This article has been
cited by other articles in ScienceCentral.
Abstract
Streptococcus pneumoniae is a major pathogen that causes various diseases, including pneumonia and sepsis, as millions of people suffer from S. pneumoniae infection worldwide. To better understand the immune and inflammatory responses to S. pneumoniae, we produced murine models. To investigate the differences between intranasal and intratracheal infection, BALB/c mice were infected with S. pneumoniae D39 intranasally or intratracheally. Mice showed no significant differences in survival rates, body weight changes, and bacterial loads. To investigate resistance and susceptibility among mouse strains, BALB/c, C57BL/6J, tumor necrosis factor-α (TNF-α) knockout, and interleukin-10 (IL-10) knockout mice were infected with S. pneumoniae D39 via intranasal or intravenous routes. In this study, BALB/c and C57BL/6J mice were resistant, IL-10 knockout mice were intermediate, and TNF-α knokout mice were susceptible to S. pneumoniae infection. These data show that intranasal and intratracheal infection induced similar results after S. pneumoniae infection, and the genetic background of mice must be considered when studying S. pneumoniae infection in vivo.
Go to :

Keywords: Genetic background, knockout mouse, Streptococcus pneumoniae
Streptococcus (S) pneumoniae, a pneumococcus, is an important pathogen that causes various infectious diseases, such as pneumonia, septicemia, meningitis, sinusitis, and otitis media [
1]. Pneumococcal pneumonia is especially common among children and elderly people, who have weak immunity. Despite the availability of excellent antibiotics therapy,
S. pneumoniae continues to induce considerable morbidity and mortality worldwide. For instance, it has been reported that about 800,000 children younger than 5 years old die due to
S. pneumoniae infection worldwide each year, and 90% of cases occur in developing countries [
2]. Two major problems in the control of
S. pneumoniae diseases are increased antibiotic resistance and restricted efficacy of the vaccine [
3,
4]. During the past three decades, antimicrobial resistance to
S. pneumoniae has severely increased, with rapid spreading of antibiotic-resistant
S. pneumoniae having been observed in Southern and Eastern Europe, North America, South America, Africa, and Asia [
5]. Several vaccines are used for the prevention of
S. pneumoniae infection, but there still are limitations. The most commonly used 23-valent polysaccharide vaccine is effective against only 23 out of 90 serotypes and does not confer a protective effect in children younger than 2 years of age or in elderly people [
6]. A 7-valent pneumococcal conjugate vaccine, which is effective in children, has also been available since 2000, but it is expensive and protects only against seven serotypes [
6].
Despite the importance of
S. pneumoniae as a major human pathogen, interactions between host and pathogen are not understood clearly. Therefore, study of the virulent factors of
S. pneumoniae as well as the host immune defense mechanisms against
S. pneumoniae infection in an animal model is required to better understand and control this disease. Recently, it was shown that susceptibility and resistance to
S. pneumoniae differ among mouse strains [
7,
8]. Furthermore, the effect of pneumolysin, a virulence factor of
S. pneumoniae, is dependent on the genetic background of the mice [
9].
During bacterial infection, the immune and inflammatory responses are characterized by complex and dynamic processed that are associated with the expression of both pro- and anti-inflammatory cytokines and chemokines [
10]. tumor necrosis factor-α (TNF-α) is a pro-inflammatory cytokine that activates immune and inflammatory responses. TNF-α has helpful [
11] as well as damaging effects [
12] on the host response to infection. Interleukin-10 (IL-10) is an anti-inflammatory cytokine that down-regulates pro-inflammatory cytokines such as TNF-α and interferin-γ (IFN-γ). During
S. pneumoniae-induced pneumonia, IL-10 attenuates the pro-inflammatory cytokine response in the lungs, hampers effective clearance of infection, and shortens survival [
13].
