Abstract
Embryonic stem cells (ESCs) are an emerging source for cell-based therapies aimed at repairing damaged organ tissues; however, the efficiency of directed differentiation is low and refinement of differentiation protocols is hampered by incomplete understanding of the mechanisms involved in this process. To find new compounds which can improve the efficiency of directed differentiation of ESCs to cardiomyocytes, we screened several thousand chemical compounds and identified a promising group. All of the compounds found have a common structure of 1H-pyrrole,2,2'-(phenylmethylene)bis. Here we report the potential mechanism of action for 31002 which showed the strongest activity among the compounds selected. In the presence of 31002, 15 times more cardiomyocytes differentiated from ESCs, i.e., 3.5% to 52% of total differentiated cells. Moreover, the cardiomyocytes showed functional characteristics including rhythmic beating and marker gene expression. 31002 inhibited the down-regulation of genes related to the three germ layers in the late stage of ESCs differentiation, implying that 31002 supports a continuous fate commitment of undifferentiated ESCs to the cardiac lineage by prolonging the three germ layer stages. Therefore, compounds in this group, including 31002, might be useful as directed cardiomyogenic differentiation-inducers to produce cells for use in cell therapy aimed at restoring damaged heart tissue.
Chronic heart failure is currently incurable and a leading cause of death worldwide [1,2]. Myocardial infarction contributes to chronic heart failure by inducing myocardial cell death [3]. At present the only effective treatment for patients with chronic heart failure is donor or artificial heart transplantation [4]. Therefore, finding new therapeutic approaches is critical. Recent progress in stem cell science is providing potential therapeutic options for heart disease patients [2,5].
Most tissues have endogenous stem/progenitor cells, which upon injury to the organ, can proliferate and differentiate at the injury site to repair damaged tissue [6-9]. Although the adult heart is composed of postmitotic and terminally differentiated cells, there is also a small subpopulation of myocardial cells with cardiac stem cell characteristics [10,11]; however, their limited availability prevents them from being used in therapeutic applications.
In contrast, embryonic stem cells (ESCs) are available in considerable numbers and have become strong candidates as a cell source for cardiac repair [12,13]. ESCs are unspecialized precursor cells with the capability to self-renew and differentiate into specialized cells in response to appropriate signals [14].There are several small molecules known to have enhancing effects for cardiomyogenic differentiation of ESCs including retinoic acid [15], ascorbic acid [16], dimethyl sulfoxide (DMSO) [17], and oxytocin [17]. Although these compounds can support directed differentiation of ESCs to cardiomyocytes, the efficiency is insufficient for therapeutic applications. Therefore, new molecules with improved efficiency would have a great impact on the preparation of cardiomyocytes for clinical applications. To identify those molecules, we used a cell-based screening system with ESCs stably transfected with a vector carrying a cardiac specific α-myosin heavy chain (MHC) gene promoter-driven enhanced green fluorescent protein (EGFP) [18]. We successfully identified a group of compounds which acted as directed cardiomyogenic stimulants. Here we report the activity and possible mechanism of action of one such compound.
The 129 strain-derived KH2 ESCs which were stably transfected with an α-MHC promoter-driven EGFP expression vector, were previously described [18]. The cells were grown on irradiated mouse embryonic fibroblast feeder layers in high glucose Dulbecco's modified Eagle medium (DMEM; Gibco/BRL, Grand Island, NY, USA) supplemented with 15% ES-qualified fetal bovine serum (FBS; Hyclone, South Logan, UT, USA), 2 mM L-glutamine (Gibco/BRL), and 10 µM β-mercaptoethanol (Sigma-Aldrich, St. Louis, MO, USA) at 37℃ in 5% CO2. The ESCs were subcultured every two days.
