Abstract
Exercise training is highly correlated with the reduced glucose-stimulated insulin secretion (GSIS), although it enhanced insulin sensitivity, glucose uptake and glucose transporter expression to reduce severity of diabetic symptoms. This study investigated the impact of short-term swimming exercise on insulin regulation in the Goto-Kakizaki (GK) rat as a non-obese model of non-insulin-dependent diabetes mellitus. Wistar (W/S) and GK rats were trained 2 hours daily with the swimming exercise for 4 weeks, and then the changes in the metabolism of insulin and glucose were assessed. Body weight was markedly decreased in the exercised GK rats compare to their non-exercised counterpart, while W/S rats did not show any exercise-related changes. Glucose concentration was not changed by exercise, although impaired glucose tolerance was improved in GK rats 120 min after glucose injection. However, insulin concentration was decreased by swimming exercise as in the decrease of GSIS after running exercise. To identify the other cause for exercise-induced insulin down-regulation, the changes in the levels of key factors involved in insulin production (C-peptide) and clearance (insulin-degrading enzyme; IDE) were measured in W/S and GK rats. The C-peptide level was maintained while IDE expression increased markedly. Therefore, these results showed that insulin down-regulation induced by short-term swimming exercise likely attributes to enhanced insulin clearance via IDE over-expression than by altered insulin production.
Insulin is a small peptide (51 amino acids) hormone produced in the islets of Langerhans located in the pancreas (Chang et al, 1997). Insulin is crucial in the regulation of carbohydrate and fat metabolism in the body, by promoting the uptake of glucose and its storage as glycogen in the liver, muscles and fat cells (Duckworth and Kitabchi, 1981). Disruption of insulin's action preludes the development of various chronic diseases including diabetes mellitus (Sonksen and Sonken, 2000). When the glucose concentration is elevated from its normal physiological value, insulin release from the β cells is greatly increased. Once the glucose concentration returns to a normal level, the release of insulin slows or ceases (Sonksen and Sonken, 2000). The insulin released from β cells has a short plasma half-life, as would be expected from the necessity to respond rapidly to the changes in the blood glucose level (Duckworth, 1988; Morishima et al, 1992). Insulin degradation helps controlling the cellular response to the hormone by decreasing insulin availability, but the degradation process may also mediate some aspects of insulin actions (Akiyama et al, 1990; Duckworth et al, 1997, 1998). Liver and kidney are the two primary sites for insulin degradation. Most insulin is removed in the liver during the first transit, while most of the systematically circulating insulin is degraded in the kidney (Castillo et al, 1994; Canas et al, 1995). Degradation of the insulin normally involves endocytosis of the insulin-receptor complex followed by the action of insulin-degrading enzyme (IDE) (Duckworth et al, 1998). Until now, research concerning the development of therapeutic drugs for diabetes has targeted the regulation of insulin metabolism (Moghissi, 2008). However, this research approach has been hindered by lack of sufficient knowledge about the regulatory factors.
The treatment of diabetes mellitus has traditionally and predominantly centered on pharmacotherapy and dietetic therapy. However, the importance of exercise in the treatment of diabetes is being increasingly recognized. Exercise training as a repeated physical activity induces the persistent increase of insulin action on skeletal muscle from insulin-resistance and/or obese individuals (Hawley, 2004). This process is related to the alteration in the expression of various proteins involved in insulin signal transduction in skeletal muscle (Hawley, 2004; Hawley and Lessard, 2008). Furthermore, running exercise decreased the level of glucose-stimulated insulin, proinsulin and C-peptide (Mikines et al, 1989; Ueda et al, 2003). Especially, a regimen of swimming exercise can be important in the relief or even prevention of the symptoms of diabetes-related disease. In an alloxin-induced diabetic rat model, a 6-week swimming training program improved aerobic conditioning and the metabolism of both glucose and protein (de Oliveira et al, 2007). Also, a 10-week swimming training program led to a reduction in body weight and blood lactate concentration during early phase of acute exercise (Ribeiro Braga et al, 2004). However, the detailed mechanisms accounting for the effect of swimming exercise on glucose and insulin regulation remains unknown.
The present study was undertaken to investigate the effect of short-term swimming exercise on the regulation of insulin level in the Goto-Kakizaki (GK) rat model of type 2 diabetes. A short-term swimming exercise induced the decrease of the insulin concentration in both types of rats without the significant alteration of glucose concentration. Our results suggested the possibility that IDE over-expression could contribute the insulin down-regulation during swimming exercise.