To compare the differences between intranasal (IN) and intratracheal (IT) infection with S. pneumoniae, we infected BALB/c mice intranasally or intratracheally. To investigate the susceptibility and resistance of mouse strains as well as the role of TNF-α and IL-10 in response to S. pneumoniae infection, we produced a murine model of pneumococcal disease using four mouse strains: BALB/c, C57BL/6J, TNF-α knockout (KO), and IL-10 KO mice. We infected mice with different numbers of S. pneumoniae by IN or intravenous (IV) routes, and observed the survival rates and body weight changes.
Materials and Methods
Animals
Male and female BALB/c, C57BL/6J, TNF-α KO (B6.129S-Tnftm1Gkl/J), and IL-10 KO (B6.129P2-Il10tm1Cgn/J) mice 7 to 8 weeks old were used in this study. BALB/c and C57BL/6J mice were purchased from Korea Research Institute of Bioscience and Biotechnology (KRIBB, Daejeon, Korea). TNF-α KO and IL-10 KO mice were purchased from Jackson Laboratory (Bar Harbor, Maine, USA). Animals were maintained in a specific pathogen free barrier facility at the College of Veterinary Medicine at Konkuk University (Seoul, Korea). Animals were allowed free access to sterilized food and water. Animal room was maintained in a 12 hour light-dark cycle, and the room temperature was maintained at 22±2℃ with 50±10% relative humidity. This study was approved by the Institutional Animal Care and Use Committee (IACUC) of Konkuk University.
Bacterial preparation
S. pneumoniae D39 serotype 2 was obtained from The Korea Centers for Disease Control and Prevention (KCDC, Osong, Korea). Bacteria were cultured on a 5% sheep blood agar plate at 37℃ and 5% CO2 for 18 hours. Bacteria were harvested, rinsed, and resuspended with sterilized phosphate buffered saline (PBS) and then transferred to brain-heart infusion (Merck, Darmstadt, Germany) broth, followed by culture at 37℃ and 5% CO2 for 6 hours. Bacteria were pelleted by centrifugation, washed, and resuspended with sterilized PBS. Bacterial concentrations were estimated by measuring the absorbance value at an optical density of 600 nm using a spectrophotometer. Colony forming units (CFU)/mL were counted by plating the serially diluted bacterial suspension.
Infection
Mice were anesthetized by intraperitoneal administration of 40 mg/kg of Zoletil™ (Virbac Laboratories, Carros, France) and 5 mg/kg of Rompun™ (Bayer Korea, Anseong, Korea). To compare the differences between IN and IT infection, 20 µL of the S. pneumoniae suspension containing 2×107 CFU was inoculated in male BALB/c mice via the nostril (IN) or trachea (IT). Briefly, a small incision was made to expose the trachea, and the bacterial suspension was injected intratracheally using a 31-gauge needle. The incision was closed using surgical staples.
To compare the resistance to pneumococcal disease among the infection routes and infectious doses, 2×102 to 2×104 and 2×106 to 2×108 CFU of bacterial suspensions were infected into BALB/c, C57BL/6J, TNF-α KO and IL-10 KO mice via the lateral tail vein and nostril, respectively. Male mice were used for the intranasal infection study while female mice were used for the intravenous infection study. After infection, survival and body weight of the mice were measured every 24 hours for 10 days.
CFU analysis
After anesthetization by peritoneal infection of 40 mg/kg of Zoletil™ (Virbac Laboratories) and 5 mg/kg of Rompun™ (Bayer Korea), blood was collected from the axillary vessels. Exactly 20 µL of blood was diluted serially and used for CFU analysis. The nasopharynx and lung were then removed from each mouse, followed by homogenization by a tissue homogenizer in sterile PBS and serial dilution. Diluted blood and homogenates were cultured on 5% sheep blood agar plates at 37℃ and under 5% CO2 atmosphere for 24 hours, after which CFU were counted.
Statistical analysis
Body weight changes and bacterial counts are expressed as mean±SD. Significant differences between the groups were evaluated using unpaired Student's t-test. Survival rates were compared by a log-rank test. Data analysis was performed by Prism 5 for Windows (Graphpad Software Inc.). P<0.05 was considered to be statistically significant.
Go to :

