Abstract
Tribromoethanol (2,2,2-tribromoethanol, TBE) is a popular injectable anesthetic agent used in mice in Korea. Our goal was to assess the risks associated with side effects (lesions) in the abdominal cavity, especially at high doses. To understand the underlying pathophysiological changes, we examined levels of cytokines through ELISA of abdominal lavage fluid and spleen collected from mice treated with low and high-dose TBE. ICR mice were anesthetized using one of the following protocols: a combination of TBE 200 mg/kg (1.25%) and xylazine 10 mg/kg; TBE 400 mg/kg (1.25%); and TBE 400 mg/kg (2.5%). Administration of high-dose TBE (400 mg/kg) increased the interleukin-1β and interleukin-6 levels in the peritoneal cavity over the short term (<1 day) compared with sham controls and low-dose TBE (200 mg/kg) groups. Cytokine expression in the low-dose TBE group was similar to the control group, whereas in the high-dose TBE group cytokine levels were higher in abdominal lavage fluid and spleen over the long term (10 days post-injection). We conclude that a combination of TBE 200 mg/kg (1.25%) and xylazine (10 mg/kg) is a safe and effective anesthetic for use in animals.
Tribromoethanol (2,2,2-tribromoethanol, TBE) has the ability to rapidly induce deep anesthesia followed by a rapid and complete recovery [1]. As a result of a TBE preparation experiment performed to determine an adequate dose of TBE, we determined whether inducing anesthesia with a TBE injection alone can cause adverse effects due to the toxicity of the chemical, including retention of food in the digestive tract, peritonitis and fibrinoid adhesions. TBE is an irritant, especially at high concentrations or with repeated use. Anesthesia with TBE can result in ileus, abdominal adhesions, increased generalized morbidity, and increased mortality [2-8]. Since TBE produces various side effects, it has been recommended only for acute terminal studies when administrated intraperitoneally (IP) to laboratory animals [9]. While such adverse effects have been found in earlier studies [5,6,8], some recent studies claimed that they could not find any adverse effects [1].
In preliminary experiments, all animals that were injected with a low dose of 1.25% TBE (200 mg/kg) displayed clear signs of light anesthesia with a strong pedal withdrawal reflex. Despite the good anesthetic effect, severe adverse effects were displayed at 2.5% TBE (400 mg/kg). A mixture of 1.25% TBE (200 mg/kg) and xylazine (10 mg/kg) appeared to be safe and provided satisfactory anesthesia in ICR mice [10].
There has been relatively little information in the literature regarding proinflammatory cytokine expression in abdominal lavage fluid and spleen lymphoid tissues [1,6,8,11]. In an attempt to reduce the side effects of TBE, Gopalan et al [2] used a combination of a lower dose of TBE and medetomidine in Sprague-Dawley (SD) rats [2]. In the present study we tested a different anesthetic combination, TBE and xylazine (an α2-adrenergic agonist that has sedative, muscle-relaxation, and analgesic properties) in ICR mice [12]. No published reports indicated significant proinflammatory cytokine expression in abdominal lavage fluid and spleen [1,6,8,11]. Inflammation involves production and release of inflammatory mediators, including three major groups of: proinflammatory, chemotactic, and anti-inflammatory cytokines [13]. Classically activated macrophages secrete proinflammatory cytokines, such as tumor-necrosis factor-α (TNF-α), interleukin-1β (IL-1β), and IL-6 [14,15]. In mice, TNF-α is the first inflammatory cytokine to be produced de novo. It induces synthesis of IL-1β [16]. IL-1β is the only IL-1 species present during acute experimental inflammation in mouse peritoneal exudates [17]. Functionally, TNF-α and IL-1β are closely related; they act synergistically to induce the expression of chemokines and up-regulate cell adhesion molecules, thus triggering leukocyte emigration to the inflammatory site [13].
Although 1.25% TBE (12.5 mg/mL) is typically used in mouse experiments, we had to increase the concentration to 2.5% (25 mg/mL) for the 400 mg/kg dose to make it adequate for IP administration. For example, in order to administer 400 mg/kg of 1.25% TBE to a 25 g mouse, 0.8 mL is required, which exceeds the recommended quantity (0.5 mL or less) and can potentially cause adverse effects [18]. It was difficult to confirm whether the adverse effects displayed in the group administered 2.5% TBE 400 mg/kg were caused by the concentration or by the dose of TBE. Hence, we determined whether a connection exists between tribromoethanol concentration and dosage in peritonitis.
In the present study, our goals were (1) to measure bacterial growth in abdominal lavage fluid of anesthetized mice and (2) to investigate the direct influence of proinflammatory cytokines (IL-1β, IL-6, and TNF-α) in abdominal lavage fluid and spleen.
Male ICR mice (n=48; weight, 20 to 29 g; 4-6 weeks old) were obtained from NaraBiotech (Pyeongkaek, Korea). These mice were free of common rodent viruses, bacteria, and parasites. Animals were housed in a barrier facility in individual ventilated caging systems with β-chip bedding on a 12:12-h light:dark cycle. The room temperature was 23±1℃, with relative humidity of 50±5% and with 25 complete changes of filtered air per hour. Animals housing was five mice per cage. Gamma-sterilized fomula-m07 food (Feed Lab, Guri, Korea) and autoclaved tap water by bottle were provided ad libitum. Animal care and experimental procedures were approved by the animal care and use committee of Konkuk University and were in accordance with published National Institutes of Health guidelines [19].
