Abstract
Inducible nitric oxide synthase (iNOS) is a main enzyme producing nitric oxide during inflammation and thus contributes to the initiation and development of inflammatory cardiovascular diseases such as atherosclerosis. Epigallocatechin-3-gallate (EGCG), the major catechin derived from green tea, has multiple beneficial effects for treating cardiovascular disease, but the effect of EGCG on the expression of vascular iNOS remains unknown. In this study, we investigated (i) whether EGCG inhibits the expression of vascular iNOS induced by angiotensin II in human umbilical vein endothelial cells and, if it does inhibit, (ii) mechanisms underlying the inhibition. Angiotensin II increased expression levels of vascular iNOS; EGCG counteracted this effect. EGCG increased the production of reactive oxygen species. Moreover, EGCG did not affect the production of reactive oxygen species induced by angiotensin II. These data suggest a novel mechanism whereby EGCG provides direct vascular benefits for treating inflammatory cardiovascular diseases.
Atherosclerosis, which has been linked to abnormalities in nitric oxide (NO) signaling, is a chronic systemic disease of the vasculature with an inflammatory component. NO is produced by nitric oxide synthase (NOS), which has three isoforms: neuronal NOS (nNOS), endothelial NOS (eNOS), and inducible NOS (iNOS) [1]. Both eNOS and nNOS are constitutively expressed. In contrast, iNOS expression is inducible by activated macrophages as an immune reaction. During infection, iNOS produces large quantities of NO [2]. iNOS knockout mice decreased diet-induced atherosclerosis [3]. apolipoprotein E (ApoE)/iNOS double knockout mice showed significantly smaller atherosclerotic lesions compared to ApoE knockout mice, suggesting that reduction in iNOS-mediated oxidative stress may protect lesion formation in double knockout mice [4].
Numerous studies have demonstrated that activation of the angiotensin II type 1 (AT1) receptor plays an important role in the pathogenesis of inflammatory diseases. AT1 receptor blockers reduced lipopolysaccharide (LPS)-induced upregulation of iNOS gene and protein expression and iNOS activity, which decreased the general peripheral inflammatory reaction to LPS [5]. LPS-stimulated glomerular iNOS expression was enhanced in diabetic nephropathy, and the activation of angiotensin II may play a role in this enhancement [6]. Olmesartan, a new AT1 receptor blocker, significantly decreased the expression of iNOS in myocardial tissue with a decrease in myocardial inflammation [7]. Losartan, an AT1 receptor blocker, reduced iNOS expression and reestablished the redox status, thus reducing early markers of diabetic retinopathy in a model of diabetes and hypertension [8].
Flavonoids are polyphenolic compounds that are widely distributed in plants, and their consumption might be associated with decreased risks for some chronic degenerative diseases in humans [9]. Epidemiological research in Japan has shown that green tea consumption protects against coronary atherosclerosis [10]. Recent evidence has shown that interleukin-1β (IL-1β)-induced expression of iNOS was markedly inhibited in human chondrocytes pretreated with epigallocatechin-3-gallate (EGCG), a flavanol that is enriched in green tea [11]. EGCG reduced IL-1β and interferon-γ (IFN-γ)-induced NO production, and reduced levels of iNOS mRNA and iNOS protein in RINm5F cells [12]. Ex vivo analysis of β-islets showed that EGCG down-regulates expression of iNOS [13] induced by multiple low doses of streptozotocin. EGCG inhibited effects induced by ultraviolet B (UVB) including activation and translocation of NF-κB, expression of iNOS mRNA and generation of NO, indicating that EGCG protects against UVB-induced skin damage [14]. However, the effect of EGCG on the expression of iNOS, a major risk factor for vascular inflammation, remains unknown. Accordingly, we investigated this knowledge gap using human umbilical vein endothelial cells (HUVECs). This could be clinically important because specific inhibitors of iNOS expression (such as EGCG) might be helpful for the treatment of cardiovascular diseases such as atherosclerosis. We hypothesized that EGCG reduces the expression of iNOS and reactive oxygen species (ROS) that is induced by angiotensin II in HUVECs.
