Abstract
Since endocrine disrupting chemicals (EDCs) may interfere with the endocrine system(s) of our body and have an estrogenicity, we evaluated the effect(s) of bisphenol A (BPA) on the transcriptional levels of altered genes in estrogen receptor (ER)-positive BG-1 ovarian cancer cells by microarray and real-time polymerase-chain reaction. In this study, treatment with 17β-estradiol (E2) or BPA increased mRNA levels of E2-responsive genes related to apoptosis, cancer and cell cycle, signal transduction and nucleic acid binding etc. In parallel with their microarray data, the mRNA levels of some altered genes including RAB31_MEMBER RAS ONCOGENE FAMILY (U59877), CYCLIN D1 (X59798), CYCLIN-DEPENDENT KINASE 4 (U37022), IGF-BINDING PROTEIN 4 (U20982), and ANTI-MULLERIAN HORMONE (NM_000479) were significantly induced by E2 or BPA in this cell model. These results indicate that BPA in parallel with E2 induced the transcriptional levels of E2-responsive genes in an estrogen receptor (ER)-positive BG-1 cells. In conclusion, these microarray and real-time polymerase-chain reaction results indicate that BPA, a potential weak estrogen, may have estrogenic effect by regulating E2-responsive genes in ER-positive BG-1 cells and BG-1 cells would be the best in vitro model to detect these estrogenic EDCs.
Endocrine disrupting chemicals (EDCs) are environmentally persistent chemicals that interfere with physiological endocrine systems, adversely affecting hormone balance or disrupting normal function in the organs that hormones regulate or modulate [1,2]. Thus, EDCs can lead to serious detrimental effects on reproductive and developmental processes of humans, animals, and plants. Consequently, endocrine disruption is a major health priority worldwide, particularly for women and children [3,4]. EDCs include both natural and man-made sources of estrogenic or androgenic chemicals that have structures similar to estrogen or androgen and that bind to estrogen receptor (ER) or androgen receptor (AR) to interfere with the actions of endogenous steroid hormones [3-6]. One class of EDCs is estrogenic phenol compounds, octyl-phenol (OP), nonyl-phenol (NP), and bisphenol A (BPA), which have chemical structure similar or distinct compared to that of 17β-estradiol (E2) [5]. Some of these phenols are breakdown products of surfactants, found in soaps and detergents. Further, there is increasing evidence that EDCs may interfere with the body's complex and carefully regulate hormonal system in diverse ways [4,7].
BPA, a compound used in polycarbonate and epoxy resins, has been implicated as a potent risk factor for women's health [8]. BPA has been widely used in plastic products such as drinking water containers, dental sealants, and coating materials to prevent corrosion on the inner surface of canned food. But BPA is released from plastic containers under normal conditions of use and its leaching is facilitated at high temperature [9]. Exposure to BPA is even possible in contact with swallowed dust [10] and exerts xenoestrogen action [11]. The mechanism by which EDCs interfere with endogenous steroid hormones is not well understood. As many EDCs are thought to affect sex hormone function, and therefore reproduction, the findings in multi-generation animal studies can provide strong evidence of the potential for endocrine disruption [12]. In vitro methods for detection of estrogenic compounds have been developed by several groups [13-15]. The recent findings indicate that BPA, a weak estrogenic EDC, activates the transcription factor, cAMP-responsive element binding protein (CREB), via an alternative mechanism involved in a non-classical membrane ER [16]. In addition, useful biomarkers have been suggested to include the cell-based endogenous genes [17,18]. Our previous research utilized immature rats to detect estrogenic compounds [5,6,19].
A better understanding of the cellular mechanisms underlying the effect of EDCs is absolutely required in novel ovarian cellular models expressing ERs. Based on our previous studies, we further examined the altered gene expressions following treatment with E2 or BPA by microarray and real-time polymerase-chain reaction (PCR). This study would provide the basis for further research into determining E2-or BPA-induced gene expressions in highly E2-responsive ovarian cancer cells.
An ovarian adenocarcinoma cell line, BG-1, is well known for the expression of ERα [20]. This cell line was obtained from Dr. K.S. Korach (National Institute of Environmental Health Sciences, Research Triangle Park, NC, USA). It was cultured in Dulbecco's modified Eagle's medium (DMEM)/F12 mixture (Sigma-Aldrich, St. Louis, MO, USA) supplemented with 10% fetal bovine serum (FBS; Hyclone Laboratories, Logan, UT, USA), 100 U/mL penicillin G and 100 µg/mL streptomycin (Life Technologies, Rockville, MD, USA) at 37℃ in a humidified atmosphere of 5% CO2-95% air.