Commercially available 2,2,2-tribromoethanol powder (T48402; Sigma-Aldrich, St. Louis, MO, USA) was used for all studies. To prepare a 1 g/mL stock solution, a measured amount of TBE powder was placed into a 50 mL polypropylene conical tube (Becton Dickinson Labware, Franklin Lakes, NJ, USA) that was wrapped in aluminum foil. A micro-pipette was used to add t-amyl-alcohol (TAA, 240486; Sigma-Aldrich), and the solution was vortexed until it dissolved at room temperature (25℃). To prepare fresh dilutions of TBE (200 and 400 mg/kg) in volumes of 0.35-0.50 mL, an appropriate volume of sterilized phosphate-buffered saline (PBS, P5368; Sigma-Aldrich) was added to a stock solution and vortexed until the whole stock solution was dissolved in PBS at 25℃. Working solutions were filtered using sterile technique with a 0.20 µm disposable syringe filter unit (Mixed Cellulose Ester, ADVANTEC®, Toyo Roshi Kaisha, Ltd., Tokyo, Japan). Using filtering, working solutions were obtained and placed in sterilized glass tubes capped with rubber stoppers and wrapped in aluminum foil. The preparer wore gloves, glass vials were autoclaved, and all glassware was triple-rinsed with distilled water before being autoclaved. Newly prepared TBE solution was stored in the dark at 4℃.
All animals received a single IP injection using a 26G 1-mL syringe in the lower-right quadrant of the mice's abdomen [20,21]. Details of the anesthetic protocols are given in Table 1. Animals were weighed and given one of the anesthetic combinations. The animal was placed back in its home cage until it lost its righting reflex. Once the righting reflex was lost, mice were maintained on a temperature-controlled heating platform (38℃, Jungdo B&P, Seoul, Korea), and their eyes were lubricated with ointment (Solco®, Hanlim Pharm Co., Ltd., Seoul, Korea). Assessment of the pedal withdrawal reflex was performed to ensure that the animal was maintained at the correct depth of anesthesia. To apply pressure uniformly, the pedal withdrawal reflex was assessed using a Touch-Test™ sensory evaluator (North Coast Medical Inc., San Jose, CA, USA) with a target force of 300 g, which indicates deep pressure sensation, every 5 min [4]. For the pedal withdrawal reflex, the mouse's hind limb was slightly extended and the interdigital webbing was touched by the sensory evaluator. A clear attempt to withdraw the limb was judged a positive reaction. All animals breathed room air for the duration of the experiment. Mice were monitored for clinical signs of illness such as lethargy, unkempt hair coat, hunched posture, or dehydration. At 4 h post-injection (HPI) and 1, 4 and 10 days post-injection (DPI), three mice from each group were sacrificed.
Animals were deeply anesthetized with isoflurane at each time point after injection. The peritoneal cavity was lavaged with 2 mL sterile saline, each mouse received a 10-sec gentle manual massage, and exudates (>1 mL) were retrieved, centrifuged at 2,000 g for 10 min, and frozen at -80℃ for later cytokine assessment [22]. Animals were then exsanguinated by placing a needle in the caudal vena cava. The spleen was excised aseptically and homogenized in 1×PBS (100 mg/mL) using a homogenizer (T10 basic ULTRA-TURRAX®, IKA Laboratory Equipment, Staufen, Germany) and centrifuged at 14,000 rpm for 10 min at 4℃ [23]. Supernatant was collected, aliquoted and stored at -80℃ for further use in ELISA.
Bacterial counts were done for aseptically harvested peritoneal fluid at each time point after injection. Abdominal lavage fluid (0.1 mL) obtained to assess cytokines was cultured on tryptic soy agar plates (HiMedia Laboratories Limited, LBS Marg, Mumbai, India). Plates were incubated (37℃) for 48 h, and bacterial growth was investigated.
To examine secreted and local cytokine production, cytokine specific ELISA was performed on abdominal lavage fluid and on extracted proteins from spleens in each group. Commercially-available kits from Biosource (Invitrogen, Carlsbad, CA, USA) were used per the manufacturer's guidelines. In each cytokine specific ELISA, we used 10 mg of the total extracted protein of the spleen.
All statistical analyses were carried out using SPSS version 14.0K and GraphPad Prism version 4.00 for Windows (GraphPad Software, San Diego, CA, USA). All data were presented as means±SEM. Comparisons of the two different anesthesia groups were made by Student's unpaired t-tests. One-way analysis of variance (ANOVA) was performed with Tukey's adjustment for multiple comparisons to evaluate differences among each anesthetic group for cytokine expression level. A value of P<0.05 was considered significant.
No bacteria were detected in abdominal lavage fluid of mice after a 48-h culture.
By 10 days after anesthetic administration, all strains in group 2 also manifested the following clinical signs of disease: dehydration, disheveled appearance, porphyrin staining of the eyes and nose, and hunched posture. Grossly, ICR mice in protocols 2 and 3 had evidence of ileus and thin abdominal walls at 1 and 4 DPI. Six and eight ICR mice in protocols 2 and 3 had focally extensive areas of fibrous adhesions between the abdominal wall, cecum, and small intestine, which were filled with gas and/or fluid at 10 DPI.
The amount of secreted cytokines in the abdominal lavage fluid was expressed as pg protein/mL (Table 2). Mice in protocol 2 showed a significant increase in IL-1β levels (131.5±20.8 pg/mL) in comparison to sham animals (28.6±4.7 pg/mL) and protocol 1 (43.1±19.7 pg/mL) at 4 HPI (P<0.05). Mice in protocol 2 showed a significant increase in IL-1β levels (83.4±1.6 pg/mL) in comparison to sham (10.5±0.6 pg/mL) and protocol 1 (12.7±1.6 pg/mL) at 4 DPI (P<0.05). Mice in protocol 3 showed a significant increase in IL-1β levels (190.1±21.3 pg/mL) in comparison to sham control and protocol 1 at 4 HPI (P<0.05). Significant differences were found in IL-1β expression between (a) protocol 3 (145.5±18.2 pg/mL) and (b) control mice (26.7±6.1 pg/mL), protocol 1 mice (16.8±0.7 pg/mL) and protocol 2 mice (76.0±11.6 pg/mL) at 1 DPI, but not at 4 and 10 DPI (P<0.05) (Table 2).