Thirty two-week-old female GK rats were purchased from Samtaco (Osan, Korea) and quarantined in a specific room for 1 week. The same age of female Wistar (W/S) rats as the control were supplied from the breeding center of National Institute of Toxicological Research (Seoul, Korea). The rats were kept in a Korea Food and Drug Administration (KFDA, Seoul, Korea) animal facility accredited in accordance with the Association for Assessment and Accreditation of Laboratory Animal Care International policies. The rats had access to a standard irradiated chow diet (Purina Mills, St. Louis, MO, USA) and water ad libitum, and were maintained in a specific pathogen-free state under a strict light cycle (06:00-18:00).
All animal experimental procedures used in this study have been reviewed by the KFDA-Institutional Animal Care and Use Committee (KFDA-IACUC) on their ethical procedures and scientific care, and it has been approved. GK and W/S rats were randomly divided into two subgroups (n=6) per group. The first subgroup of GK and W/S rats received a comparable level of daily swimming exercise, while the second subgroup did not receive any exercise. The two-tier protocol for swimming exercise involved adaptation and training phases. On the first day of the adaptation phase, the animals were swum in the tank for 10 min. Thereafter, the exercise period was extended by 10 min each day. By the day 6, swimming exercise was 60 continuous min. After the adaptation phase, the exercise consisted of two swimming sessions of 60 min separated by a break of 15 min. This regimen was continued for 3 weeks (Figure 1). All exercise sessions were conducted in a circular plastic pool (1,962 cm2 in surface area and 50 cm deep). This pool was filled with water that was maintained at 30-34℃. Only three rats were present in the pool at any time to allow unimpeded space for free swimming.
Glucose tolerance tests were performed after the exercise period for 2 weeks. Glucose tolerance was determined in rats using an intraperitoneal glucose tolerance test (IPGTT; 1.5 g glucose/kg) after a 24 h fast. Blood glucose measurements were made at 0, 15, 30, 60, 90, and 120 min (Hwang et al, 2007). The glucose level of whole blood collected from tail was measured with the sensitive strip method using a Blood Glucose Monitoring System (I-sens, Seoul, Korea).
Following the final exercise application, the rats were fasted for 24 h, after which whole blood was collected from their abdominal vein during anesthesia. Serum was obtained by centrifuging the blood (15,000 rpm, 4℃, 10 min), followed by incubation for 30 min at room temperature. The serum was then stored at -80℃ until analysis. The concentrations of insulin and C-peptide in serum from W/S and GK rats were detected using a solid phase two-site enzyme immunoassay procedure and reagents (Rat Insulin ELISA kit and Rat C-peptide ELISA kit, Mercodia, Uppsala, Sweden). The ELISA was based on the direct sandwich technique, in which two monoclonal antibodies are directed against separate epitopes on the insulin molecule. Each serum sample and an enzyme conjugate solution were added to appropriate wells of a microplate and incubated on a plate shaker (700-900 rpm) for 1 h at room temperature. The unbound enzyme-labeled antibody and other molecules were removed by a washing step using a solution comprised of 50 mM Tris, 0.14 M NaCl and 0.05% Tween-20 (pH 8.0). The bound conjugate was detected by reaction with 3,3',5,5'-tetramethylbenzidine in a shaker for 30 min at room temperature. The reaction was stopped by adding 0.5 M H2SO4 to give a colorimetric endpoint that was read spectrophotometrically at 450 nm using a Vmax plate reader (Molecular Devices, Sunnyvale, CA, USA).
The proteins prepared from the liver tissues of W/S and GK rats were resolved by 4-20% sodium dodecyl sulfate-polyacrylamide gel electrophoresis for 2 h. The proteins were transferred to a nitrocellulose membrane for 2 h at 40 V. The membrane was incubated with primary antibody-either anti-IDE (Calbiochem, San Diego, CA, USA) or anti-actin (Sigma-Aldrich, St. Louis, MO, USA)-overnight at 4℃. The membrane was washed with a washing buffer (137 mM NaCl, 2.7 mM KCl, 10 mM Na2HPO4, 2 mM KH2PO4, and 0.05% Tween-20) and incubated with a 1:2,000 dilution of horseradish peroxidase-conjugated goat anti-rabbit IgG (Zymed, Carlsbad, CA, USA) at room temperature for 2 h. The membrane blots were developed using an enhanced chemiluminescence Reagent Plus kit (Pharmacia, Uppsala, Sweden).
One-way ANOVA was used to determine if significant differences existed between the non-exercise group and exercise group (SPSS for Windows, Release 10.10, Standard Version; SPSS, Chicago, IL, USA). In addition, differences in the responses of the W/S and GK rats were evaluated using a post-hoc test of the variance and significance levels using the same software. All values were reported as the mean±SD. A P<0.05 was considered significant.
To detect the exercise effects on the body weight, the rats were weighed once after the exercise period for 3 weeks. The body weights of GK rats were significantly reduced in the exercise group compare to non-exercise group, while exercise had no effect on body weight in W/S rats (Figure 2).