After 70% confluence, BG-1 cells were treated with a concentration of E2 (10-7 M; Sigma-Aldrich) or BPA (10-5 M; Sigma-Aldrich) at different time points (1, 8 and 24 h) before harvest. Ethanol was added to the control media in the same final solvent concentration (typically 0.1%).
Western blot assay was performed to identify the expression of ERα and ERβ in BG-1 cells. Thirty µg of total protein was run on a 10% sodium dodecyl sulfate-polyacrylamide gel electrophoresis (SDS-PAGE) and transferred to nitrocellulose membrane. The membrane was Western-blotted using a mouse monoclonal antibody for ERα (Santa Cruz Biotechnology, Santa Cruz, CA, USA) and ERβ (Abcam, Cambridge, MA, USA). Briefly, 1×106 cells were washed twice with ice-cold phosphate-buffered saline (PBS) and lysed in 100 µL of in ice-cold RIPA buffer [containing 1× PBS (pH 7.4), 1% NP-40, 0.5% sodium deoxycholate, 0.1% SDS, 10 mg/mL phenylmethylsulfonyl fluoride, 30 mg/mL aprotinin, and 10 mg/mL leupeptin] for 15 min on ice. The protein concentration was determined using Bradford assay (Bio-Rad Laboratories, Hercules, CA, USA). The protein solution was then subjected to electrophoresis on a 10% SDS-PAGE and electrotransferred to a nitrocellulose membrane (Hybond C; Amersham Pharmacia, Oakville, ON, Canada). After washing three times with tri-buffered saline-Tween (TBS-T; 0.1% Tween-20 in TBS) for 15 min, the signals were detected with horseradish peroxidase-conjugated secondary antibody (Amersham Pharmacia) and visualized using the ECL chemiluminescent system (Amersham Pharmacia).
Total RNA was extracted with TriZol (Invitrogen Life Technologies, Carlsbad, CA, USA) according to manufacturer's suggested procedure, and purified using RNeasy total RNA isolation kit (Qiagen, Valencia, CA, USA) according to the manufacturer's instructions. DNA was digested using an RNase-free DNase set (Qiagen) during RNA purification. Total RNA was quantified by spectrophotometer and its integrity was assessed by running on a 0.8% agarose gel contained diethyl pyrocarbonate (DEPC). To make cDNA from total RNA for microarray analysis, the same quantity of each RNA sample from the treated groups (n=4) or control groups (n=3) was pooled. A cDNA microarray consists of approximate 8,000 cDNA spot including Incyte clones, housekeeping genes and Arabidopsis thaliana (A. thaliana) DNA as controls. The PCR was prepared according to the standard protocol and reaction mixtures were subjected to be amplified at 35 cycles. The primer pair used for amplification was overlap primer-1 (5'-AAT TAA CCC TCA CTA AAG GG-3') and overlap primer-2 (5'-GTA ATA CGA CTC ACT ATA GGG C-3'). The size and amount of the PCR products were verified on 1% agarose gel. The PCR products were purified by ethanol precipitation, then resuspended in 15 µL of hybridization solution (GenoCheck, Sangrokgu, Korea), and spotted onto CMT-GAPS II silane slide glass (Corning Life Sciences, Corning, NY, USA) with a pixsys 5500 arrayer (Cartesian Technologies Inc., Irvine, CA, USA) using 16-Stealth Micro spotting pins. The printed slides were processed according to CMT-GAPS II silane slide protocol. Briefly, the spots were re-hydrated with 1× SSC for 1 min and then DNA linked using a UV crosslinker (Stratagene Corp., La Jolla, CA, USA). The slides were soaked in the succinic anhydride/sodium borate solution for 15 min with gentle agitation and then transferred to a 95℃ water bath for 2 min. The slides were quickly transferred to 95% ethanol for 1 min and then dried using a centrifuge at 3,000 rpm for 20 sec.
Eighty mL of probe mixture was heated at 95℃ for 3 min, and kept at 65℃ until use. Prior to hybridization, array slide was incubated with prehybridization buffer containing 5× SSC, 0.1% SDS and 1% bovine serum albumin (BSA) at 48℃ for 45 min. The slide was washed by dipping in deionized water for 30 sec and dipped in isopropanol for 10 sec and spun dried in a centrifuge for 2 min. To hybridize probe with array slide, probe was added to the denatured array slide and cover slip was put on this slide. This slide was transferred to hybridization chamber and incubated overnight at 42℃. The slide was washed twice with wash buffer containing 1× SSC and 0.2% SDS at 42℃ and washed two times with 1× SSC at room temperature. Slide was then spun dried for 5 min. This microarray was scanned as described by the scanner's manufacturer. Microarray results were categorized into several functional groups related with apoptosis, cancer, cell cycle, growth factor/hormone, and signal transduction.