Undifferentiated KH2 cells were trypsinized and suspended at a concentration of 3.2×104 cells/mL in differentiation medium [ESC culture medium with 20% FBS and 0.1 mM non-essential amino acids (Gibco/BRL)] on differentiation day (DD) 0. To form embryoid bodies (EBs), 25 µL-drops of cell suspension (800 cells/drop) were incubated in a 5% CO2 incubator as hanging drops for three days. EBs were collected on DD3 and three EBs were placed in each well of a 96-well plate and cultured for 12 days with differentiation medium. The differentiation medium was changed every 1-2 days during the culture period. Differentiated cells on DD15 were trypsinized, fixed with 1% formalin in phosphate-buffered saline (PBS), and subjected to flow cytometric analysis (FACScalibur; Becton-Dickinson, San Jose, CA, USA) for detection of EGFP-expressing cells.
Test compounds prepared in DMSO were obtained from the Korea Chemical Bank (Daejeon, Korea). The compounds were diluted with differentiation medium [1/160-1/1,000 final dilutions (equal to 1.6-5 µM of final concentration) depending on the provided concentrations]. For the first and second round of screening, test compounds were added to each well of a 96-well plate including three EBs of KH2 cells from DD3 to DD15. The differentiation medium including the test compound was changed every 1 or 2 days. Differentiated cells on DD15 were subjected to fluorescence activated cell sorter (FACS) analysis as described previously.
Total RNA from cells at different differentiating stages was extracted using RNeasy Kits (Qiagen, Hilden, Germany), and cDNA was synthesized from the total RNA with SuperScript II reverse transcriptase (Invitrogen, Carlsbad, CA, USA) according to the manufacturers' instructions. PCR amplification of the murine genes was carried out as described in Table 1. Gene expression levels were normalized to the β-actin level. At least three independent experiments were performed.
To confirm that the EGFP-expressing cells were cardiomyocytes, marker protein expression for cardiomyocytes was demonstrated by immunocytochemistry. In brief, EBs on DD3 were transferred on a coverslip coated with 0.1% gelatin and cultured with medium including 10 mM 31002 until DD15. Differentiated cells on DD15 were fixed in neutral 10% formalin for 10 min, washed three times with PBS, and blocked with PBS containing 0.3% bovine serum albumin (BSA) and 1% goat serum at room temperature for 40 min. The cells were incubated with anti-α-actinin (EA-53, Sigma, St. Louis, MO, USA) or anti-troponin T (Santa Cruz Biotechnology, Santa Cruz, CA, USA) monoclonal antibodies at a dilution of 1:800 or 1:200, respectively, at room temperature for 1 h. After washing with PBS, rhodamine-labeled goat anti-mouse IgG (KPL, Gaithersburg, MD, USA) was applied at room temperature for 1 h. After a brief incubation with 4',6-diamidino-2-phenylindole (DAPI; KPL) for nuclear staining, the cells were observed with a fluorescence microscope (BX62, Olympus, Tokyo, Japan).
We obtained 6,480 structurally representative compounds from the Korea Chemical Bank. The compounds were screened for their ability to enhance the appearance of EGFP-expressing cells from KH2 ESCs after differentiation for 15 days as described in Methods. The screening identified one active compound. Subsequently, 50 structural derivatives of the active compound containing a common chemical structure of 1H-pyrrole,2,2'-(phenylmethylene)bis were also subjected to screening. Of those, 12 showed outstanding activity (Figures 1 and 2). Two compounds, 30984 and 31002, had the highest activity and showed dose-dependent activity. Based on availability, we selected 31002 for further characterization.
The EGFP-expressing cells derived from KH2 ESCs in the presence of 31002 were easily detected by flow cytometry (Figure 3A).
To confirm that the EGFP-expressing KH2 cells had characteristics of cardiac cells, we performed immunofluorescence staining for the cardiac marker proteins α-actinin and troponin T (Figure 3B). EBs cultured on coverslips with or without 31002 were stained for the markers as described in Methods. As expected, almost all EGFP-expressing cells co-expressed troponin T and α-actinin, irrespective of the presence of 31002. Notably, EGFP-positive cell clumps differentiated with 31002 were much larger than those of the vehicle control. Furthermore, EGFP-expressing cell clumps could be identified within all the spontaneous beating areas in EBs when observed with a fluorescence microscope prior to fixation (data not shown). These results suggest that EGFP-expressing cells derived from KH2 ESCs in the presence of 31002 are functional cardiomyocytes.
When KH2 ESCs were differentiated with 31002, the EGFP-expressing cell clumps were hardly observed until DD8, after which, an abrupt increase in the cell ratio was observed between DD8 and DD12 (Figure 4). The increase reached a peak of 52% at DD17 and remained at that level with a small fluctuation until DD34. In contrast, the ratio in vehicle controls remained under 4% during the entire experimental period.