Mice in protocol 2 showed a significant increase in IL-6 levels (1,465.4±432.8 pg/mL) in comparison to sham (130.2±92.1 pg/mL) and protocol 1 (209.3±48.9 pg/mL) at 4 HPI (P<0.05). Significant differences were found in IL-6 expression between (a) protocol 3 (2786.0±364.2 pg/mL) and (b) control (130.2±92.1 pg/mL), protocol 1 (209.3±48.9 pg/mL) and protocol 2 (1465.4±432.8 pg/mL) at 4 HPI (P<0.05). IL-6 was not secreted in detectable amounts in control and protocol 1 at 4 and 10 DPI as well as protocol 3 at 10 DPI (Table 2).
TNF-α expression showed no significant difference between controls and the other groups at any time (Table 2).
Cytokines production was expressed as pg/mL supernatant of spleen (Table 3). A significant difference was found in IL-1β expression between sham and protocol 3 mice spleen at 1, 4 and 10 DPI (P<0.05). IL-1β production in protocol 1 (126.8±6.6 and 116.9±11.1 pg/mL) was less than that in protocol 3 (235.2±15.5 pg/mL) at 4 DPI and less than that in protocol 2 (216.4±13.1 pg/mL) at 10 DPI (P<0.05). Mice in protocol 2 showed a significant increase in IL-1β levels (219.9±1.8 and 216.4±13.1 pg/mL) in comparison to sham (114.0±10.8 and 112.6±14.3 pg/mL) at 4 and 10 DPI (P<0.05).
A nearly constant amount of IL-6 was expressed at all days in protocol 2 (84-92 pg/mL) and protocol 3 [110-113 pg/mL]. IL-6 production in the sham group was higher (152.2±6.5 pg/mL) than in protocols 1, 2 and 3 (98.0±6.8, 89.2±2.5 and 113.6±3.6 pg/mL, respectively) at 4 HPI (P<0.05) but not at 1, 4 and 10 DPI. A significant increase was presented in protocol 3 mice compared with sham at 1, 4 and 10 DPI (P<0.05). The level for protocol 2 (84.0±2.1 pg/mL) was significantly higher than for sham (45.2±5.9 pg/mL) at 4 DPI. The level for protocol 2 (92.3±5.5 pg/mL) was significantly higher than for sham (33.3±2.9 pg/mL) and for protocol 1 (27.8±3.2 pg/mL) at 10 DPI (P<0.05). A significant increase was present in protocol 3 mice in comparison with protocol 1 mice at 10 DPI (P<0.05).
In the spleen of sham controls (371.1±26.0 pg/mL) at 4 HPI, TNF-α levels were significantly higher than for protocol 1, 2, and 3 mice (272.0±13.0, 93.1±15.7, and 194.2±20.8 pg/mL, respectively). However, protocol 2 and 3 mice had significantly higher values (82.4±14.2 and 80.2±3.1 pg/mL, respectively) than sham and protocol 1 mice (15.6±0.4 and 15.2±0.3 pg/mL, respectively) at 10 DPI (P<0.05).
To understand the underlying pathophysiological changes, we examined the level of cytokines and protein levels through ELISA experiments with abdominal lavage fluid and spleen fluid collected from mice treated with low-dose TBE. TBE at 400 mg/kg was a reliable anesthetic that provided surgical anesthesia for approximately 40 min in ICR mice [10]. However, it is recommended that one does not increase the TBE dose since fibrous adhesions in the abdominal cavity, irritation, and mortalities have been reported, especially with high doses or repetitive use [2]. The combination of 200 mg/kg TBE and 10 mg/kg xylazine induced proper anesthetic depth in ICR mice [10]. The dose of 400 mg/kg TBE used in protocol 2 was based on doses reported in the literature [1,8,24]. While the lowest dose of TBE that resulted in adequate anesthesia, based on the toe-pinch reflex, was a dose of 375 mg/kg, the lowest dose that consistently produced adequate anesthesia in all ICR mice tested was 400 mg/kg [4]. However, fibrous adhesions between the abdominal wall, cecum, and small intestine, which were filled with gas and/or fluid, were observed in all mice strains for protocol 2. These side effects are induced by TBE per se and not related to the solvent used [6,8]. External stimuli such as peritoneal insults that induce inflammatory cascades, subsequently caused a reduction in gastrointestinal motility [25]. Therefore, in the present study, local inflammation in the peritoneal cavity can be postulated as the cause.
In this study, local cytokine production was investigated in spleen and abdominal lavage fluid for TBE-administered mice using intraperitoneal injection. The use of anesthesia induces a disturbed balance of pro- and anti-inflammatory cytokines in certain states [26]. To the best of our knowledge, it is the first study to examine changes in cytokine profiles in these samples using the IP route for TBE anesthetics in mice. In the present study, spleen was used because it is an organ that is a prominent marker in different peritonitis models and therefore can be used as an abdominal organ representative of other lymphatic organs [27].
The injection of a wide range of irritants into the peritoneal cavity induces the hallmarks of inflammation, including pain, leukocyte infiltration, and synthesis of inflammatory mediators [28]. IL-1β, IL-6, and TNF-α were selected as proinflammatory mediators. These cytokines are essential in helping to initiate and potentiate inflammatory responses [29]. For example, IL-1β and IL-6 are accepted as markers for tissue reactions and inflammation in pain-induced stress, and for mechanical trauma or surgical stress, respectively. TNF-α is a marker for bacterial endotoxin or enterotoxin-induced immune stimulation [30,31]. The peritoneum is mainly protected by the innate immune system [32]. Peritoneal insults induce the influx of polymorphonuclear neutrophils [14]. Peritoneal mesothelial cells participate during the recruitment of phagocytes in to the peritoneal cavity by responding to macrophage-derived cytokines TNF-α and IL-1β [14].