All antibodies for Western blotting were purchased from Santa Cruz Biotechnology (Santa Cruz, CA, USA). The culture medium was obtained from Invitrogen (Carlsbad, CA, USA). Angiotensin II, EGCG and other reagents were purchased from Sigma-Aldrich (St. Louis, MO, USA), unless otherwise specified.
HUVECs were obtained from Clonetics (Walkersville, MD, USA). They were grown in medium 199 containing 0.1 mg/mL heparin, 25 µg/mL endothelial cell growth factor (Biomedical Technologies, Stoughton, MA, USA), 2 mM L-glutamine, 100 U/mL penicillin G, 100 µg/mL streptomycin and 20% fetal bovine serum (FBS). The medium was renewed every two days until confluence, when cells were subcultured at a 1:3 ratio and then cultured in an atmosphere of 95% air and 5% CO2 at 37℃.
HUVEC cultures were starved for 12 h and treated with the desired drugs for the desired times. Cells were lysed in ice-cold buffer (20 mM Tris-HCl pH 7.4, 1% Triton X-100, 150 mM NaCl, 1 mM EDTA, 1 mM EGTA, 2.5 mM sodium pyrophosphate, 1 mM β-glycerol phosphate, 1 mM Na3VO4, 1 mM PMSF and 1 µg/mL leupeptin). The lysates were sonicated and centrifuged (12,000 rpm, 20 min). The protein concentration was measured by the Bradford method. Equal amounts of protein (10 µg) were run on 12% SDS-PAGE and blotted onto polyvinylidene difluoride membranes. These were incubated with rabbit polyclonal antibodies (1:100) against iNOS. Secondary anti-rabbit antibodies and enhanced chemiluminescence (ECL) Plus reagent kits (Amersham, Little Chalfont, Buckinghamshire, UK) were used for detection. Membranes were subsequently exposed to ECL hyperfilms.
To determine whether expression of iNOS, a risk factor for vascular inflammation, is affected by angiotensin II, HUVECs were treated with angiotensin II. Angiotensin II (100 nM) increased the expression of iNOS in a time-dependent manner (Figure 1) causing iNOS levels to increase for the next 24 h. Thus, angiotensin II increases protein levels of vascular endothelial iNOS.
To determine whether angiotensin II-stimulated iNOS expression is affected by EGCG, HUVECs were pretreated for 0.5 h with 10, 30, 50 µM EGCG prior to treatment with angiotensin II (100 nM) for 24 h. Increasing concentrations of EGCG inhibited Ang II-induced iNOS expression (Figure 2) Thus, EGCG, a major catechin obtained from green tea leaf, decreases the protein level of iNOS in a concentration-dependent manner.
To determine whether ROS production is affected by EGCG, HUVECs were pretreated with EGCG. EGCG (10, 30 and 50 µM) increased ROS production compared with control (no treatment), (Figure 3A). Angiotensin II (100 nM) increased ROS production compared with control and this was not prevented by 30 or 50 µM EGCG (Figure 3B). These data suggest that EGCG decreases the protein level of iNOS without affecting ROS.
Inflammation is an important event in the development of vascular diseases such as hypertension, atherosclerosis, and restenosis [15]. Recent knockout studies on iNOS have clarified the role of NO in inflammatory responses [16]. Plasma levels of the proinflammatory cytokines tumour necrosis factor-α (TNF-α), IL-6, and IL-1β were also significantly reduced in iNOS-knockout mice in comparison with control wild-type mice [17]. Expression of iNOS in the endothelium may be a more important mediator of vascular dysfunction than expression of iNOS in the adventitia [18]. Excess NO resulting from iNOS activation was important in modulating vascular permeability during sepsis [19]. iNOS was partially responsible for pulmonary oxidative and nitrosative stress reactions in a murine model [20]. A selective iNOS inhibitor, ONO1714, retarded the development of atherosclerosis [21] induced by a high-cholesterol diet. Increased iNOS activity was found in circulating neutrophils of septic shock patients compared to healthy volunteers [22].
