by inhibition of the neuroimmune activation of microglia [11]. In addition, minocyline treatment reduces the levels of interleukin-1β (IL-1β) and tumor necrosis factor-alpha, lipid peroxidation, nitrite, and improved antioxidant defense in spinal cords of type 1 diabetic rats [11]. Using a db/db type 2 diabetes mouse model that displays obvious MA, spinal astrocytes are dramatically activated and "Astrocyte/IL-1β/NMDA receptor-Neuron" pathway may be the detailed mechanism of astrocyte-induced allodynia [18]; activation does not occur in microglia. In addition, we found that the streptozotocin-induced type 1 diabetic rat has increased astrocyte activation. Together with altered microglia and astrocyte expressions, this may contribute to painful episodes in diabetic peripheral neuropathy from type 1 diabetes.
In conclusion, the present study recommends an optimal pain parameter (MH-TH) for evaluating the underlying mechanism of streptozotocin-induced diabetic peripheral neuropathy in the rat. Moreover, it is proper to include a study of glia study, in addition to neuronal activity, to understand the overall mechanism underlying diabetic peripheral neuropathy.
Figures and Tables
Figure 1
The severity of mechanical allodynia (MA, A) or cold allodynia (CA, B) after streptozotocin or zymosan injection. The severity of MA was divided into 4 groups: severe (<5 g), moderate (5-8 g), mild (8-15 g) and non-pain induction (non, >15 g). The severity of CA was divided into four groups: severe (>16 times), moderate (10-15 times), mild (5-10 times) and non-pain induction (non, <5 times).
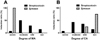
Figure 2
The severity of thermal hyperalgesia (TH, A) or mechanical hyperalgesia (MH, B) after streptozotocin or zymosan injection. The severity of TH was divided into 4 groups: severe (<8 sec), moderate (8-16 sec), mild (16-20 sec) and non-pain induction (non, >20 sec). The severity of CA was divided into four groups: severe (<90 g), moderate (90-130 g), mild (130-200 g) and non-pain induction (non, >200 g).
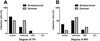
Acknowledgments
This research was supported by Basic Science Research Program through the National Research Foundation of Korea (NRF) funded by the Ministry of Education, Science and Technology (2009-0069754).
References
1. Coppey LJ, Davidson EP, Dunlap JA, Lund DD, Yorek MA. Slowing of motor nerve conduction velocity in streptozotocin-induced diabetic rats is preceded by impaired vasodilation in arterioles that overlie the sciatic nerve. Int J Exp Diabetes Res. 2000. 1(2):131–143.
2. Obrosova IG. Diabetic painful and insensate neuropathy: pathogenesis and potential treatments. Neurotherapeutics. 2009. 6(4):638–647.
3. Morrow TJ. Animal models of painful diabetic neuropathy: the STZ rat model. Curr Protoc Neurosci. 2004. Chapter 9:Unit 9. 18.
4. Chen SR, Samoriski G, Pan HL. Antinociceptive effects of chronic administration of uncompetitive NMDA receptor antagonists in a rat model of diabetic neuropathic pain. Neuropharmacology. 2009. 57(2):121–126.
5. Mixcoatl-Zecuatl T, Jolivalt CG. A spinal mechanism of action for duloxetine in a rat model of painful diabetic neuropathy. Br J Pharmacol. 2011. 164(1):159–169.
6. Xu GY, Li G, Liu N, Huang LY. Mechanisms underlying purinergic P2X3 receptor-mediated mechanical allodynia induced in diabetic rats. Mol Pain. 2011. 7:60.
7. Fuchs D, Birklein F, Reeh PW, Sauer SK. Sensitized peripheral nociception in experimental diabetes of the rat. Pain. 2010. 151(2):496–505.
8. Nirogi R, Jabaris SL, Jayarajan P, Abraham R, Shanmuganathan D, Rasheed MA, Royapalley PK, Goura V. Antinociceptive activity of α4β2* neuronal nicotinic receptor agonist A-366833 in experimental models of neuropathic and inflammatory pain. Eur J Pharmacol. 2011. 668(1-2):155–162.
9. Manni L, Florenzano F, Aloe L. Electroacupuncture counteracts the development of thermal hyperalgesia and the alteration of nerve growth factor and sensory neuromodulators induced by streptozotocin in adult rats. Diabetologia. 2011. 54(7):1900–1908.
10. Talbot S, Chahmi E, Dias JP, Couture R. Key role for spinal dorsal horn microglial kinin B1 receptor in early diabetic pain neuropathy. J Neuroinflammation. 2010. 7(1):36.
11. Pabreja K, Dua K, Sharma S, Padi SS, Kulkarni SK. Minocycline attenuates the development of diabetic neuropathic pain: possible anti-inflammatory and anti-oxidant mechanisms. Eur J Pharmacol. 2011. 661(1-3):15–21.
12. Bianchi R, Cervellini I, Porretta-Serapiglia C, Oggioni N, Burkey B, Ghezzi P, Cavaletti G, Lauria G. Beneficial effects of PKF275-055, a novel, selective, orally bioavailable, long-acting dipeptidyl peptidase IV inhibitor in streptozotocin-induced diabetic peripheral neuropathy. J Pharmacol Exp Ther. 2012. 340(1):64–72.
13. Messinger RB, Naik AK, Jagodic MM, Nelson MT, Lee WY, Choe WJ, Orestes P, Latham JR, Todorovic SM, Jevtovic-Todorovic V. In vivo silencing of the Ca(V)3.2 T-type calcium channels in sensory neurons alleviates hyperalgesia in rats with streptozocin-induced diabetic neuropathy. Pain. 2009. 145(1-2):184–195.
14. Naruse K, Sato J, Funakubo M, Hata M, Nakamura N, Kobayashi Y, Kamiya H, Shibata T, Kondo M, Himeno T, Matsubara T, Oiso Y, Nakamura J. Transplantation of bone marrow-derived mononuclear cells improves mechanical hyperalgesia, cold allodynia and nerve function in diabetic neuropathy. PLoS One. 2011. 6(11):e27458.
15. Graeber MB, Christie MJ. Multiple mechanisms of microglia: a gatekeeper's contribution to pain states. Exp Neurol. 2012. 234(2):255–261.
16. Dixon WJ. Efficient analysis of experimental observations. Annu Rev Pharmacol Toxicol. 1980. 20:441–462.
17. Hwang HS, Yang EJ, Lee SM, Lee SC, Choi SM. Antiallodynic Effects of Electroacupuncture Combined with MK-801 Treatment through the Regulation of p35/p25 in Experimental Diabetic Neuropathy. Exp Neurobiol. 2011. 20(3):144–152.
18. Liao YH, Zhang GH, Jia D, Wang P, Qian NS, He F, Zeng XT, He Y, Yang YL, Cao DY, Zhang Y, Wang DS, Tao KS, Gao CJ, Dou KF. Spinal astrocytic activation contributes to mechanical allodynia in a mouse model of type 2 diabetes. Brain Res. 2011. 1368:324–335.