Hybridized slides were scanned with the Axon Instruments GenePix 4000B scanner and the scanned images were analyzed with the software program GenePix Pro 5.1 (Axon Instruments, Foster, CA, USA) and GeneSpring 7.1 (Sillicon Genetics, Redwood, CA, USA). In order to allow algorithm to eliminate all bad spots, no data points were eliminated by visual inspection from the initial GenePix image. For signal normalization, housekeeping genes (β-actin) and positive control genes (A. thaliana genes) were spotted onto each slide. The signals of these spots were used for normalization. To filter out the unreliable data, spots with signal-to-noise (signal-background-background SD) below 100 were not included in the data. Data were normalized by global, lowess, print-tip and scaled normalization for data reliability. Data were sorted of above 2-fold altered genes using GeneSpring 7.1 (Sillicon Genetics, Redwood, CA, USA) and a hierarchical clustering analysis was performed using Pearson correlation. The statistical significance of differential expression was assessed by computing a q-value for each gene. To determine the q-value, we used a permutation procedure, and for each permutation a two-sample t-statistic was computed for each gene. The result was considered significant when the logarithmic gene expression ratio of three independent hybridizations was more than twofold the difference in the expression level. The accuracy of microarray analysis in this study was confirmed by real-time PCR.
The standard curve was generated for a standard RNA preparation by serial dilution (1, 1/10, 1/100, 1/1000, and 0). A real-time PCR reaction was carried out in a 25 µL final volume containing 12.5 µL of 2× premix (TaKaRa Bio, Otsu, Japan), 0.3 µL of each of forward and reverse primers, 1 µL of cDNA, and distilled water up to 25 µL. The oligonucleotide sequences of primers were employed to detect various genes as shown Table 1. PCR using the Smart Cycle System (TaKaRa Bio) began with an initial denaturation at 95℃ for 30 sec. Each of the 35 amplification cycles consisted of denaturation at 95℃ for 5 sec, annealing at 55℃ for 15 sec, and extension at 72℃ for 15 sec. Relative expression levels of each sample were calculated based on the cycle threshold (Ct) and monitored for an amplification curve. The PCR amplification curves were evaluated by fluorescence of the double-stranded DNA-specific dye, SYBR Green, versus the amount of standardized PCR product. All gene expressions were normalized to the expression of β-actin mRNA as a control.
We confirmed the expression of ERα and ERβ by immunoblot analysis using ERα and ERβ specific antibodies in ovarian cancer cells (BG-1). Both ERα and ERβ proteins were expressed in BG-1 cells and detected in 68 kDa and 55 kDa, respectively (Figure 1).
Following individual treatment of BG-1 cells with E2 or BPA, total RNAs from the cells were isolated and processed by microarray analysis as described. Although the number of genes whose expression was altered by E2 or BPA tested is modest, there is substantial change in the gene expression altered or modified by their exposure time. Increased expression levels were shown in red and decreased expression levels shown green (Figure 2). The intensities of each spot in this study were calculated and plotted. A clustering analysis of typical of the three self vs. self comparisons was performed in Figure 2, which showed that a more than two-fold change would be necessary to overcome the intrinsic variability introduced by the hybridization procedure. For this reason, the data was further filtered to select for genes that showed an absolute differential of at least two-fold or greater. The change in the mRNA expressions of 51 genes was listed in Table 2 and Table 3. Table 2 showed the common 33 genes related apoptosis, cancer and cell cycle induced by E2 or BPA treatment at different time points (1, 8, and 24 h). In addition, altered gene expressions involved in signal transduction and nucleic acid binding were listed following treatment with E2 or BPA in Table 3. These genes might be universal estrogen-responsive genes among various tissues. This compound exhibited a reasonable hierarchical clustering; BPA resembled to E2.
The expression patterns of several genes up-regulated by E2 or BPA were confirmed to verify the results of the microarray analysis using real-time PCR analysis. The expression levels of altered genes including RAB31_MEMBER RAS ONCOGENE FAMILY (U59877), CYCLIN D1 (X59798), CYCLIN-DEPEND ENT KINASE 4 (U37022), INSULIN-LIKE GROWTH FACTOR-BINDING PROTEIN 4 (U20982), and ANTI-MULLERIAN HORMONE (NM_000479) were measured by microarray analysis following treatment with E2 or BPA at 24 h in BG-1 cells. In parallel with their microarray results, the mRNA levels of these genes were significantly induced by E2 or BPA at 24 h as shown in Figure 3. Among them, the most increased gene was RAB31_MEMBER RAS ONCOGENE FAMILY (U59877) as demonstrated. These results indicate that BPA in parallel with E2 induced the transcriptional levels of E2-responsive genes in an ER-positive BG-1 cells.