Angiotensin II, a peptide hormone, also plays an important role in atherosclerosis. Ang II, through activation of NF-κB-mediated pro-inflammatory genes, promoted vascular inflammation, leading to acceleration of atherosclerosis [23]. Inhibition of NF-κB by the antioxidant pyrrolidine dithiocarbamate (PDTC) markedly reduced inflammation and iNOS expression in a double transgenic rat model, in which rats transgenic for the human angiotensinogen and renin genes are crossed [24]. Angiotensin II via AT1 receptor increased the accumulation of neutrophils and iNOS expression and played a significant role in mediating inflammation in gastric mucosa exposed to ischemia-reperfusion [25]. Irbesartan, an AT1 receptor antagonist, attenuated atherosclerosis. This effect was partly related to the inhibition of oxidative stress and inflammatory signal transduction pathways in high cholesterol-diet ApoE knockout mice [26]. Increased formation of angiotensin II and iNOS in infiltrated macrophages and medial smooth muscle cells (SMCs) might play important roles in the development and progression of human coronary atherosclerosis [27].
Catechin, a flavanol derived from green tea, possesses antioxidant, antiangiogenesis and antiproliferation activities during the prevention and treatment of cardiovascular diseases [28]. EGCG decreased the risk of cardiovascular disease by reducing inflammatory markers in rats fed an atherogenic diet [29]. In the EGCG groups, iNOS expression was reduced in 1-methyl-4-phenyl-1,2,3,6-tetrahydropyridine (MPTP)-treated mice [30]. EGCG activated an adenosine 5'-monophosphate-activated protein kinase and blocked LPS/IFN-γ-induced iNOS expression, supernatant NO and IL-6 in mesangial cells from MRL/lpr lupus-like mice [31]. Pretreatment of NHBE cells with EGCG resulted in a significant down-regulation of NF-κB-regulated proteins such as cyclin D1, matrix metalloproteinase-9 (MMP-9), IL-8 and iNOS [32]. In the ventral prostate, EGCG significantly reduced cell proliferation, induced apoptosis, and decreased androgen receptors, insulin-like growth factor-1 (IGF-1), IGF-1 receptor, phospho-extracellular signal-regulated kinases 1 and 2, cyclooxygenase-2 (COX-2), and iNOS [33]. Administration of EGCG significantly suppressed iNOS transgene induction by IFN-γ and LPS [34].
In this study, we investigated whether EGCG reduces angiotensin II-mediated iNOS expression in HUVECs. Angiotensin II increased the levels of iNOS (Figure 1). EGCG prevented the iNOS expression induced by angiotensin II in a concentration-dependent manner (Figure 2). This novel finding for the effects of green tea/EGCG is complemented by findings that resveratrol, an important antioxidant found in grapes and wine, diminished IFN-γ-induced levels of iNOS protein, attenuated iNOS mRNA levels, and inhibited IFN-γ-induced promoter activity of the iNOS gene [35]. Resveratrol inhibited NO production by NO scavenging and down-regulation of iNOS expression in RAW 264.7 cells [36]. Moreover, quercetin, a dietary flavonoid, decreased mRNA expression of inducible enzymes such as COX-2 and iNOS, and thus has protective effects against the oxidized low-density lipoproteins (ox-LDL) induced inflammation in peripheral blood mononuclear cells [37]. Quercetin inhibited myocardial ischemia-reperfusion-induced iNOS mRNA and protein expression in rabbit [38]. Sulforaphane, mainly derived from cruciferous vegetables, inhibited the expression of iNOS that had been stimulated by LPS in HUVECs [39]. Together, these results show that flavonoids have a common inhibitory effect on iNOS expression in a number of different cell types in a variety of conditions.
To understand the mechanisms for EGCG attenuation of iNOS expression by angiotensin II, we examined that ROS attenuation in HUVECs induced by EGCG led to an inhibition of iNOS expression. Our data demonstrate that EGCG increases ROS and does not affect ROS increased by angiotensin II (Figure 3). EGCG triggered ROS resulted in chondrosarcoma cell death [40]. However, EGCG decreased the angiotensin II-induced increase of O2- and ROS in vascular smooth muscle cells [41]. Therefore, interference with ROS production by EGCG may be different in different cell types and under a variety of conditions. Alternatively, overproduction of ROS by EGCG might inhibit the expression of iNOS in HUVECs.
In conclusion, the anti-atherosclerotic activity of EGCG is likely not associated with decreased ROS production, although there may be some benefit through inhibiting the expression of iNOS induced by angiotensin II.
References
1. Alderton WK, Cooper CE, Knowles RG. Nitric oxide synthases: structure, function and inhibition. Biochem J. 2001; 357:593–615. PMID: 11463332.