Surprisingly, there was no increased expression of IL-1β and IL-6 in TBE-treated groups compared with sham, but a decrease in TNF-α expression at 4 HPI in spleen. These results are in close agreement with those of previous studies comparing cytokine mRNA levels in the spleen of rats injected with 400 mg/kg TBE [27]. In this context, Bette et al [27] reported that a single injection of high-dose TBE did not initiate 'chemically-induced peritonitis' by inflammation or infection in the abdomen [27]. However, this result showed that these cytokines in sham control and protocol 1 mice sharply declined from 1 to 10 DPI in spleen. Proinflammatory cytokines of protocol 2 and 3 in spleen were significantly higher than sham and protocol 1 at 4 and 10 DPI. Prolonged stimulation of the inflammatory response may lead to tissue damage and there is evidence linking mice incapable of producing IL-6 with decreased mortality and inflammatory responses when they are injected IP with zymosan [33,34]. It was speculated that there is a correlation between late effects in these cytokine levels and histopathological changes of abdominal organs [10].
In addition, to confirm a correlation between pathologic changes and concentrations of TBE, we compared protocol 2 (1.25% TBE 400 mg/kg) with protocol 3 (2.5% TBE 400 mg/kg) regarding these proinflammatory cytokines. Cytokine levels of protocol 3 were substantially increased compared with protocol 2 in abdominal lavage fluid and spleen at each time point.
IP drug delivery is overused in rodent studies and carries many concerns such as contamination, splenic trauma, serosal hemorrhage, drug delivery into visceral organs or fat pads, urinary bladder, muscle, and other untoward effects [12]. Some believe that the intestines cannot be penetrated by an IP injection because they are free to move away from the needle, whereas about 10 to 20% of IP injections are placed somewhere other than the peritoneal cavity [21,35]. In an attempt to confirm correct placement, most texts advise gently withdrawing the syringe plunger before administering the dose. The disadvantage of this is that, although urine will be readily aspirated, intestinal or cecal contents are not easy to be aspirated through the gauge of needle normally used. There will also be no indication that the needle has penetrated fat or muscle [36]. Indeed, IP injections have been described as 'injections into a black box', because the error in IP injections may not be recognized [37]. Hence, the skill of the technician will be critical to ensure accurate delivery. In this experiment, correct administration could be directly assessed by whether animals reached a deep anesthetic stage. If such failures had been detected, the subject would have been omitted from the experiment.
Physiologic parameters such as changes in respiratory rate, heart rate, or blood pressure are the most sensitive, objective, and quantitative indicators of anesthetized animals [12]. Such an approach is routinely used in human and companion animal anesthesia, whereas it is not possible for routine laboratory experiment with mice because of the requirement of specialized equipment for the small size of the animal and its extreme physiologic value [11]. Reflex assessment is easy to carry out and is considered an acceptable method and can be done without extensive equipment [38,39]. The pedal withdrawal reflex used in this study is the most suitable and reliable parameter to gauge surgical tolerance [11,39-41]. The hindlimb reflex is a more reliable indicator of anesthetic depth than the forelimb. It is more sensitive than a cutaneous reflex and a tail pinch reflex test [40]. Cutaneous reflexes showed only moderate sensitivity at an early stage of sedation, and tail pinch reflexes were unable to determine any of the stages of anesthesia [41]. In this study, to reduce sources of variation in response to the pedal withdrawal reflex, the same operator took measurements and assessed reflexes.
Bacterial translocation in a non-lethal rat model of zymosaninduced sterile peritonitis was detected in peritoneal fluid [34]. Zymosan particles, the insoluble polysaccharides (β-glucan and α-mannan components) of the cell walls of Saccharomyces cerevisiae, are commonly used for induction of acute peritonitis in mice [16,17,42-45]. Bacterial translocation is known as the passage of viable resident bacteria and macromolecules from the gut to the normally sterile intra-abdominal tissues [46]. In this study, no bacteria were cultured from the peritoneal fluid at any time points, but we are limited to culturing aerobic not anaerobic bacteria. However, in a previous study, bacterial growths from peritoneal fluid were detected simultaneously in aerobic and anaerobic culture environments [34]. Therefore, it is conceivable that aerobic bacteria detection alone is enough evidence whether TBE-induced peritonitis is the sterile inflammatory or not.
In conclusion, these data show that the effects of intraperitoneally-injected TBE on the immune system should always be considered. Hence, the usage of 400 mg/kg TBE should be avoided when studies are being designed to analyze long-term (10 days) as well as short-term (<1 day) effects on the immune system. In addition, the decreased cytokine level in the low-dose TBE+xylazine group means that this anesthetic regime is safe in terms of correlation between pathologic changes and concentration of TBE.
Acknowledgments
This work was supported by a grant (Code: 20070401034011) from BioGreen 21 Program, Rural Development Administration, Republic of Korea.
References
1. Gardner DJ, Davis JA, Weina PJ, Theune B. Comparison of tribromoethanol, ketamine/acetylpromazine, telazol/xylazine, pentobarbital, and methoxyflurane anesthesia in HSD:ICR mice. Lab Anim Sci. 1995; 45:199–204. PMID: 7603025.
2. Gopalan C, Hegade GM, Bay TN, Brown SR, Talcott MR. Tribromoethanol-medetomidine combination provides a safe and reversible anesthetic effect in Sprague-Dawley rats. Contemp Top Lab Anim Sci. 2005; 44:7–10. PMID: 15697191.
3. Green CJ. Neuroleptanalgesic drug combinations in the anaesthetic management of small laboratory animals. Lab Anim. 1975; 9:161–178. PMID: 240064.