EDCs are environmental chemicals that interfere with physiological systems, adversely affecting hormone balance (endocrine systems) or disrupting normal function in the organs that hormones regulate or modulate [3,21-25]. Through diverse mechanisms, EDCs can lead to serious reproductive, developmental, and metabolic dysfunction in humans as well as a wide range of animal and plant species [21,26-28]. Of particular relevance to mammalian biology are EDCs that are structurally similar to estrogen, and can therefore bind to the ERs and interfere with the actions of the endogenous steroid hormone [3]. Many EDCs are believed to have potential estrogenicity and androgenicity. For example, recent studies demonstrate that exposure of gestating female rats to vinclozolin (an antiandrogenic compound) or methoxychlor (an estrogenic compound) decreased spermatogenic capacity of pups, which resulted in increased incidence of male infertility and an epigenetic transgenerational phenotype involving a number of diseases [26,29]. In our previous studies, several phenol products, OP, NP and BPA, have an estrogenic or progestogenic effect on immature rats or mice to induce calbindin-D9k (CaBP-9k), a cytoplasmic calcium binding protein [5,30-32]. We demonstrated that the absorption and distribution of environmental estrogenic compounds in maternal and neonatal uteri are extremely rapid, and these chemicals can easily pass through the placenta during pregnancy or breast milk during lactation to affect functions of neonatal or fetal reproductive tissues [33-36]. In addition, genistein, an ERβ ligand, enhanced CaBP-9k gene via ERα in the uterus of immature rats, suggesting that ERα may be a key mediator in uterine CaBP-9k gene induction in immature rats [37].
Despite the prevalence of environmental EDCs, there are few in vitro and in vivo methods for determining whether or not a chemical is an EDC. These methods include the receptor binding assay, reporter gene assay, and immature rat uterotrophic assay. The reporter gene assay has many benefits as a promising prescreening procedure, because this assay could be performed as a rapid screening process to detect an EDC mixed with thousands of other chemicals [38]. Genetically-sensitive animal models, based on mice and rats, could screen estrogenic chemicals for the induction of endocrine-related genes. Efforts to identify the mechanisms of endocrine disruption by estrogenic chemicals need to be supported; these might find optimal tests for thousands of chemicals potentially active in reproductive development and function [39]. In our previous study, the EDC-induced proliferation is mediated by a genomic effect through ERs and estrogen response element (ERE), but EDC-activated mitogen-activated protein kinase (MAPK) is unlikely involved in the EDC-induced cell growth in estrogen-responsive ovarian cancer cells [40]. In this study, we employed BG-1 cells (ovarian cancer) model expressing ERs to detect the effect E2 or BPA by using microarray and real-time PCR analysis. The genes related to apoptosis, cancer, cell cycle, signal transduction and nucleic acid binding etc. were induced by E2 or BPA treatment at different time points. These genes might be universal estrogen-responsive genes among various tissues. This compound exhibited a reasonable hierarchical clustering; BPA resembled to E2.
To confirm the results of microarray analysis, we selected several altered genes among them and further measured the transcriptional levels of 5 altered genes including RAB31_MEMBER RAS ONCOGENE FAMILY (U59877), CYCLIN D1 (X59798), CYCLIN-DEPENDENT KINASE 4 (U37022), INSULIN-LIKE GROWTH FACTOR-BINDING PROTEIN 4 (U20982), and ANTI-MALLERIAN HORMONE (NM_00479) by real-time PCR following treatment with E2 (10-7 M) or BPA (10-5 M) at 24 h in this cell model. In parallel with their microarray results, the mRNA levels of these genes were significantly induced by E2 or BPA treatment as shown in Figure 3. These results indicate that BPA in parallel with E2 induced the transcriptional levels of E2-responsive genes in an ER-positive BG-1 cells.
In conclusion, these microarray and real-time PCR results indicate that BPA, a potential weak estrogen, may have estrogenic effect by regulating E2-responsive genes in ER positive BG-1 cells and BG-1 cells would be the best in vitro model to detect these estrogenic EDCs.
Acknowledgments
This work was supported by the research grant of the Chungbuk National University in 2010.
References
1. Choi KC, Jeung EB. The biomarker and endocrine disruptors in mammals. J Reprod Dev. 2003; 49(5):337–345. PMID: 14967909.