To determine the critical time point of 31002 action in cardiomyogenic differentiation of ESCs, seven experimental cell groups were treated with 31002 at different time points for three days. Cells in all groups were analyzed for EGFP positivity by flow cytometry on DD15 (Figure 5). Interestingly, group 7 which was treated with 31002 only for the last 3 days, showed a comparable EGFP-positive cell ratio to those in groups 1 and 2 in which 31002 was applied for 12 and 15 days, respectively. An intermediate EGFP-positive cell ratio was observed in group 6 which was treated with 31002 for three days between DD9 and DD12. However, there was no detectable 31002 effect in any groups in which a three-day treatment was applied between DD0 and DD9.
To obtain information related to the mechanism of action of 31002 in ESC differentiation, time-dependent expression patterns of developmental marker genes were analyzed in the cells at different stage of differentiation. KH2 cells were differentiated for 15 days as described in Materials and Methods in the presence of 10 µM 31002 or vehicle (0.1% DMSO). The expression of marker genes for endodermal (AFP and Foxa2), mesodermal (Brachyury and Flk1), and ectodermal (FGF5 and Pax6) [19,20] levels were analyzed (Figure 6A).
The Foxa2 expression level in 31002-treated cells was much lower than that in the vehicle control on DD3. However, the level showed a gradual increase with time in 31002-treated cells and a gradual decrease in vehicle control cells and was reversed between the cell groups by DD15. In the case of FGF5 expression, high expression levels observed on DD3 decreased abruptly in both cell groups by DD 9. However, full-recovery of the FGF5 expression level was observed by DD15 in 31002-treated cells, but not in vehicle control cells (Figure 6A). It should also be noted that there was a tendency for higher expression of Flk1 and Pax6 genes in 31002-treated cells compared to vehicle control cells in the late differentiation stage (between DD12 and DD15).
Nkx2.5 and GATA4 are required for cardiac commitment of mesodermal cells and are known to be expressed in the early stage of cardiac differentiation [19,20]. On the contrary, α-MHC and MLC-2v are cellular components of cardiomyocytes; therefore, these molecules are known to appear at the later stage of differentiation. In the present study, 31002 treatment inhibited the abrupt down-regulation of Nkx2.5 gene expression shown in vehicle control cells at the late stage of differentiation (Figure 6B). However, no effect of 31002 treatment was observed on the expression of GATA4. For α-MHC and MLC-2v, as expected from the higher EGFP expressing cell ratio, a much higher expression level was observed in 31002-treated cells compared to that of vehicle controls on DD15.
ESCs are one of the most promising tools for cell-based therapy to recover damaged myocardium [2]. However, in order for ESCs to be applicable for clinical use, many limitations must be resolved. One critical limitation is the inefficiency of directed differentiation of ESCs to cardiomyocytes. To overcome this hurdle, various genetic techniques, protein factors, and chemical compounds [19-21] have been applied to optimize differentiation protocols. Known biologically active molecules used for this purpose include periostin [11], transforming growth factor beta (TGFwith bone morphogenetic protein 2 (BMP2) [20,22,23], insulin-like growth factor (IGF) [19], nitric oxide [24], reactive oxygen species [25], and oxytocin [17]. Several chemical compounds such as 6-bromoindirubin-3'-oxime (BIO) [21], ascorbic acid [16,26], retinoic acid, and DMSO [17] are known to induce cardiomyocyte differentiation of ESCs as well. Although significant progress has been made in producing cardiac lineage cells, the efficiency for directed differentiation of ESCs to cardiomyocytes is currently insufficient for clinical utility and our limited understanding of the mechanisms involved in the process further limits the refinement of differentiation protocols.