Results
Comparing intranasal infection with intratracheal infection
To compare IN and IT infection, we measured survival rate and changes in body weight after infection with 2×10
7 CFU of
S. pneumoniae D39 (
Figure 1). Mice infected by IN administration began to die at 3 days after infection, whereas mice infected by IT administration began to die at 2 days after infection. At 5 days after infection, the survival rates of mice infected intranasally and intratracheally were 60% and 50%, respectively. IT infection resulted in a 10% higher death rate compared to IN infection, but no significant difference was observed between the two groups. The body weights of the mice infected intranasally and intratracheally decreased until 4 days after infection and began to increase from 5 days after infection. In the early and late phases of infection, body weight changes were significantly different (
P<0.05) between the two groups. Additionally, at 12 hours after infection, both the IN and IT groups demonstrated increased bacterial numbers in the nasopharynx, lung, and blood. Specifically, bacterial numbers in the blood of intranasally infected mice were significantly (
P<0.05) lower than those in the blood of intratracheally infected mice at this time. At 36 hours after infection, bacterial numbers in the lung of intranasally infected mice were significantly (
P<0.05) higher than those of in the lung of intratracheally infected mice. Based on these observations, there were no significant differences between IN and IT infection (
Figure 2).
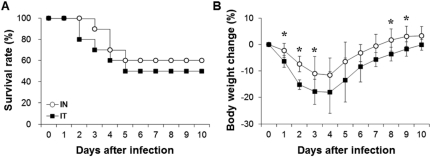 | Figure 1Survival rates (A) and body weight changes (B) of BALB/c mice infected with 2×107 CFU of S. pneumoniae D39 serotype 2 via intranasal (IN) and intratracheal (IT) routes. Data are means±SD. *P<0.05 versus IT infection at each time point. 
|
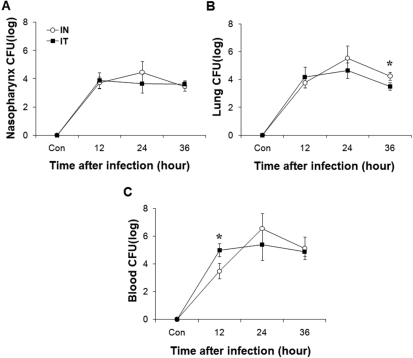 | Figure 2Bacterial numbers in the nasopharynx (A), lung (B), and blood (C) of BALB/c mice infected with 2×107 CFU of S. pneumoniae D39 serotype 2 via intranasal (IN) and intratracheal (IT) routes. Data are means±SD. *P<0.05 versus IN or IT infection at each time point. 
|
Comparing the bacterial resistance of intranasally infected mice by infectious dose
To compare bacterial resistance by infectious dose, BALB/c, C57BL6/J, TNF-α KO, and IL-10 KO mice were infected intranasally with
S. pneumoniae D39. The results show marked differences in survival rates and body weight changes among the mouse strains (
Figure 3). After infection with 2×10
6 CFU of
S. pneumoniae D39, BALB/c and C57BL/6J mice showed high resistance. The survival rate was 87.5% in both the BALB/c and C57BL/6J strains. One BALB/c mouse died at 4 days after infection, but the remaining seven mice survived until 10 days after infection, at which time the experiment was ended. In BALB/c mice, no body weight changes were observed for 4 days after infection, but increases occurred from 5 days after infection. One C57BL/6J mouse died at 5 days after infection, and no more dead mice were observed during the experiment. No difference in the survival rate was observed between BALB/c and C57BL/6J mice. C57BL/6J mice underwent slight body weight gains from 6 days after infection. TNF-α KO mice were susceptible to
S. pneumoniae D39 infection. One TNF-α KO mouse began to die at 3 days after infection, and 62.5% of the mice died at 4 days after infection. The remaining 25% mice died gradually until 7 days after infection. TNF-α KO mice showed significantly (
P<0.01) lower survival rate than the other three mouse strains. IL-10 KO mice began to die at 4 days after infection, and 50% of the IL-10 KO mice survived until the end of the experiment. Body weights of TNF-α KO and IL-10 KO mice decreased after infection, and both TNF-α KO and IL-10 KO mice did not recover their body weight.
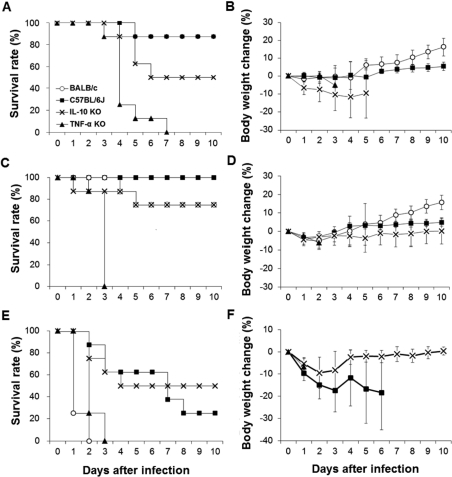 | Figure 3Survival rates (A, C, and E) and body weight changes (B, D, and F) of BALB/c, C57BL6J, IL-10 knockout, and TNF-α knockout mice. Mice were infected with 2×106 (A and B), 2×107 (C and D) and 2×108 (E and F) CFU of S. pneumoniae D39 serotype 2 via intranasal route. Data are means±SD. 
|