2. Dang VH, Choi KC, Jeung EB. Tetrabromodiphenyl ether (BDE 47) evokes estrogenicity and calbindin-D9k expression through an estrogen receptor-mediated pathway in the uterus of immature rats. Toxicol Sci. 2007; 97(2):504–511. PMID: 17361017.


3. Daston GP, Cook JC, Kavlock RJ. Uncertainties for endocrine disrupters: our view on progress. Toxicol Sci. 2003; 74(2):245–252. PMID: 12730617.


4. Borgert CJ, LaKind JS, Witorsch RJ. A critical review of methods for comparing estrogenic activity of endogenous and exogenous chemicals in human milk and infant formula. Environ Health Perspect. 2003; 111(8):1020–1036. PMID: 12826475.


5. An BS, Choi KC, Kang SK, Hwang WS, Jeung EB. Novel Calbindin-D(9k) protein as a useful biomarker for environmental estrogenic compounds in the uterus of immature rats. Reprod Toxicol. 2003; 17(3):311–319. PMID: 12759100.


6. An BS, Kang SK, Shin JH, Jeung EB. Stimulation of calbindin-D(9k) mRNA expression in the rat uterus by octyl-phenol, nonylphenol and bisphenol. Mol Cell Endocrinol. 2002; 191(2):177–186. PMID: 12062901.


7. Crisp TM, Clegg ED, Cooper RL, Wood WP, Anderson DG, Baetcke KP, Hoffmann JL, Morrow MS, Rodier DJ, Schaeffer JE, Touart LW, Zeeman MG, Patel YM. Environmental endocrine disruption: an effects assessment and analysis. Environ Health Perspect. 1998; 106(Suppl 1):11–56. PMID: 9539004.


8. Wozniak AL, Bulayeva NN, Watson CS. Xenoestrogens at picomolar to nanomolar concentrations trigger membrane estrogen receptor-alpha-mediated Ca2+ fluxes and prolactin release in GH3/B6 pituitary tumor cells. Environ Health Perspect. 2005; 113(4):431–439. PMID: 15811834.
9. Markey CM, Rubin BS, Soto AM, Sonnenschein C. Endocrine disruptors: from Wingspread to environmental developmental biology. J Steroid Biochem Mol Biol. 2002; 83(1-5):235–244. PMID: 12650721.