To break through these current limitations, we attempted to identify new compounds with the potential to induce directed differentiation of ESCs to cardiomyocytes. For this purpose, we established a screening system using KH2 ESCs stably transfected with an α-MHC promoter-driven EGFP expression vector [18]. The cells were successfully used to identify a group of compounds, including 31002, which could act as cardiomyocyte-directed differentiation stimulants for ESCs. Those found to be active had a common structure of 1H-pyrrole,2,2'-(phenylmethylene)bis and their effect was dose-dependent. We also observed an indication that 31002 might have cardiomyogenic differentiation-enhancing activity in human ESCs (our unpublished data). Clarification as to the action mechanism of 31002 with regard to cardiomyogenic differentiation of ESCs will be beneficial to this area of research.
In contrast to several other cardiomyogenic stimulants for ESCs which are known to elicit their effects during the early stages of differentiation [15,16], the stimulating effect of 31002 was obvious only with treatment during the later stages of ESC differentiation. When KH2 cells were differentiated according to the protocol described in Methods without 31002, the once elevated expressions of Nkx2.5, α-MHC, and MLC-2v in the middle stages of differentiation declined in the later stages. This change can be attributed to the relative decrease in the ratio of cardiomyocytes among the total cells. However, KH2 cells differentiated in the presence of 31002 did not down-regulate gene expression, and even showed enhanced expression in the case of Nkx2.5 and MLC-2v in the later stage of differentiation. This finding is consistent with the abrupt increase in the ratio of cardiomyocytes in cells differentiated with 31002.
Embryonic developmental studies showed that endodermal factors such as Nodal, BMP2, FGFs, and Shh affect cardiac development [20,27]. In the present study, although the expression of the endodermal marker Foxa2 was inhibited in the early differentiation stage by 31002 treatment, it was gradually promoted with time, resulting in much higher expression in the later stage of differentiation compare to vehicle control cells. Activation of endodermal cells might be connected to the increased production of endodermal factors which can stimulate cardiomyogenic differentiation. The ectodermal marker gene FGF5 also showed an unusual increase in the later stage of differentiation with 31002 treatment. It should also be noted that the Pax6 expression levels in 31002-treated cells was superior to that in vehicle control cells during the later stages of differentiation. Collectively, these results imply that 31002 may inhibit early activation of the three germ layer-forming stages and prolong the stage which is indispensable for cardiomyogenic commitment of ESCs. These results are consistent with the observation that the EGFP expressing cell ratios in 31002-treated cells during the last three differentiation days were similar to those in cells treated with 31002 for all the differentiation periods.
Again, this finding could also explain why the expression levels of Nkx2.5, α-MHC, and MLC-2v are maintained high or even increased in the later stage of the differentiation period. The Nkx2.5 gene is expressed during the cardiac commitment of mesodermal cells and is known to be expressed in the early stage of cardiac differentiation [19]. Therefore, provided that the three germ layer stages continue until DD15, cardiac committed cells expressing Nkx2.5 will be increased, resulting in remarkable increases of cardiomyocytes and increased expression of α-MHC and MLC-2v genes. The effect of 31002 in ESC differentiation appears to be specific to the cardiomyogenic pathway, because we could not detect any enhancing effects on hematopoietic lineage or neuronal lineage cell differentiations (our unpublished data).
In conclusion, 31002 might support a continuous fate commitment of undifferentiated ESCs to the cardiac lineage by prolonging the early differentiating stage, resulting in an increase of cardiomyocytes. Therefore, the compounds in this group, particularly 31002, might be used as a directed cardiomyogenic differentiation inducer to produce cells for cell therapy to repair damaged heart tissue.
Acknowledgments
We thank the Korea Chemical Bank for providing test compounds. This study was supported by a grant (Nam KH) from KOSEF and a grant (Kim HC) from the KRIBB Research Initiative Program.
References
1. Cohn JN, Bristow MR, Chien KR, Colucci WS, Frazier OH, Leinwand LA, Lorell BH, Moss AJ, Sonnenblick EH, Walsh RA, Mockrin SC, Reinlib L. Report of the National Heart, Lung, and Blood Institute Special Emphasis Panel on Heart Failure Research. Circulation. 1997; 95(4):766–770. PMID: 9054723.