2. Stuehr DJ, Marletta MA. Induction of nitrite/nitrate synthesis in murine macrophages by BCG infection, lymphokines, or interferon-γ. J Immunol. 1987; 139:518–525. PMID: 3110273.
3. Liu VW, Huang PL. Cardiovascular roles of nitric oxide: A review of insights from nitric oxide synthase gene disrupted mice. Cardiovasc Res. 2008; 77:19–29. PMID: 17658499.
4. Kuhlencordt PJ, Chen J, Han F, Astern J, Huang PL. Genetic deficiency of inducible nitric oxide synthase reduces atherosclerosis and lowers plasma lipid peroxides in apolipoprotein E-knockout mice. Circulation. 2001; 103:3099–3104. PMID: 11425775.


5. Sánchez-Lemus E, Benicky J, Pavel J, Larrayoz IM, Zhou J, Baliova M, Nishioku T, Saavedra JM. Angiotensin II AT1 blockade reduces the lipopolysaccharide-induced innate immune response in rat spleen. Am J Physiol Regul Integr Comp Physiol. 2009; 296(5):R1376–R1384. PMID: 19225144.


6. Lee HY, Noh HJ, Gang JG, Xu ZG, Jeong HJ, Kang SW, Choi KH, Han DS. Inducible nitric oxide synthase (iNOS) expression is increased in lipopolysaccharide (LPS)-stimulated diabetic rat glomeruli: effect of ACE inhibitor and angiotensin II receptor blocker. Yonsei Med J. 2002; 43(2):183–192. PMID: 11971212.


7. Seko Y. Effect of the angiotensin II receptor blocker olmesartan on the development of murine acute myocarditis caused by coxsackievirus B3. Clin Sci (Lond). 2006; 110(3):379–386. PMID: 16336207.


8. Silva KC, Rosales MA, de Faria JB, de Faria JM. Reduction of inducible nitric oxide synthase via angiotensin receptor receptor blocker prevents the oxidative retinal damage in diabetic hypertensive rats. Curr Eye Res. 2010; 35(6):519–528. PMID: 20465447.
9. Middleton E Jr, Kandaswami C, Theoharides TC. The effects of plant flavonoids on mammalian cells: Implications for inflammation, heart disease, and cancer. Pharmacol Rev. 2000; 52:673–751. PMID: 11121513.
10. Sasazuki S, Kodama H, Yoshimasu K, Liu Y, Washio M, Tanaka K, Tokunaga S, Kono S, Arai H, Doi Y, Kawano T, Nakagaki O, Takada K, Koyanagi S, Hiyamuta K, Nii T, Shirai K, Ideishi M, Arakawa K, Mohri M, Takeshita A. Relation between green tea consumption and the severity of coronary atherosclerosis among Japanese men and women. Ann Epidemiol. 2000; 10:401–408. PMID: 10964006.


11. Ahmed S, Rahman A, Hasnain A, Lalonde M, Goldberg VM, Haqqi TM. Green tea polyphenol epigallocatechin-3-gallate inhibits the IL-1β-induced activity and expression of cyclooxygenase-2 and nitric oxide synthase-2 in human chondrocytes. Free Radic Biol Med. 2002; 33(8):1097–1105. PMID: 12374621.


12. Han MK. Epigallocatechin gallate, a constituent of green tea, suppresses cytokine-induced pancreatic β-cell damage. Exp Mol Med. 2003; 35(2):136–139. PMID: 12754418.


13. Song EK, Hur H, Han MK. Epigallocatechin gallate prevents autoimmune diabetes induced by multiple low doses of streptozotocin in mice. Arch Pharm Res. 2003; 26(7):559–563. PMID: 12934649.


14. Song XZ, Bi ZG, Xu AE. Green tea polyphenol epigallocatechin-3-gallate inhibits the expression of nitric oxide synthase and generation of nitric oxide induced by ultraviolet B in HaCaT cells. Chin Med J. 2006; 119(4):282–287. PMID: 16537022.


15. Heo SK, Yun HJ, Noh EK, Park WH, Park SD. LPS induces inflammatory responses in human aortic vascular smooth muscle cells via Toll-like receptor 4 expression and nitric oxide production. Immunol Lett. 2008; 120(1-2):57–64. PMID: 18675302.


16. Wei XQ, Charles IG, Smith A, Ure J, Feng GJ, Huang FP, Xu D, Muller W, Moncada S, Liew FY. Altered immune responses in mice lacking inducible nitric oxide synthase. Nature. 1995; 375(6530):408–411. PMID: 7539113.