10. Staples CA, Dorn PB, Klecka GM, O'Block ST, Branson DR, Harris LR. Bisphenol A concentrations in receiving waters near US manufacturing and processing facilities. Chemosphere. 2000; 40(5):521–525. PMID: 10665389.


11. Steinmetz R, Mitchner NA, Grant A, Allen DL, Bigsby RM, Ben-Jonathan N. The xenoestrogen bisphenol A induces growth, differentiation, and c-fos gene expression in the female reproductive tract. Endocrinology. 1998; 139(6):2741–2747. PMID: 9607780.


12. Cupp AS, Skinner MK. Actions of the endocrine disruptor methoxychlor and its estrogenic metabolite on in vitro embryonic rat seminiferous cord formation and perinatal testis growth. Reprod Toxicol. 2001; 15(3):317–326. PMID: 11390175.


13. Jorgensen M, Vendelbo B, Skakkebaek NE, Leffers H. Assaying estrogenicity by quantitating the expression levels of endogenous estrogen-regulated genes. Environ Health Perspect. 2000; 108(5):403–412. PMID: 10811566.


14. Miller S, Kennedy D, Thomson J, Han F, Smith R, Ing N, Piedrahita J, Busbee D. A rapid and sensitive reporter gene that uses green fluorescent protein expression to detect chemicals with estrogenic activity. Toxicol Sci. 2000; 55(1):69–77. PMID: 10788561.


15. Shelby MD, Newbold RR, Tully DB, Chae K, Davis VL. Assessing environmental chemicals for estrogenicity using a combination of in vitro and in vivo assays. Environ Health Perspect. 1996; 104(12):1296–1300. PMID: 9118870.


16. Quesada I, Fuentes E, Viso-Leon MC, Soria B, Ripoll C, Nadal A. Low doses of the endocrine disruptor bisphenol-A and the native hormone 17-beta-estradiol rapidly activate transcription factor CREB. FASEB J. 2002; 16(12):1671–1673. PMID: 12207000.
17. Ren L, Marquardt MA, Lech JJ. Estrogenic effects of nonylphenol on pS2, ER and MUC1 gene expression in human breast cancer cells-MCF-7. Chem Biol Interact. 1997; 104(1):55–64. PMID: 9158695.


18. Witorsch RJ. Endocrine disruptors: can biological effects and environmental risks be predicted. Regul Toxicol Pharmacol. 2002; 36(1):118–130. PMID: 12383724.


19. Hong E, Choi KC, Jeung EB. Maternal-fetal transfer of endocrine disruptors in the induction of Calbindin-D9k mRNA and protein during pregnancy in rat model. Mol Cell Endocrinol. 2003; 212:63–72. PMID: 14654251.


20. Geisinger KR, Kute TE, Pettenati MJ, Welander CE, Dennard Y, Collins LA, Berens ME. Characterization of a human ovarian carcinoma cell line with estrogen and progesterone receptors. Cancer. 1989; 63(2):280–288. PMID: 2910432.


21. Tabb MM, Blumberg B. New modes of action for endocrine disrupting chemicals. Mol Endocrinol. 2006; 20(3):475–482. PMID: 16037129.
22. McCormick SD, O'Dea MF, Moeckel AM, Lerner DT, Bjornsson BT. Endocrine disruption of parr-smolt transformation and seawater tolerance of Atlantic salmon by 4-nonylphenol and 17beta-estradiol. Gen Comp Endocrinol. 2005; 142(3):280–288. PMID: 15935154.
23. Fisher JS. Environmental anti-androgens and male reproductive health: focus on phthalates and testicular dysgenesis syndrome. Reproduction. 2004; 127(3):305–315. PMID: 15016950.


24. McVey MJ, Cooke GM, Curran IH. Increased serum and testicular androgen levels in F1 rats with lifetime exposure to soy isoflavones. Reprod Toxicol. 2004; 18(5):677–685. PMID: 15219630.


25. Tyler CR, Jobling S, Sumpter JP. Endocrine disruption in wildlife: a critical review of the evidence. Crit Rev Toxicol. 1998; 28(4):319–361. PMID: 9711432.


26. Anway MD, Cupp AS, Uzumcu M, Skinner MK. Epigenetic transgenerational actions of endocrine disruptors and male fertility. Science. 2005; 308(5727):1466–1469. PMID: 15933200.