4. Lieggi CC, Artwohl JE, Leszczynski JK, Rodriguez NA, Fickbohm BL, Fortman JD. Efficacy and safety of stored and newly prepared tribromoethanol in icr mice. Contemp Top Lab Anim Sci. 2005; 44:17–22. PMID: 15697193.
5. Lieggi CC, Fortman JD, Kleps RA, Sethi V, Anderson JA, Brown CE, Artwohl JE. An evaluation of preparation methods and storage conditions of tribromoethanol. Contemp Top Lab Anim Sci. 2005; 44:11–16. PMID: 15697192.
6. Reid WC, Carmichael KP, Srinivas S, Bryant JL. Pathologic changes associated with use of tribromoethanol (avertin) in the sprague dawley rat. Lab Anim Sci. 1999; 49:665–667. PMID: 10638506.
7. Tarin D, Sturdee A. Surgical anaesthesia of mice: Evaluation of tribromo-ethanol, ether, halothane and methoxyflurane and development of a reliable technique. Lab Anim. 1972; 6:79–84. PMID: 4553189.


8. Zeller W, Meier G, Burki K, Panoussis B. Adverse effects of tribromoethanol as used in the production of transgenic mice. Lab Anim. 1998; 32:407–413. PMID: 9807753.


9. Meyer RE, Fish RE. A review of tribromoethanol anesthesia for production of genetically engineered mice and rats. Lab Anim (NY). 2005; 34:47–52. PMID: 16261153.