17. Cuzzocrea S, Chatterjee PK, Mazzon E, Dugo L, De Sarro A, Van de Loo FA, Caputi AP, Thiemermann C. Role of induced nitric oxide in the initiation of the inflammatory response after postischemic injury. Shock. 2002; 18(2):169–176. PMID: 12166782.


18. Gunnett CA, Lund DD, McDowell AK, Faraci FM, Heistad DD. Mechanisms of inducible nitric oxide synthase-mediated vascular dysfunction. Arterioscler Thromb Vasc Biol. 2005; 25(8):1617–1622. PMID: 15933248.
19. Hauser B, Matejovic M, Radermacher P. Nitric oxide, leukocytes and microvascular permeability: causality or bystanders? Crit Care. 2008; 12(1):104. PMID: 18226179.


20. Lange M, Nakano Y, Traber DL, Hamahata A, Esechie A, Jonkam C, Bansal K, Traber LD, Enkhbaatar P. Role of different nitric oxide synthase isoforms in a murine model of acute lung injury and sepsis. Biochem Biophys Res Commun. 2010; 399(2):286–291. PMID: 20655878.


21. Hayashi T, Matsui-Hirai H, Fukatsu A, Sumi D, Kano-Hayashi H, Rani P JA, Iguchi A. Selective iNOS inhibitor, ONO1714 successfully retards the development of high-cholesterol diet induced atherosclerosis by novel mechanism. Atherosclerosis. 2006; 187(2):316–324. PMID: 16325187.


22. Hersch M, Scott JA, Izbicki G, McCormack D, Cepinkas G, Ostermann M, Sibbald WJ. Differential inducible nitric oxide synthase activity in circulating neutrophils vs. mononuclears of septic shock patients. Intensive Care Med. 2005; 31(8):1132–1135. PMID: 15959762.


23. Tham DM, Martin-McNulty B, Wang YX, Wilson DW, Vergona R, Sullivan ME, Dole W, Rutledge JC. Angiotensin II is associated with activation of NF-κB-mediated genes and downregulation of PPARs. Physiol Genomics. 2002; 11(1):21–30. PMID: 12361987.


24. Theuer J, Dechend R, Muller DN, Park JK, Fiebeler A, Barta P, Ganten D, Haller H, Dietz R, Luft FC. Angiotensin II induced inflammation in the kidney and in the heart of double transgenic rats. BMC Cardiovasc Disord. 2002; 2:3. PMID: 11835691.


25. Gemici B, Tan R, Ongut G, Izgüt-Uysal VN. Expressions of inducible nitric oxide synthase and cyclooxygenase-2 in gastric ischemia-reperfusion: role of angiotensin II. J Surg Res. 2010; 161(1):126–133. PMID: 19959193.


26. Yao R, Cheng X, Chen Y, Xie JJ, Yu X, Liao MY, Ding YJ, Tang TT, Liao YH. Molecular mechanisms of irbesartan suppressing atherosclerosis in high cholesterol-diet apolipoprotein E knock-out mice. Int J Cardiol. 2010; 139(2):113–122. PMID: 19028402.
27. Ohishi M, Dusting GJ, Fennessy PA, Mendelsohn FA, Li XC, Zhuo JL. Increased expression and co-localization of ACE, angiotensin II AT(1) receptors and inducible nitric oxide synthase in atherosclerotic human coronary arteries. Int J Physiol Pathophysiol Pharmacol. 2010; 2(2):111–124. PMID: 21179388.
28. Cooper R, Morré DJ, Morré DM. Medicinal benefits of green tea: Part 1. Review of noncancer health benefits. J Altern Complement Med. 2005; 11:521–528. PMID: 15992239.
29. Ramesh E, Geraldine P, Thomas PA. Regulatory effect of epigallocatechin gallate on the expression of C-reactive protein and other inflammatory markers in an experimental model of atherosclerosis. Chem Biol Interact. 2010; 183(1):125–132. PMID: 19782057.


30. Kim JS, Kim JM, O JJ, Jeon BS. Inhibition of inducible nitric oxide synthase expression and cell death by (-)-epigallocatechin-3-gallate, a green tea catechin, in the 1-methyl-4-phenyl-1,2,3,6-tetrahydropyridine mouse model of Parkinson's disease. J Clin Neurosci. 2010; 17(9):1165–1168. PMID: 20541420.