27. Younglai EV, Holloway AC, Foster WG. Environmental and occupational factors affecting fertility and IVF success. Hum Reprod Update. 2005; 11(1):43–57. PMID: 15601728.


28. Younglai EV, Holloway AC, Lim GE, Foster WG. Synergistic effects between FSH and 1,1-dichloro-2,2-bis(P-chlorophenyl) ethylene (P,P'-DDE) on human granulosa cell aromatase activity. Hum Reprod. 2004; 19(5):1089–1093. PMID: 15070871.
29. Anway MD, Skinner MK. Epigenetic transgenerational actions of endocrine disruptors. Endocrinology. 2006; 147(6 Suppl):S43–S49. PMID: 16690803.


30. An BS, Choi KC, Kang SK, Lee GS, Hong EJ, Hwang WS, Jeung EB. Mouse calbindin-D(9k) gene expression in the uterus during late pregnancy and lactation. Mol Cell Endocrinol. 2003; 205(1-2):79–88. PMID: 12890569.


31. Lee GS, Kim HJ, Jung YW, Choi KC, Jeung EB. Estrogen receptor alpha pathway is involved in the regulation of Calbindin-D9k in the uterus of immature rats. Toxicol Sci. 2005; 84(2):270–277. PMID: 15635152.
32. Hong EJ, Ji YK, Choi KC, Manabe N, Jeung EB. Conflict of estrogenic activity by various phthalates between in vitro and in vivo models related to the expression of Calbindin-D9k. J Reprod Dev. 2005; 51(2):253–263. PMID: 15883486.


33. Hong EJ, Choi KC, Jeung EB. Induction of calbindin-D9k messenger RNA and protein by maternal exposure to alkylphenols during late pregnancy in maternal and neonatal uteri of rats. Biol Reprod. 2004; 71(2):669–675. PMID: 15070829.
34. Hong EJ, Choi KC, Jeung EB. Maternal exposure to bisphenol a during late pregnancy resulted in an increase of Calbindin-D9k mRNA and protein in maternal and postnatal rat uteri. J Reprod Dev. 2005; 51(4):499–508. PMID: 15947454.


35. Hong EJ, Choi KC, Jung YW, Leung PC, Jeung EB. Transfer of maternally injected endocrine disruptors through breast milk during lactation induces neonatal Calbindin-D9k in the rat model. Reprod Toxicol. 2004; 18(5):661–668. PMID: 15219628.


36. Hong EJ, Choi KC, Jeung EB. Maternal-fetal transfer of endocrine disruptors in the induction of Calbindin-D9k mRNA and protein during pregnancy in rat model. Mol Cell Endocrinol. 2003; 212:63–72. PMID: 14654251.


37. Lee GS, Choi KC, Kim HJ, Jeung EB. Effect of genistein as a selective estrogen receptor beta agonist on the expression of Calbindin-D9k in the uterus of immature rats. Toxicol Sci. 2004; 82(2):451–457. PMID: 15456916.


38. Schrader TJ, Cooke GM. Effects of Aroclors and individual PCB congeners on activation of the human androgen receptor in vitro. Reprod Toxicol. 2003; 17(1):15–23. PMID: 12507654.


39. Spearow JL, Barkley M. Reassessment of models used to test xenobiotics for oestrogenic potency is overdue. Hum Reprod. 2001; 16(5):1027–1029. PMID: 11331655.


40. Park SH, Kim KY, An BS, Choi JH, Jeung EB, Leung PC, Choi KC. Cell growth of ovarian cancer cells is stimulated by xenoestrogens through an estrogen-dependent pathway, but their stimulation of cell growth appears not to be involved in the activation of the mitogen-activated protein kinases ERK-1 and p38. J Reprod Dev. 2009; 55(1):23–29. PMID: 18854640.