10. Cho YJ, Lee JW, Kim JI, Lee YA, Kim TY, Han JS. Evaluation on efficacy and safety of tribromoethanol and tribromoethanol plus α-adrenergic agonists in different mouse strains. Lab Anim Res. 2010; 26(3):241–247.
11. Arras M, Autenried P, Rettich A, Spaeni D, Rulicke T. Optimization of intraperitoneal injection anesthesia in mice: drugs, dosages, adverse effects, and anesthesia depth. Comp Med. 2001; 51:443–456. PMID: 11924805.
12. Fish RE, Brown MJ, Danneman PJ, Karas AZ. Fish RE, editor. Pharmacology of injectable anesthetics, sedatives, and tranquilizers. Anesthesia and Analgesia in Laboratory Animals. 2008. Burlington: Academic Press;p. 27–82.
13. Dinarello CA. Role of pro- and anti-inflammatory cytokines during inflammation: experimental and clinical findings. J Biol Regul Homeost Agents. 1997; 11:91–103. PMID: 9498158.
14. Hall JC, Heel KA, Papadimitriou JM, Platell C. The pathobiology of peritonitis. Gastroenterology. 1998; 114:185–196. PMID: 9428232.


15. Herwig R, Glodny B, Kuhle C, Schluter B, Brinkmann OA, Strasser H, Senninger N, Winde G. Early identification of peritonitis by peritoneal cytokine measurement. Dis Colon Rectum. 2002; 45:514–521. PMID: 12006934.