Swimming exercise improves glucose and protein metabolism of diabetic rats (de Oliveira et al, 2007). To investigate the effect of short-term swimming exercise on the glucose tolerance response after last exercise, glucose concentration was measured in blood samples obtained at selected intervals after the injection of glucose. At 0 min, 32-week-old GK rats displayed a high level of glucose, compared with W/S rats. But, there is no difference between non-exercise and exercise groups on glucose concentration. After glucose injection, the glucose level rapidly increased in both rats. The maximum level of glucose was significantly higher in GK rats than in W/S rats. With time, the glucose level in W/S rats rapidly returned to the basal level regardless of exercise (Figure 3A). However, in the non-exercise group of GK rats, the glucose level gradually increased from 30-120 min after glucose injection and reached the maximum level at 120 min. In the exercised GK rats, the glucose level was slightly decreased from 15-120 min following injection. But, the level at 120 min did not decrease to basal level, and remained 55% higher than the level at 0 min (Figure 3B). The results are consistent with a significantly impaired glucose tolerance in the GK rats, which could be lessened by short-term swimming exercise.
To study the effect of short-term swimming exercise on the insulin concentration in the serum of GK rats, the insulin concentration in the serum collected from blood was measured using a commercial ELISA system that utilized anti-insulin antibody. Non-exercised GK rats presented slightly higher of insulin concentration (23%) than non-exercised W/S rats. After exercise, the insulin concentration was markedly decreased in both types of rats, but the trend of slightly higher insulin level in GK rats was maintained in the exercise groups (Figure 4). The results were consistent with the view that short-term swimming exercise could stimulate insulin down-regulation, as evident in the blood serum of W/S and GK rats, but to differing degrees.
Glucose-stimulated insulin secretion (GSIS) was considered as main factor decreasing in normal young men and rats after exercise training (Ueda et al, 2003). However, the aforementioned results indicated that the insulin concentration was significantly decreased regardless of glucose concentration. Therefore, to explore potential mechanisms for the decreased insulin concentration after short-term swimming exercise, the key factors of insulin production and degradation were detected in both types of rats because insulin concentration is tightly regulated by the production and clearance process in difference organs (Valera Mora et al, 2003). Also, it is well accepted that the level of insulin production was indirectly detected as the measurement of C-peptide level (Marques et al, 2004). The level of C-peptide in both types of rat was very similar regardless of exercise status (Figure 5). The results suggest that short-term swimming exercise does not significantly affect the production of insulin from pancreatic β cells. To investigate whether insulin degradation could cause insulin down-regulation, the expression level of IDE protein, which is a key protein for insulin clearance in the liver, was determined by Western blot assay. The expression level of IDE protein was significantly increased in the exercise group compare to the non-exercise group (Figure 6). These results indicated that the swimming exercise could up-regulate IDE expression, thereby reducing the insulin concentration.
Regular exercise including walking, jogging or swimming is beneficial in disease control. Of the various types of exercise, swimming exercise appears useful for treating metabolic diseases related to obesity and diabetes (Buemann and Tremblay, 1996; Enevoldsen et al, 2000). In a 6-week-long study of db/db mice, this training significantly decreased the concentration of serum free fatty acid, cholesterol and triglycerides, and was associated with gains in body weight and adipose tissue mass in obese and lean mice (Oh et al, 2007). Also, another study in which W/S rats were injected with monosodium glutamate (MSG) reported the reduction of blood lactate accumulation and body weight during acute exercise (de Souza et al, 2003; Ribeiro et al, 2004). In this study, GK and W/S rats were trained for swimming exercise in a plastic circular pool for 3 weeks. After exercise, the body weight was significantly decreased only in GK rats. The discrepancy in the findings with W/S rats in the previous and present studies needs to be reconciled.
The study used a GK rat model of non-insulin-dependent diabetes mellitus (i.e., type 2 diabetes) without obesity. The model was originally produced by repeated selective breeding of rats with glucose intolerance starting from a non-diabetic W/S rat colony (Goto et al, 1975; Suzuki et al, 1992). After the onset of diabetes, the GK rats display fasting hyperglycemia, impaired secretion of insulin in response to glucose, and hepatic and peripheral insulin resistance. The model is considered as the best to test drug efficacy, because the phenotypes, which include metabolic, hormonal and vascular disorders, are very reminiscent of human diabetes (Ostenson et al, 1993). Presently, 8-month-old GK rats, which displayed impaired glucose tolerance and altered insulin concentration compared to control W/S rats, were used to evaluate the effect of a short-term swimming exercise program on insulin regulation.