Mice infected intranasally with 2×107 CFU of S. pneumoniae D39 began to die earlier than mice infected with 2×106 CFU of S. pneumoniae D39. One IL-10 KO mouse began to die at 1 day after infection, whereas TNF-α KO mice began to die at 2 days after infection. Surprisingly, all of the remaining TNF-α KO mice died at 3 days after infection. At the end of the experiment, all of the C57BL/6J mice, 75% of the BALB/c, and 75% of the IL-10 KO mice survived. TNF-α KO mice showed a significantly (P<0.01) lower survival rate compared to the other mouse strains. Body weights of the infected mice decreased during early phase of infection, after which they began to increase continuously except in TNF-α KO mice. TNF-α KO mice showed continuous body weight loss after infection.
Mice infected intranasally with 2×108 CFU of S. pneumoniae D39 experienced the most severe lethality and body weight losses. Interestingly, 75% of the BALB/c mice began to die at 1 day after infection, whereas the remaining 25% died at 2 days after infection. On the other hand, BALB/c mice showed high resistance to 106 and 107 CFU of S. pneumoniae D39 infection. Regarding TNF-α KO mice, 75% began to die at 2 days after infection, whereas the remaining 25% died at 3 days after infection. C57BL/6J and IL-10 KO mice also began to die at 2 days after infection, whereas 25% of the C57BL/6J mice and 50% of the IL-10 KO mice survived until the end of the experiment. TNF-α KO and BALB/c mice showed a significantly (P<0.05) lower survival rate compared to the C57BL/6J and IL-10 KO mice. The BALB/c, C57BL/6J, and TNF-α KO mice experienced continuous losses in body weight, but the IL-10 KO mice recovered.
Comparing the bacterial resistance of intravenously infected mice by infectious dose
To compare bacterial resistance by infectious dose, BALB/c, C57BL6/J, TNF-α KO, and IL-10 KO mice were infected intravenously with
S. pneumoniae D39. The results show marked differences in survival rates and body weight changes among the mouse strains (
Figure 4). BALB/c and C57BL6/J mice were resistant to
S. pneumoniae D39 infection. After infection with 2×10
2 CFU of
S. pneumoniae D39, one BALB/c mouse began to die at 2 days after infection, whereas the remaining mice survived until the end of the experiment. All of the C57BL/6J mice survived until the end of the experiment. Exactly 37.5% of the TNF-α KO mice began to die at 2 days after infection, whereas 50% died at 3 days after infection. One IL-10 KO mouse began to die at 3 days after infection, and 75% mice survived until the end of the experiment. TNF-α KO mice showed a significantly lower survival rate compared to the other three mouse strains (
P<0.01). The body weights of the BALB/c, C57BL/6J, and IL-10 KO mice slightly increased, whereas that of TNF-α KO mice decreased after infection. Body weight of BALB/c mice increased continuously after infection.
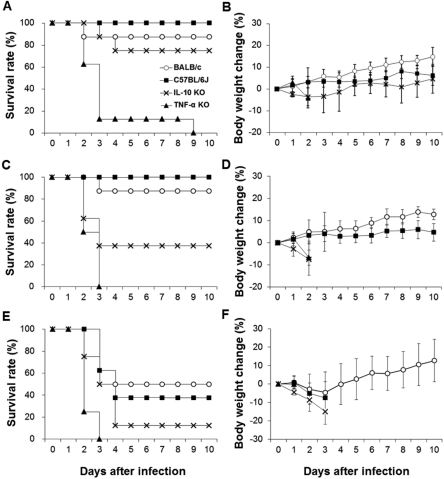 | Figure 4Survival rates (A, C, and E) and body weight changes (B, D, and F) of BALB/c, C57BL6J, IL-10 knockout, and TNF-α knockout mice. Mice were infected with 2×102 (A and B), 2×103 (C and D) and 2×104 (E and F) CFU of S. pneumoniae D39 serotype 2 via intravenous route. Data are means±SD. 
|
After mice were infected intravenously with 2×103 CFU of S. pneumoniae D39, exactly 87.5% of the BALB/c mice and all of the C57BL/6J mice survived until the end of the experiment. Both the IL-10 KO and TNF-α KO mice began to die at 2 days after infection. All of the TNF-α KO and 62.5% of the IL-10 KO mice died within 3 days after infection. The remaining 37.5% IL-10 KO mice survived until the end of the experiment. TNF-α KO mice showed a significantly (P<0.01) lower survival rate compared to the BALB/c and C57BL/6J mice, whereas the IL-10 KO mice showed a significantly (P<0.05) lower survival rate compared to the C57BL/6J mice. The body weights of the BALB/c and C57BL/6J mice slightly increased, whereas those of the TNF-α KO and IL-10 KO mice decreased after infection.
After mice infected intravenously with 2×104 CFU of S. pneumoniae D39, surprisingly, 75% of the TNF-α KO mice died at 2 days after infection, whereas the remaining 25% died at 3 days after infection. The IL-10 mice began to die at 2 days after infection, and the remaining 87.5% died within 4 days after infection. Exactly 50% of the BALB/c mice died within 3 days after infection, and 62.5% of the C57BL/6J mice died within 4 days. TNF-α KO mice showed a significantly (P<0.05) lower survival rate compared to the BALB/c and C57BL/6J mice. Body weight of the BALB/c mice decreased after infection and recovered, whereas those of the C57BL/6J, TNF-α KO, and IL-10 mice decreased after infection.
Go to :