16. Kolaczkowska E, Seljelid R, Plytycz B. Role of mast cells in zymosan-induced peritoneal inflammation in BALB/c and mast cell-deficient wbb6f1 mice. J Leukoc Biol. 2001; 69:33–42. PMID: 11200065.
17. Perretti M, Solito E, Parente L. Evidence that endogenous interleukin-1 is involved in leukocyte migration in acute experimental inflammation in rats and mice. Agents Actions. 1992; 35:71–78. PMID: 1509980.


18. Hau J, Van Hoosier GL. Hau J, editor. Laboratory animal analgesia, anesthesia, and euthanasia. Handbook of Laboratory Animal Science. 2003. Volume I. Boca Raton: CRC Press;p. 413–457.
19. Nation Research Council. Guide for the Care and Use of Laboratory Animals. 1996. Washington DC: National Academy Press.
20. Coria-Avila GA, Gavrila AM, Menard S, Ismail N, Pfaus JG. Cecum location in rats and the implications for intraperitoneal injections. Lab Anim (NY). 2007; 36:25–30. PMID: 17585354.


21. Miner NA, Koehler J, Greenaway L. Intraperitoneal injection of mice. Appl Microbiol. 1969; 17:250–251. PMID: 5775909.


22. Suzumori N, Katano K, Suzumori K. Peritoneal fluid concentrations of epithelial neutrophil-activating peptide-78 correlate with the severity of endometriosis. Fertil Steril. 2004; 81:305–308. PMID: 14967364.


23. Sakurai F, Terada T, Yasuda K, Yamashita F, Takakura Y, Hashida M. The role of tissue macrophages in the induction of proinflammatory cytokine production following intravenous injection of lipoplexes. Gene Ther. 2002; 9:1120–1126. PMID: 12140741.


24. Thompson JS, Brown SA, Khurdayan V, Zeynalzadedan A, Sullivan PG, Scheff SW. Early effects of tribromoethanol, ketamine/xylazine, pentobarbitol, and isoflurane anesthesia on hepatic and lymphoid tissue in icr mice. Comp Med. 2002; 52:63–67. PMID: 11900415.
25. Person B, Wexner SD. The management of postoperative ileus. Curr Probl Surg. 2006; 43:6–65. PMID: 16412717.


26. McBride WT, Armstrong MA, McBride SJ. Immunomodulation: An important concept in modern anaesthesia. Anaesthesia. 1996; 51:465–473. PMID: 8694161.


27. Bette M, Schlimme S, Mutters R, Menendez S, Hoffmann S, Schulz S. Influence of different anaesthetics on pro-inflammatory cytokine expression in rat spleen. Lab Anim. 2004; 38:272–279. PMID: 15207038.
28. Cash JL, White GE, Greaves DR. Chapter 17. Zymosan-induced peritonitis as a simple experimental system for the study of inflammation. Methods Enzymol. 2009; 461:379–396. PMID: 19480928.