Blood glucose levels can be exercise-regulated through three different mechanisms: acute stimulation of muscle glucose transport, acute increase of insulin action and long term up-regulation of the insulin signaling pathway (Choi and Kim, 2010). Previous studies have employed daily swimming for different periods. In one study involving 13-week-old Zucker diabetic rats, glucose tolerance normally always deteriorated, while in rats that were engaged in a 13-week swimming exercise regimen the 2 h post-glucose tolerance was significantly improved (Kiraly et al, 2008). In a hypothalamic obesity model induced by administration of monosodium glutamate, chronic aerobic exercise (swimming) improved glucose tolerance and reduced insulin resistance (de Mello et al, 2001). But, until now, a similar study had not been conducted using GK rats. Consistent with those of the aforementioned studies, GK rats exercised for only 4 weeks displayed significant improvements at 120 min after glucose administration, although glucose concentration did not completely recover to the basal level. Furthermore, exercise enhances glucose utilization, and thereby glucose concentration is significantly decreased in blood (Ochiai and Matsuo, 2009). Simultaneously, increases in glucose uptake and glucose transpoter expression were observed in mammalian species (Ueda et al, 2003). In our study, the glucose concentration was lower in exercise group than non-exercise group of W/S and GK rats, although rate of decrease was different between two rat species. These results indicated that swimming exercise could effectively enhance the glucose utilization, although more comparison research on the effects of various exercises.
Studies using animal models have demonstrated the effects of exercise on insulin concentration. The increase of insulin concentration and β cell mass was observed in Zucker diabetic rats in a 13-week swimming exercise program. However, at the 4-week point of the study the insulin concentration temporarily decreased compared to the non-exercised rats, thereafter steadily increasing to week 13 (Kiraly et al, 2008). Also, another study involving the same breed reported that swimming exercise for 4 weeks produced a considerable decrease in insulin concentration in lean Zucker rats, but only a slight decrease in obese Zucker rats (Kibenge and Chan, 2002). There are several factors that could be considered as causes of insulin reduction. Of these, the decrease of GSIS was a well-known major factor that induced the decline of insulin concentration in young men and rats after exercise training (Zawalich et al, 1982; Mikines et al, 1989; Koranyi et al, 1991). However, in the present study, 4 weeks of swimming exercise decreased insulin concentration in both W/S and GK rats, with the reduction being greater in W/S rats without significant change of glucose concentration. Therefore, this study was needed to find the novel factors for insulin regulation during swimming exercise.
Mechanistically, two possibilities can be suggested: a decrease in insulin production and an increase in insulin clearance. Decreased insulin production is indicated by altered C-peptide concentration. In all mammals, the processing of proinsulin occurs within β cells to yield the insulin and C-peptide, which are then secreted in equimolar quantities into the blood circulation (Marques et al, 2004). Measurement of C-peptide in blood may provide valuable information about insulin production in pancreatic β cells. As shown in Figure 5, the concentration of C-peptide was unaffected by swimming exercise in the W/S and GK rats, suggesting that the decrease of insulin concentration was unaffected by the insulin production of pancreatic β cells. In the present study, the possibility of increased insulin clearance was examined by the detection of IDE expression. IDE is associated with the primary mechanism of insulin degradation, although other systems, including protein disulfide isomerase and lysozyme or other enzymes, undoubtedly contribute to the metabolism of insulin (Duckworth et al, 1998). Also, IDE is broadly expressed in both insulin-sensitive and insulin-insensitive tissues, and it is primarily localized in the cell cytoplasm (Seta and Roth, 1997; Vekrellis et al, 2000). IDE in the various tissues could bind and degrade several high-affinity substrates (polypeptides 28-51 amino acids in length), which include a variety of physiological peptides such as insulin, glucagons (Kirschner and Goldberg, 1983), atrial natriuretic peptide (Muller et al, 1991), transforming growth factor α (Garcia et al, 1989; Gehm et al, 1991), atrial natriuretic peptide (Muller et al, 1992), β-endorphin and dynorphins (Safavi et al, 1996), amylin (Bennett et al, 2002), and amyloid β peptides (Mukherjee et al, 2000; Chesneau et al, 2002). Presently, measurements of the expression level of IDE in the liver of W/S and GK rats revealed a marked increased in the exercised group (Figure 6).
The present collective results indicate that the IDE overexpression was consider as a factor of insulin down-regulation during short-term swimming exercise, although this exercise can effectively improve the insulin sensitivity.
Acknowledgments
We thank S.M. Choi and M.K. Jang, the animal technicians who directed the Animal Facility and Care at the Division of Laboratory Animal Resources. This research was supported by grants from the Korea Food and Drug Administration.
References
1. Akiyama H, Yokono K, Shii K, Ogawa W, Taniguchi H, Baba S, Kasuga M. Natural regulatory mechanisms of insulin degradation by insulin degrading enzyme. Biochem Biophys Res Commun. 1990; 170:1325–1330. PMID: 2202301.