Discussion
Currently, interactions between the host and S. pneumoniae are not understood clearly. To better understand the host defense mechanism against S. pneumoniae infection, we produced a S. pneumoniae disease mouse model using BALB/c, C57BL/6J, TNF-α KO, and IL-10 KO mouse strains. Various CFU of S. pneumoniae D39 serotype 2 were inoculated by IN, IT, and IV routes, and survival rates and body weight changes were monitored. Each strain demonstrated a distinct immune response and showed a different survival rate and body weight after S. pneumoniae infection. This study compared IN and IT infection between resistant and susceptible mouse strains and explored the roles of TNF-α and IL-10 during S. pneumoniae D39 infection.
IT infection in BALB/c mice resulted in a higher rate of mortality, more rapid death, and more body weight loss compared to IN infection, but no significant differences were observed between the two groups. These findings suggest that noninvasive IN infection induced similar results compared to IT infection. The advantages of IT infection are the perfect delivery of the bacterial inoculums and development of pneumonia, but IT infection requires practice and invasive surgical procedures for induction of disease [
14]. On the other hand, IN infection is easy and fast to carry out without complex and invasive surgical techniques and mimics the natural route of infection [
6]. For these reasons, IN infection is the most commonly used method. In this study, similar CFU in the nasopharynx, blood, and lung were observed in both infection models. Thus, we used the noninvasive IN method for the next experiment.
If BALB/c, C57BL/6J, TNF-α KO, and IL-10 KO mice are divided into resistant, intermediate, and susceptible strains based on their survival rates in this study, BALB/c and C57BL/6J mice were the resistant strains, IL-10 KO mouse was the intermediate strain, and TNF-α KO mouse was the susceptible strain. The most resistant strain was the C57BL/6J mice while the second most resistant strain was BALB/c mice, but a significant difference between the survival rates of the BALB/c and C57BL/6J mice was not observed using both infection routes with 2×10
6 or 2×10
7 CFU of
S. pneumoniae D39. However, when BALB/c mice were infected intranasally with 2×10
8 CFU of
S. pneumoniae D39, interestingly, the survival rate of BALB/c mice was significantly lower than those of C57BL/6J and IL-10 KO mice. However, in a previous study, BALB/c mice were resistant and C57BL/6 mice were intermediate to
S. pneumoniae D39 infection [
7], even though the infectious dose of was lower than that of our experiment. Another previous study reported that a significant difference in the survival rate did not occur between the BALB/c and C57BL/6J mice infected with 5×10
7 CFU of
S. pneumoniae WU2 [
8]. Based on previous and our studies, the differences in susceptibility between the mouse strains may be dependent on the infectious dose and the bacterial strain.
TNF-α has been reported to play important roles in bacterial clearance and survival in response to
S. pneumoniae infection [
15-
18]. Administration of anti-TNF-α monoclonal antibody leads to impaired recruitment of neutrophils, impaired bacterial clearance, and accelerated death after infection with
S. pneumoniae [
19]. In this study, TNF-α KO mice showed the most susceptibility to
S. pneumoniae D39 infection. All of the TNF-α KO mice infected with
S. pneumoniae D39 died before 9 days after infection. This may have been due to impaired bacterial clearance due to the lack of TNF-α. The time between infection and death in our study quickly decreased as the infectious dose of
S. pneumoniae D39 was increased.
IL-10 acts as an important regulator of the immune response by limiting the inflammatory response and protecting the host from tissue damage caused by excessive inflammation [
20]. This prevention is based on the down-regulation of the production of pro-inflammatory cytokines and chemokines and the reduction of the expression of adhesion molecules of
S. pneumoniae [
21]. In the case of intranasal infection, interestingly, the survival rates of IL-10 KO mice did not decrease as the infectious dose of
S. pneumoniae D39 was increased. The survival rates of IL-10 KO mice after IN infection with 10
6, 10
7, and 10
8 CFU of
S. pneumoniae D39 were 50, 75, and 50%, respectively. Furthermore, IL-10 KO mice showed the highest survival rate among the mice infected intranasally with 10
8 CFU of
S. pneumoniae D39. This may have resulted from the enhanced inflammatory response of IL-10 KO mice due to increased pro-inflammatory cytokine and chemokine production in response to a high dose of
S. pneumoniae. To conform this result, further research is be needed.
In summary, the genetic background of the mouse, serotype, infectious dose of S. pneumoniae, infection route, and production of cytokines associated with the immune response have to be considered when studying the pathogenesis of S. pneumoniae diseases in a murine model.
Go to :