2. Puceat M. Human embryonic stem cell: is it a realistic cell source for regenerative therapy of heart failure? Pathol Biol (Paris). 2008; 56(2):47–49. PMID: 18178334.
3. Pasumarthi KB, Field LJ. Cardiomyocyte cell cycle regulation. Circ Res. 2002; 90(10):1044–1054. PMID: 12039793.


5. Kehat I, Kenyagin-Karsenti D, Snir M, Segev H, Amit M, Gepstein A, Livne E, Binah O, Itskovitz-Eldor J, Gepstein L. Human embryonic stem cells can differentiate into myocytes with structural and functional properties of cardiomyocytes. J Clin Invest. 2001; 108(3):407–414. PMID: 11489934.
6. Cleland JG, Freemantle N, Coletta AP, Clark AL. Clinical trials update from the American Heart Association: REPAIR-AMI, ASTAMI, JELIS, MEGA, REVIVE-II, SURVIVE, and PROACTIVE. Eur J Heart Fail. 2006; 8(1):105–110. PMID: 16387630.


7. Urbanek K, Quaini F, Tasca G, Torella D, Castaldo C, Nadal-Ginard B, Leri A, Kajstura J, Quaini E, Anversa P. Intense myocyte formation from cardiac stem cells in human cardiac hypertrophy. Proc Natl Acad Sci USA. 2003; 100(18):10440–10445. PMID: 12928492.