2. Bennett RG, Duckworth WC, Hamel FG. Degradation of amylin by insulin-degrading enzyme. J Biol Chem. 2000; 275:36621–36625. PMID: 10973971.


3. Buemann B, Tremblay A. Effects of exercise training on abdominal obesity and related metabolic complications. Sports Med. 1996; 21:191–212. PMID: 8776009.


4. Canas X, Fernandez-Lopez JA, Ardevol A, Adan C, Esteve M, Rafecas I, Remesar X, Alemany M. Rat insulin turnover in vivo. Endocrinology. 1995; 136:3871–3876. PMID: 7649094.
5. Castillo MJ, Scheen AJ, Letiexhe MR, Lefebvre PJ. How to measure insulin clearance. Diabetes Metab Rev. 1994; 10:119–150. PMID: 7956676.


6. Chang X, Jorgensen AM, Bardrum P, Led JJ. Solution structures of the R6 human insulin hexamer. Biochemistry. 1997; 36:9409–9422. PMID: 9235985.
7. Chesneau V, Vekrellis K, Rosner MR, Selkoe DJ. Purified recombinant insulin-degrading enzyme degrades amyloid beta-protein but does not promote its oligomerization. Biochem J. 2000; 351:509–516. PMID: 11023838.
8. Choi K, Kim YB. Molecular mechanism of insulin resistance in obesity and type 2 diabetes. Korean J Intern Med. 2010; 25(2):119–129. PMID: 20526383.


9. de Mello MA, de Souza CT, Braga LR, dos Santos JW, Ribeiro IA, Gobatto CA. Glucose tolerance and insulin action in monosodium glutamate (MSG) obese exercise-trained rats. Physiol Chem Phys Med NMR. 2001; 33:63–71. PMID: 11758736.
10. de Oliveira CA, Luciano E, Marcondes MC, de Mello MA. Effects of swimming training at the intensity equivalent to aerobic/anaerobic metabolic transition in alloxan diabetic rats. J Diabetes Complicat. 2007; 21:258–264. PMID: 17616357.


11. de Souza CT, Nunes WM, Gobatto CA, de Mello MA. Insulin secretion in monosodium glutamate (MSG) obese rats submitted to aerobic exercise training. Physiol Chem Phys Med NMR. 2003; 35:43–53. PMID: 15139282.
12. Duckworth WC, Bennett RG, Hamel FG. Insulin degradation: progress and potential. Endocr Rev. 1998; 19:608–624. PMID: 9793760.


13. Duckworth WC, Bennett RG, Hamel FG. The significance of intracellular insulin to insulin action. J Investig Med. 1997; 45:20–27.
14. Duckworth WC. Insulin degradation: mechanisms, products, and significance. Endocr Rev. 1988; 9:319–345. PMID: 3061785.


15. Duckworth WC, Kitabchi AE. Insulin metabolism and degradation. Endocr Rev. 1981; 2:210–233. PMID: 7028472.


16. Enevoldsen LH, Stalknecht B, Fluckey JD, Galbo H. Effect of exercise training on in vivo insulin-stimulated glucose uptake in intra-abdominal adipose tissue in rats. Am J Physiol Endocrinol Metab. 2000; 278:E25–E34. PMID: 10644533.