Acknowledgments
This research was supported by a fund (2010E4600400) by Research of Korea Centers for Disease Control and Prevention.
Go to :

References
1. Kadioglu A, Andrew PW. The innate immune response to pneumococcal lung infection: the untold story. Trends Immunol. 2004; 25(3):143–149. PMID:
15036042.

2. O'Brien KL, Wolfson LJ, Watt JP, Henkle E, Deloria-Knoll M, McCall N, Lee E, Mulholland K, Levine OS, Cherian T. Burden of disease caused by
Streptococcus pneumoniae in children younger than 5 years: global estimates. Lancet. 2009; 374(9693):893–902. PMID:
19748398.
3. Finkelstein JA, Huang SS, Daniel J, Rifas-Shiman SL, Kleinman K, Goldmann D, Pelton SI, DeMaria A, Platt R. Antibiotic-resistant
Streptococcus pneumoniae in the heptavalent pneumococcal conjugate vaccine era: predictors of carriage in a multicommunity sample. Pediatrics. 2003; 112(4):862–869. PMID:
14523178.
4. Huang SS, Hinrichsen VL, Stevenson AE, Rifas-Shiman SL, Kleinman K, Pelton SI, Lipsitch M, Hanage WP, Lee GM, Finkelstein JA. Continued impact of pneumococcal conjugate vaccine on carriage in young children. Pediatrics. 2009; 124(1):e1–e11. PMID:
19564254.

5. Liñares J, Ardanuy C, Pallares R, Fenoll A. Changes in antimicrobial resistance, serotypes and genotypes in
Streptococcus pneumoniae over a 30-year period. Clin Microbiol Infect. 2010; 16(5):402–410. PMID:
20132251.
6. Chiavolini D, Pozzi G, Ricci S. Animal models of
Streptococcus pneumoniae disease. Clin Microbiol Rev. 2008; 21(4):666–685. PMID:
18854486.
7. Gingles NA, Alexander JE, Kadioglu A, Andrew PW, Kerr A, Mitchell TJ, Hopes E, Denny P, Brown S, Jones HB, Little S, Booth GC, McPheat WL. Role of genetic resistance in invasive pneumococcal infection: identification and study of susceptibility and resistance in inbred mouse strains. Infect Immun. 2001; 69(1):426–434. PMID:
11119534.