Figure 1
Expression of ERα and ERβ proteins in estrogen-responsive ovarian cancer cells (BG-1). The protein was isolated and prepared as described in the Materials and Methods. The protein levels of ERα (68 kDa) and ERβ (55 kDa) were detected by immunoblot analysis by using an ERα or ERβ monoclonal antibody in BG-1 cells.
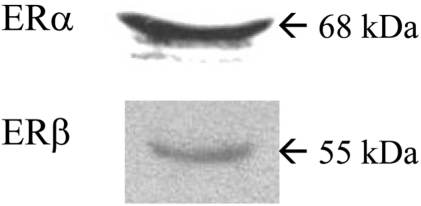
Figure 2
Hierarchical clustering analysis. A hierarchical clustering analysis was performed following treatments with 17β-estradiol (E2) and bisphenol A (BPA) at different time points (1, 8, and 24 h) in BG-1 cells. Two-dimensional hierarchical clustering was applied to the expression data from approximate 8.0 k genes, which showed significant changes in the balanced differential expression. Increased expression levels were shown in red and decreased expression levels shown in green.
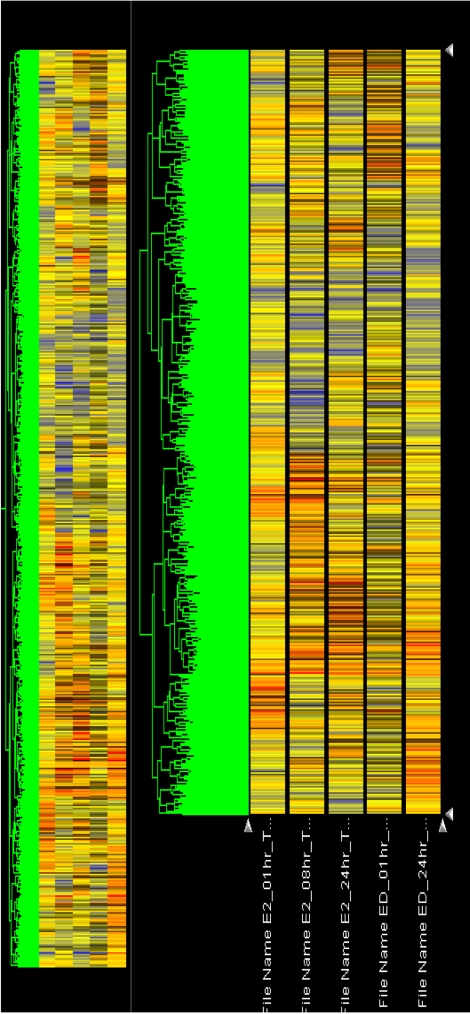
Figure 3
Confirmation of gene profiles by real-time polymerase-chain reaction (PCR) analysis. Relative values of expression of the altered genes quantified by real-time PCR were shown in the graph, indicating the comparison of fold change determined by real-time PCR analysis by 17β-estradiol (E2, 10-7 M) or bisphenol A (BPA, 10-5 M) following 24 h treatment in BG-1 cells. The representative genes included RAB31_MEMBER RAS ONCOGENE FAMILY (U59877), CYCLIN D1 (X59798), CYCLIN-DEPENDENT KINASE 4 (U37022), INSULIN-LIKE GROWTH FACTOR-BINDING PROTEIN 4 (U20982), and ANTI-MULLERIAN HORMONE (NM_000479). *P<0.05 vs. vehicle.
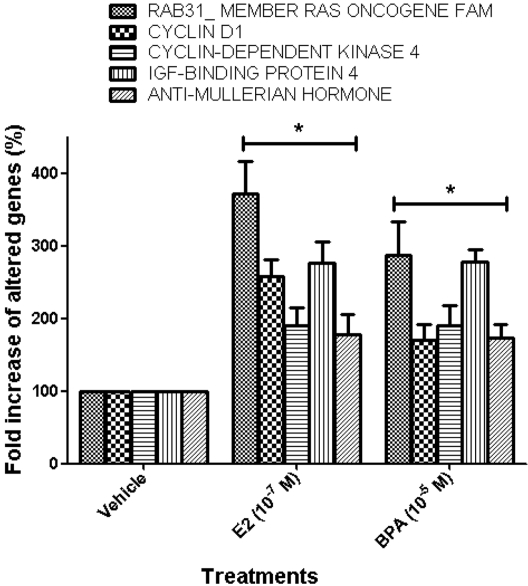