17. Garcia JV, Gehm BD, Rosener MR. An evolutionarily conserved enzyme degrades transforming growth factor-alpha as well as insulin. J Cell Biol. 1989; 109:1301–1307. PMID: 2670957.


18. Gehm BD, Rosner MR. Regulation of insulin, epidermal growth factor, and transforming growth factor-alpha levels by growth factor-degrading enzymes. Endocrinology. 1991; 128:1603–1610. PMID: 1847863.
19. Goto Y, Kakizaki M, Masaki N. Spontaneous diabetes produced by selective breeding of normal Wistar rats. Proc Jpn Acad. 1975; 51:80–85.


20. Hawley JA. Exercise as a therapeutic intervention for the prevention and treatment of insulin resistance. Diabetes Metab Res Rev. 2004; 20:383–393. PMID: 15343584.


21. Hawley JA, Lessard SJ. Exercise training-induced improvements in insulin action. Acta Physiol (Oxf). 2008; 192:127–135. PMID: 18171435.


22. Hwang DY, Seo SJ, Kim YK, Kim CK, Shim SB, Jee SW, Lee SH, Sin JS, Cho JY, Kang BC, Jang IS, Cho JS. Significant change in insulin production, glucose tolerance and ER stress signaling in transgenic mice coexpressing insulin-siRNA and human IDE. Int J Mol Med. 2007; 19:65–73. PMID: 17143549.


23. Kibenge MT, Chan CB. The effects of high-fat diet on exercise-induced changes in metabolic parameters in Zucker fa/fa rats. Metabolism. 2002; 51:708–715. PMID: 12037723.


24. Király MA, Bates HE, Kaniuk NA, Yue JT, Brumell JH, Matthews SG, Riddell MC, Vranic M. Swim training prevents hyperglycemia in ZDF rats: mechanisms involved in the partial maintenance of beta-cell function. Am J Physiol Endocrinol Metab. 2008; 294:E271–E283. PMID: 18029442.
25. Kirschner RJ, Goldberg AL. A high molecular weight metalloendopeptidase from the cytosol of mammalian cells. J Biol Chem. 1983; 258:967–976. PMID: 6401723.
26. Koranyi LI, Bourey RE, Slentz CA, Holloszy JO, Permutt MA. Coordinate reduction of rat pancreatic islet glucokinase and proinsulin mRNA by exercise training. Diabetes. 1991; 40(3):401–404. PMID: 1705526.


27. Marques RG, Fontaine MJ, Rogers J. C-peptide: much more than a byproduct of insulin biosynthesis. Pancreas. 2004; 29:231–238. PMID: 15367890.
28. Mikines KJ, Sonne B, Tronier B, Galbo H. Effects of training and detraining on dose-response relationship between glucose and insulin secretion. Am J Physiol. 1989; 256:E588–E596. PMID: 2655469.


29. Moghissi ES. Insulin strategies for managing inpatient and outpatient hyperglycemia and diabetes. Mt Sinai J Med. 2008; 75:558–566. PMID: 19021195.


30. Morishima T, Pye S, Bradshaw C, Radziuk J. Posthepatic rate of appearance of insulin: measurement and validation in the nonsteady state. Am J Physiol. 1992; 263:E772–E779. PMID: 1415699.


31. Mukherjee A, Song E, Kihiko-Ehmann M, Goodman JP Jr, Pyrek JS, Estus S, Hersh LB. Insulysin hydrolyzes amyloid beta peptides to products that are neither neurotoxic nor deposit on amyloid plaques. J Neurosci. 2000; 20:8745–8749. PMID: 11102481.
32. Muller D, Baumeister H, Buck F, Richter D. Atrial natriuetic peptide (ANP) is a high-affinity substrate for rat insulin-degrading enzyme. Eur J Biochem. 1991; 202:285–292. PMID: 1836994.
33. Muller D, Schulze C, Baumeister H, Buck F, Richter D. Rat insulin-degrading enzyme: cleavage pattern of the natriuretic peptide hormones ANP, BNP, and CNP revealed by HPLC and mass spectrometery. Biochemistry. 1992; 31:11138–11143. PMID: 1445854.
34. Ochiai M, Matsuo T. Effects of Short-Term Dietary Change from High-Carbohydrate Diet to High-Fat Diet on Storage, Utilization, and Fatty Acid Composition of Rat Muscle Triglyceride during Swimming Exercise. J Clin Biochem Nutr. 2009; 44(2):168–177. PMID: 19308271.