8. Mizrachi-Nebenzahl Y, Lifshitz S, Teitelbaum R, Novick S, Levi A, Benharroch D, Ling E, Dagan R. Differential activation of the immune system by virulent
Streptococcus pneumoniae strains determines recovery or death of the host. Clin Exp Immunol. 2003; 134(1):23–31. PMID:
12974750.
9. Benton KA, Paton JC, Briles DE. The hemolytic and complement-activating properties of pneumolysin do not contribute individually to virulence in a pneumococcal bacteremia model. Microb Pathog. 1997; 23(4):201–209. PMID:
9344781.

10. Standiford TJ, Kunkel SL, Greenberger MJ, Laichalk LL, Strieter RM. Expression and regulation of chemokines in bacterial pneumonia. J Leukoc Biol. 1996; 59(1):24–28. PMID:
8558063.

11. Klebanoff SJ, Vadas MA, Harlan JM, Sparks LH, Gamble JR, Agosti JM, Waltersdorph AM. Stimulation of neutrophils by tumor necrosis factor. J Immunol. 1986; 136(11):4220–4225. PMID:
3009619.
12. Sriskandan S, Cohen J. Gram-positive sepsis. Mechanisms and differences from gram-negative sepsis. Infect Dis Clin North Am. 1999; 13(2):397–412. PMID:
10340174.
13. van der Poll T, Marchant A, Keogh CV, Goldman M, Lowry SF. Interleukin-10 impairs host defense in murine pneumococcal pneumonia. J Infect Dis. 1996; 174(5):994–1000. PMID:
8896500.
14. Rubins JB, Charboneau D, Fasching C, Berry AM, Paton JC, Alexander JE, Andrew PW, Mitchell TJ, Janoff EN. Distinct roles for pneumolysin's cytotoxic and complement activities in the pathogenesis of pneumococcal pneumonia. Am J Respir Crit Care Med. 1996; 153(4 Pt 1):1339–1346. PMID:
8616564.

15. Takashima K, Tateda K, Matsumoto T, Iizawa Y, Nakao M, Yamaguchi K. Role of tumor necrosis factor alpha in pathogenesis of pneumococcal pneumonia in mice. Infect Immun. 1997; 65(1):257–260. PMID:
8975920.

16. O'Brien DP, Briles DE, Szalai AJ, Tu AH, Sanz I, Nahm MH. Tumor necrosis factor alpha receptor I is important for survival from
Streptococcus pneumoniae infections. Infect Immun. 1999; 67(2):595–601. PMID:
9916064.
17. Rijneveld AW, Florquin S, Branger J, Speelman P, Van Deventer SJ, van der Poll T. TNF-alpha compensates for the impaired host defense of IL-1 type I receptor-deficient mice during pneumococcal pneumonia. J Immunol. 2001; 167(9):5240–5246. PMID:
11673538.
18. Hatta M, Yamamoto N, Miyazato A, Ishii N, Nakamura K, Inden K, Aoyagi T, Kunishima H, Hirakata Y, Suzuki K, Kaku M, Kawakami K. Early production of tumor necrosis factor-alpha by Gr-1 cells and its role in the host defense to pneumococcal infection in lungs. FEMS Immunol Med Microbiol. 2010; 58(2):182–192. PMID:
19909342.
19. van der Poll T, Keogh CV, Buurman WA, Lowry SF. Passive immunization against tumor necrosis factor-alpha impairs host defense during pneumococcal pneumonia in mice. Am J Respir Crit Care Med. 1997; 155(2):603–608. PMID:
9032201.

20. Sanjabi S, Zenewicz LA, Kamanaka M, Flavell RA. Anti-inflammatory and pro-inflammatory roles of TGF-beta, IL-10, and IL-22 in immunity and autoimmunity. Curr Opin Pharmacol. 2009; 9(4):447–453. PMID:
19481975.
21. Kerr AR, Irvine JJ, Search JJ, Gingles NA, Kadioglu A, Andrew PW, McPheat WL, Booth CG, Mitchell TJ. Role of inflammatory mediators in resistance and susceptibility to pneumococcal infection. Infect Immun. 2002; 70(3):1547–1557. PMID:
11854243.

Go to :