8. Beltrami AP, Barlucchi L, Torella D, Baker M, Limana F, Chimenti S, Kasahara H, Rota M, Musso E, Urbanek K, Leri A, Kajstura J, Nadal-Ginard B, Anversa P. Adult cardiac stem cells are multipotent and support myocardial regeneration. Cell. 2003; 114(6):763–776. PMID: 14505575.


9. Laugwitz KL, Moretti A, Lam J, Gruber P, Chen Y, Woodard S, Lin LZ, Cai CL, Lu MM, Reth M, Platoshyn O, Yuan JX, Evans S, Chien KR. Postnatal isl1+ cardioblasts enter fully differentiated cardiomyocyte lineages. Nature. 2005; 433(7026):647–653. PMID: 15703750.
10. Hsieh PC, Segers VF, Davis ME, MacGillivray C, Gannon J, Molkentin JD, Robbins J, Lee RT. Evidence from a genetic fate-mapping study that stem cells refresh adult mammalian cardiomyocytes after injury. Nat Med. 2007; 13(8):970–974. PMID: 17660827.


11. Kuhn B, del Monte F, Hajjar RJ, Chang YS, Lebeche D, Arab S, Keating MT. Periostin induces proliferation of differentiated cardiomyocytes and promotes cardiac repair. Nat Med. 2007; 13(8):962–969. PMID: 17632525.


12. Ménard C, Hagège AA, Agbulut O, Barro M, Morichetti MC, Brasselet C, Bel A, Messas E, Bissery A, Bruneval P, Desnos M, Pucéat M, Menasché P. Transplantation of cardiac-committed mouse embryonic stem cells to infarcted sheep myocardium: a preclinical study. Lancet. 2005; 366(9490):1005–1012. PMID: 16168783.