35. Oh KS, Kim EY, Yoon M, Lee CM. Swim training improves leptin receptor deficiency-induced obesity and lipid disorder by activating uncoupling proteins. Exp Mol Med. 2007; 39:385–394. PMID: 17603293.


36. Ostenson CG, Khan A, Abdel-Halim AM, Guenifi A, Suzuki K, Goto Y, Efendic S. Abnormal insulin secretion and glucose metabolism in pancreatic islets from the spontaneously diabetic GK rat. Diabetologia. 1993; 36:3–8. PMID: 8436249.


37. Ribeiro Braga L, de Mello MA, Gobatto CA. Continuous and intermittent exercise: effects of training and detraining on body fat in obese rats. Arch Latinoam Nutr. 2004; 54:58–65. PMID: 15332357.
38. Safavi A, Miller BC, Cottam L, Hersh LB. Identification of gamma-endorphin-generating enzyme as insulin-degrading enzyme. Biochemistry. 1996; 35:14318–14325. PMID: 8916918.
39. Seta KA, Roth RA. Overexpression of insulin degrading enzyme: cellular localization and effects on insulin signaling. Biochem Biophys Res Commun. 1997; 231:167–171. PMID: 9070242.


40. Sonksen P, Sonksen J. Insulin: understanding its action in health and disease. Br J Anaesth. 2000; 85:69–79. PMID: 10927996.


41. Suzuki KI, Goto Y, Toyota T. Shafrir E, editor. Spntaneously diabetic GK (Goto-Kakizaki) rats. Lessons from Animal Diabetes. 1992. 1st ed. London: Smith-Gordon;p. 107–116.
42. Ueda H, Urano Y, Sakurai T, Kizaki T, Hitomi Y, Ohno H, Izawa T. Enhanced expression of neuronal nitric oxide synthase in islets of exercise-trained rats. Biochem Biophys Res Commun. 2003; 312(3):794–800. PMID: 14680835.


43. Valera Mora ME, Scarfone A, Calvani M, Greco AV, Mingrone G. Insulin clearance in obesity. J Am Coll Nutr. 2003; 22:487–493. PMID: 14684753.


44. Vekrellis K, Ye Z, Qiu WQ, Walsh D, Hartley D, Chesneau V, Rosner MR, Selkoe DJ. Neurons regulate extracellular levels of amyloid beta-protein via proteolysis by insulin-degrading enzyme. J Neurosci. 2000; 20:1657–1665. PMID: 10684867.
45. Zawalich W, Maturo S, Felig P. Influence of physical training on insulin release and glucose utilization by islet cells and liver glucokinase activity in the rat. Am J Physiol. 1982; 243(6):E464–E469. PMID: 6756163.