31. Peairs A, Dai R, Gan L, Shimp S, Rylander MN, Li L, Reilly CM. Epigallocatechin-3-gallate (EGCG) attenuates inflammation in MRL/lpr mouse mesangial cells. Cell Mol Immunol. 2010; 7(2):123–132. PMID: 20140007.


32. Syed DN, Afaq F, Kweon MH, Hadi N, Bhatia N, Spiegelman VS, Mukhtar H. Green tea polyphenol EGCG suppresses cigarette smoke condensate-induced NF-κB activation in normal human bronchial epithelial cells. Oncogene. 2007; 26(5):673–682. PMID: 16862172.


33. Harper CE, Patel BB, Wang J, Eltoum IA, Lamartiniere CA. Epigallocatechin-3-Gallate suppresses early stage, but not late stage prostate cancer in TRAMP mice: mechanisms of action. Prostate. 2007; 67(14):1576–1589. PMID: 17705241.


34. Zhang N, Weber A, Li B, Lyons R, Contag PR, Purchio AF, West DB. An inducible nitric oxide synthase-luciferase reporter system for in vivo testing of anti-inflammatory compounds in transgenic mice. J Immunol. 2003; 170(12):6307–6319. PMID: 12794164.


35. Chung EY, Kim BH, Hong JT, Lee CK, Ahn B, Nam SY, Han SB, Kim Y. Resveratrol down-regulates interferon-γ-inducible inflammatory genes in macrophages: molecular mechanism via decreased STAT-1 activation. J Nutr Biochem. 2010; in press.


36. Hoshino J, Park EJ, Kondratyuk TP, Marler L, Pezzuto JM, van Breemen RB, Mo S, Li Y, Cushman M. Selective synthesis and biological evaluation of sulfate-conjugated resveratrol metabolites. J Med Chem. 2010; 53(13):5033–5043. PMID: 20527891.


37. Bhaskar S, Shalini V, Helen A. Quercetin regulates oxidized LDL induced inflammatory changes in changes in human PBMCs by modulating the TLR-NF-κB signaling pathway. Immunobiology. 2011; 216(3):367–373. PMID: 20828867.
38. Wan LL, Xia J, Ye D, Liu J, Chen J, Wang G. Effects of quercetin on gene and protein expression of NOX and NOS after myocardial ischemia and reperfusion in rabbit. Cardiovasc Ther. 2009; 27(1):28–33. PMID: 19207477.


39. Shan Y, Zhao R, Geng W, Lin N, Wang X, Du X, Wang S. Protective effect of sulforaphane on human vascular endothelial cells against lipopolysaccharide-induced inflammatory damage. Cardiovasc Toxicol. 2010; 10(2):139–145. PMID: 20405237.


40. Yang WH, Fong YC, Lee CY, Jin TR, Tzen JT, Li TM, Tang CH. Epigallocatechin-3-gallate induces cell apoptosis of human chondrosarcoma cells through apoptosis signal-regulating kinase 1 pathway. J Cell Biochem. 2011; 112(6):1601–1611. PMID: 21328612.


41. Peng N, Liu JT, Guo F, Li R. Epigallocatechin-3-gallate inhibits interleukin-6- and angiotensin II-induced production of C-reactive protein in vascular smooth muscle cells. Life Sci. 2010; 86(11-12):410–415. PMID: 20100497.


Figure 1
Effect of angiotensin II (Ang II) treatment (100 nM, 0-24 h) on the expression levels of inducible nitric oxide synthase (iNOS) in human umbilical vein endothelial cells. Summary data are shown as the mean±SEM.
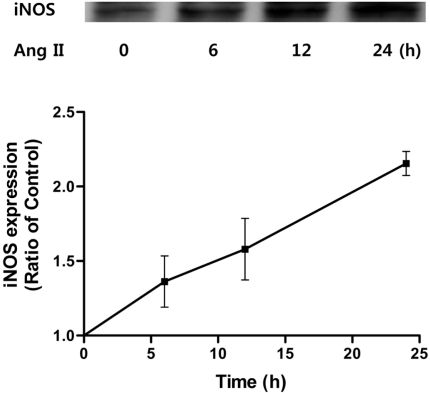