13. Tomescot A, Leschik J, Bellamy V, Dubois G, Messas E, Bruneval P, Desnos M, Hagège AA, Amit M, Itskovitz J, Menasché P, Pucéat M. Differentiation in vivo of cardiac committed human embryonic stem cells in postmyocardial infarcted rats. Stem Cells. 2007; 25(9):2200–2205. PMID: 17540853.
14. Thomson JA, Itskovitz-Eldor J, Shapiro SS, Waknitz MA, Swiergiel JJ, Marshall VS, Jones JM. Embryonic stem cell lines derived from human blastocysts. Science. 1998; 282(5391):1145–1147. PMID: 9804556.


15. Wobus AM, Kaomei G, Shan J, Wellner MC, Rohwedel J, Ji G, Fleischmann B, Katus HA, Hescheler J, Franz WM. Retinoic acid accelerates embryonic stem cell-derived cardiac differentiation and enhances development of ventricular cardiomyocytes. J Mol Cell Cardiol. 1997; 29(6):1525–1539. PMID: 9220339.


16. Takahashi T, Lord B, Schulze PC, Fryer RM, Sarang SS, Gullans SR, Lee RT. Ascorbic acid enhances differentiation of embryonic stem cells into cardiac myocytes. Circulation. 2003; 107(14):1912–1916. PMID: 12668514.


17. Paquin J, Danalache BA, Jankowski M, McCann SM, Gutkowska J. Oxytocin induces differentiation of P19 embryonic stem cells to cardiomyocytes. Proc Natl Acad Sci USA. 2002; 99(14):9550–9555. PMID: 12093924.


18. Kim EK, Seo YJ, Oh GT, Yoon M, Lee Y, Kim HC, Choi YK, Lee CH, Kang JS, Nam KH. Fluorescence labeled cardiomyocytes derived from embryonic stem cells. Lab Anim Res. 2005; 21(1):60–68.
19. Boheler KR, Czyz J, Tweedie D, Yang HT, Anisimov SV, Wobus AM. Differentiation of pluripotent embryonic stem cells into cardiomyocytes. Circ Res. 2002; 91(3):189–201. PMID: 12169644.


20. Yuasa S, Itabashi Y, Koshimizu U, Tanaka T, Sugimura K, Kinoshita M, Hattori F, Fukami S, Shimazaki T, Ogawa S, Okano H, Fukuda K. Transient inhibition of BMP signaling by Noggin induces cardiomyocyte differentiation of mouse embryonic stem cells. Nat Biotechnol. 2005; 23(5):607–611. PMID: 15867910.


21. Tseng AS, Engel FB, Keating MT. The GSK-3 inhibitor BIO promotes proliferation in mammalian cardiomyocytes. Chem Biol. 2006; 13(9):957–963. PMID: 16984885.


22. Behfar A, Zingman LV, Hodgson DM, Rauzier JM, Kane GC, Terzic A, Puceat M. Stem cell differentiation requires a paracrine pathway in the heart. FASEB J. 2002; 16(12):1558–1566. PMID: 12374778.


23. Min JY, Yang Y, Sullivan MF, Ke Q, Converso KL, Chen Y, Morgan JP, Xiao YF. Long-term improvement of cardiac function in rats after infarction by transplantation of embryonic stem cells. J Thorac Cardiovasc Surg. 2003; 125(2):361–369. PMID: 12579106.


24. Kanno S, Kim PK, Sallam K, Lei J, Billiar TR, Shears LL 2nd. Nitric oxide facilitates cardiomyogenesis in mouse embryonic stem cells. Proc Natl Acad Sci USA. 2004; 101(33):12277–12281. PMID: 15304656.


25. Sauer H, Rahimi G, Hescheler J, Wartenberg M. Role of reactive oxygen species and phosphatidylinositol 3-kinase in cardiomyocyte differentiation of embryonic stem cells. FEBS Lett. 2000; 476(3):218–223. PMID: 10913617.


26. Sato H, Takahashi M, Ise H, Yamada A, Hirose S, Tagawa Y, Morimoto H, Izawa A, Ikeda U. Collagen synthesis is required for ascorbic acid-enhanced differentiation of mouse embryonic stem cells into cardiomyocytes. Biochem Biophys Res Commun. 2006; 342(1):107–112. PMID: 16480687.