Figure 1
Scheme of short-term swimming exercise during 3 weeks. A. In the adaptation phase, the swimming time was extended by 10 min every day until reaching 60 min after 6 days. B. In the training phase, all animals were trained for 2 h per day. After swimming exercise, the wet body of rat was rapidly dried with a Kimtowel to maintain body temperature.
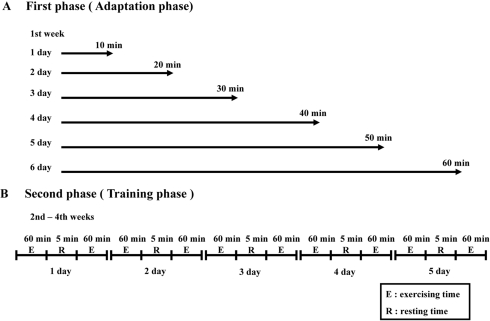
Figure 2
Effect of short-term swimming exercise on body weight. Six rats per group were used to measure body weights. The data represents the mean±SD from triplicates. *P<0.05; significant difference between W/S and GK rats. **P<0.05; significant difference between non-exercise group and exercise group in each rat type.
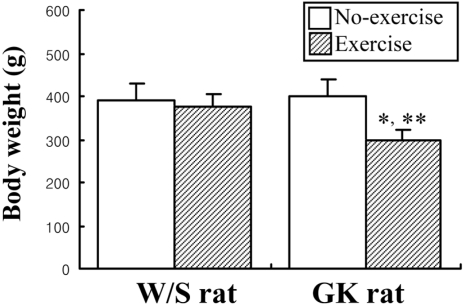
Figure 3
Post-prandial intraperitoneal glucose tolerance response in W/S (A) and GK (B) rats. Glucose was intraperitoneally injected (1.5 g/kg body weight) and blood glucose was determined at indicated intervals. Four or five rats per group were assayed in the IPGT test. The data represents the mean±SD from triplicates. *P<0.05; significant difference between W/S and GK rats.
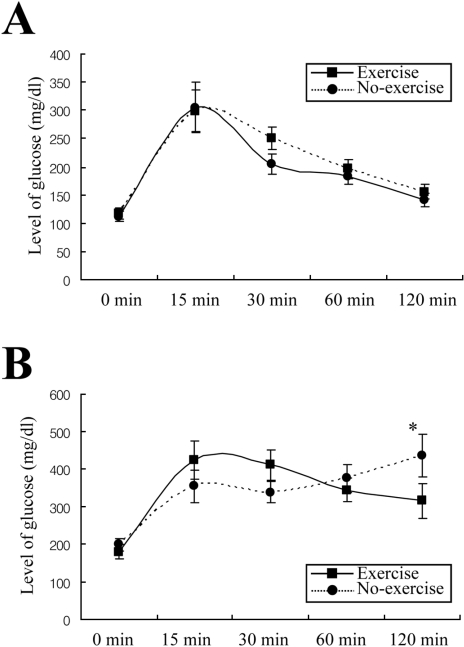
Figure 4
Effect of short-term swimming exercise on serum insulin concentration. Serum was harvested from the blood sample collected from the abdominal veins of W/S and GK rats. The insulin level was determined using ELISA. The data represents the mean±SD from triplicates. *P<0.05; significant difference between W/S and GK rats. **P<0.05; significant difference between non-exercise group and exercise group per rat type.
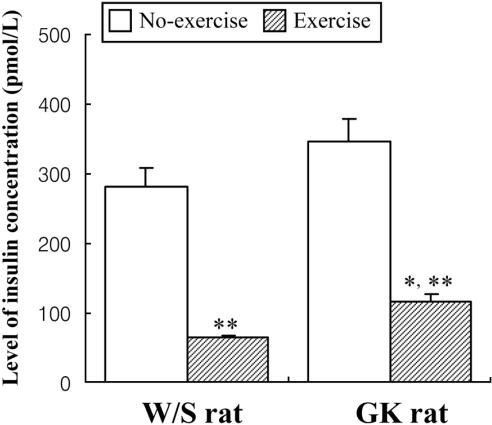
Figure 5
Effect of short-term swimming exercise on the serum C-peptide concentration. Serum was harvested from the blood samples collected from the abdominal veins of the W/S and GK rats. C-peptide level was determined using a C-peptide ELISA kit. The data represents the mean±SD from triplicates.
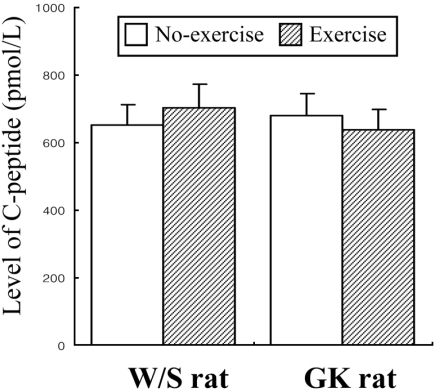
Figure 6
Effect of short-term swimming exercise on insulin-degrading enzyme (IDE) expression. IDE expression in the liver was detected with anti-IDE primary antibody and horseradish peroxidase-conjugated goat anti-rabbit IgG as described in Materials and Methods. The densitometric intensity of the IDE protein band was determined. The data represents the mean±SD from triplicates. *P<0.05; significant difference between W/S and GK rats.
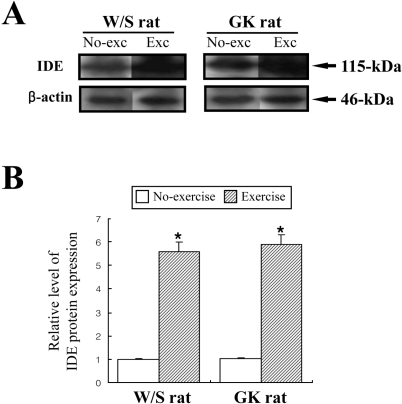