27. Menard C, Grey C, Mery A, Zeineddine D, Aimond F, Puceat M. Cardiac specification of embryonic stem cells. J Cell Biochem. 2004; 93(4):681–687. PMID: 15389971.
Figure 1
Chemical structure of 31002 and the structural derivatives showing activity propensity for cardiomyocyte-directed differentiation of embryonic stem cells (ESCs).
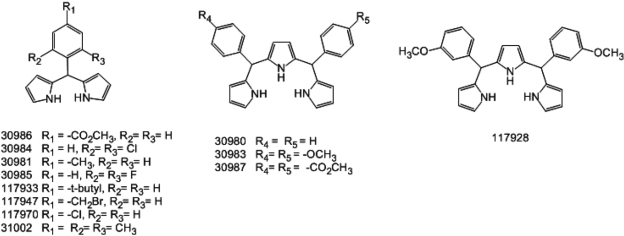
Figure 2
Activity of test compounds for cardiomyogenic differentiation induction of ESCs. KH2 ESCs were differentiated with one of the compounds at a final concentration of 1or 10 mM as described in Materials and Methods. On day 15 of differentiation, the cells were trypsinized, fixed with 1% formaldehyde and subjected to fluorescence activated cell sorter (FACS) analysis to detect enhanced green fluorescent protein (EGFP) expressing cells. Three independent experiments were repeated and the representative result is shown. All the experiments were done in triplicate.
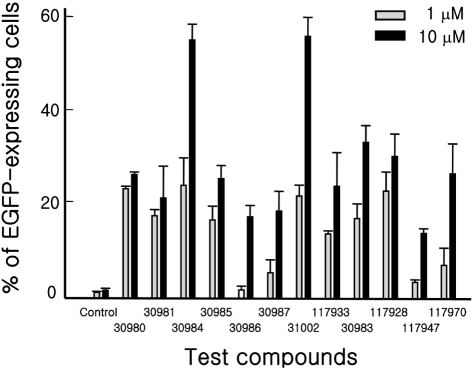
Figure 3
Co-expression of cardiomyocyte marker proteins in EGFP-expressing cells. (A) Representative flow cytometric plots of differentiated KH2 cells in the presence of 10 mM 31002. KH2 ESCs were differentiated with 10 mM 31002 as described in Materials and Methods. On day 15 of differentiation, the cells were trypsinized, fixed with 1% formaldehyde and subjected to FACS analysis to detect EGFP expressing cells. (B) Differentiated cells on day 15 were fixed and immunostained for troponin T (b and d; red) or α-actinin (f; red) as described in Materials and Methods. EGFP (green) in the cells was observed under a fluorescence microscope (a, c and e). Three independent experiments were repeated and the representative results are shown. Troponin T (×100), α-actinin (×200).
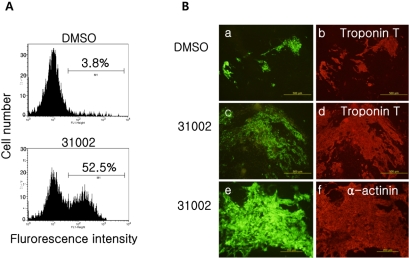
Figure 4
Time-dependent increase of EGFP-positive cells. KH2 ESCs were differentiated with 10 mM 31002 as described in Materials and Methods. On the differentiation days indicated, the cells were trypsinized, fixed with 1% formaldehyde, and subjected to FACS analysis to detect EGFP expressing cells. Three independent experiments were repeated and the representative results are shown. All experiments were performed in triplicate.
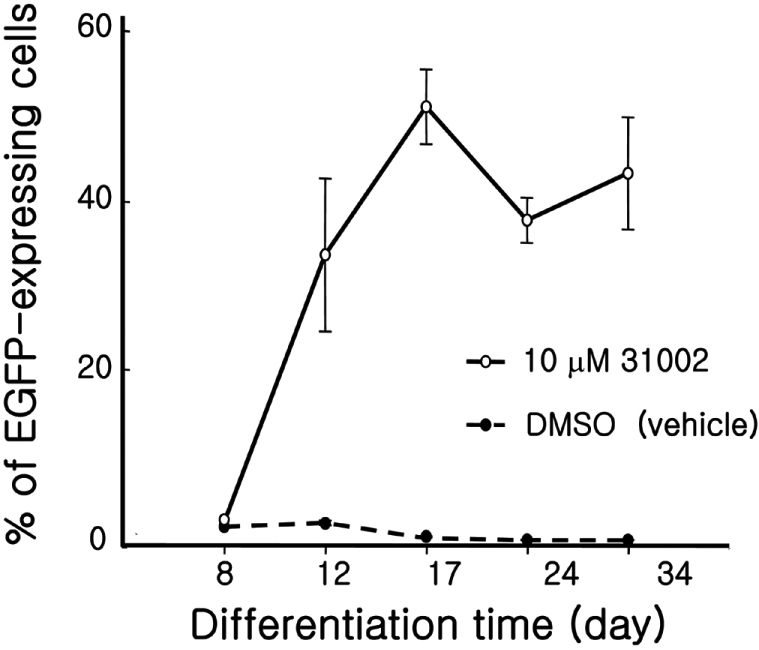
Figure 5
The effects of periodic 31002 treatments on cardiomyogenic differentiation of ESCs. KH2 cells were differentiated for 15 days according to the differentiation protocol described in Materials and Methods. The cells were treated with 10 µM 31002 during only the indicated period. On day 15, flow cytometric analysis was performed for all cell groups. Three independent experiments were repeated and representative results are shown. All experiments were performed in triplicate. *Significant difference at P<0.05 compared to Group 1.
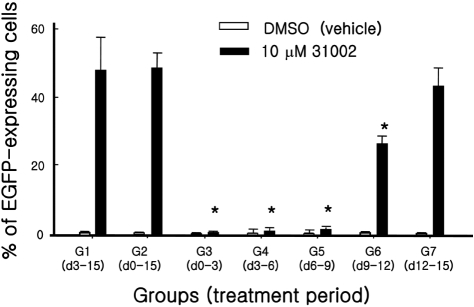
Figure 6
Marker gene expressions of differentiating ESCs. KH2 ESCs were differentiated in the presence of 10 µM 31002 as described in Materials and Methods. Total RNA was obtained from the differentiating cells collected at the indicated times. Marker genes for three germ layers (A) and cardiac lineage cells (B) were analyzed by semi-quantitative RT-PCR. The plots in the right panel show the relative expression levels of target genes which were normalized to β-actin expression. Open- and filled-symbols indicate dimethyl sulfoxide (DMSO) and 31002 treatments, respectively.
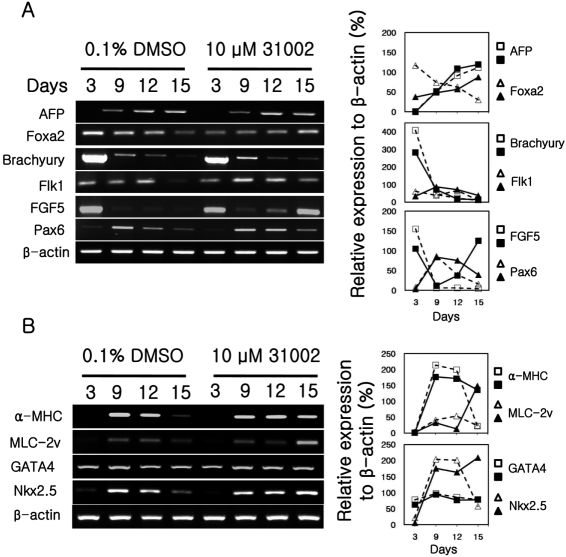