30. Fu ES, Norman JG, Scharf JE, Burdash N. Effect of type of anesthesia and lower-abdominal laparotomy in mice on the cytokine response to acute stress. Reg Anesth. 1996; 21:470–473. PMID: 8896011.
31. Bette M, Schafer MK, van Rooijen N, Weihe E, Fleischer B. Distribution and kinetics of superantigen-induced cytokine gene expression in mouse spleen. J Exp Med. 1993; 178:1531–1539. PMID: 8228806.


32. Heel KA, Hall JC. Peritoneal defences and peritoneum-associated lymphoid tissue. Br J Surg. 1996; 83:1031–1036. PMID: 8869299.


33. Cuzzocrea S, de Sarro G, Costantino G, Mazzon E, Laura R, Ciriaco E, de Sarro A, Caputi AP. Role of interleukin-6 in a non-septic shock model induced by zymosan. Eur Cytokine Netw. 1999; 10:191–203. PMID: 10400825.
34. Yao V, Cooper D, McCauley R, Platell C, Hall J. Bacterial translocation in a non-lethal rat model of peritonitis. Colorectal Dis. 2001; 3:338–344. PMID: 12790957.


35. Steward JP, Ornellas EP, Beernink KD, Northway WH. Errors in the technique of intraperitoneal injection of mice. Appl Microbiol. 1968; 16:1418–1419. PMID: 5676408.


36. Gaines Das R, North D. Implications of experimental technique for analysis and interpretation of data from animal experiments: outliers and increased variability resulting from failure of intraperitoneal injection procedures. Lab Anim. 2007; 41(3):312–320. PMID: 17640458.


37. Svendsen O. Ethics and animal welfare related to in vivo pharmacology and toxicology in laboratory animals. Basic Clin Pharmacol Toxicol. 2005; 97(4):197–199. PMID: 16176551.


38. Smith W. Responses of laboratory animals to some injectable anaesthetics. Lab Anim. 1993; 27:30–39. PMID: 8437433.


39. Zuurbier CJ, Emons VM, Ince C. Hemodynamics of anesthetized ventilated mouse models: aspects of anesthetics, fluid support, and strain. Am J Physiol Heart Circ Physiol. 2002; 282:H2099–H2105. PMID: 12003817.
40. Buitrago S, Martin TE, Tetens-Woodring J, Belicha-Villanueva A, Wilding GE. Safety and efficacy of various combinations of injectable anesthetics in balb/c mice. J Am Assoc Lab Anim Sci. 2008; 47:11–17. PMID: 18210992.
41. Flecknell PA. Laboratory Animal Anaesthesia. 2005. San Diego: Elsevier Academic Press;p. 160–171.
42. Ajuebor MN, Flower RJ, Hannon R, Christie M, Bowers K, Verity A, Perretti M. Endogenous monocyte chemoattractant protein-1 recruits monocytes in the zymosan peritonitis model. J Leukoc Biol. 1998; 63(1):108–116. PMID: 9469480.


43. Doherty NS, Poubelle P, Borgeat P, Beaver TH, Westrich GL, Schrader NL. Intraperitoneal injection of zymosan in mice induces pain, inflammation and the synthesis of peptidoleukotrienes and prostaglandin E2. Prostaglandins. 1985; 30(5):769–789. PMID: 3001831.


44. Sato M, Sano H, Iwaki D, Kudo K, Konishi M, Takahashi H, Takahashi T, Imaizumi H, Asai Y, Kuroki Y. Direct binding of Toll-like receptor 2 to zymosan, and zymosan-induced NF-κB activation and TNF-α secretion are down-regulated by lung collectin surfactant protein A. J Immunol. 2003; 171(1):417–425. PMID: 12817025.


45. Torok K, Nemeth K, Erdo F, Aranyi P, Szekely JI. Measurement and drug induced modulation of interleukin-1 level during zymosan peritonitis in mice. Inflamm Res. 1995; 44(6):248–252. PMID: 7583520.


46. Lemaire LC, Van Lanschot JJ, Stoutenbeek CP, Van Deventer SJ, Wells CL, Gouma DJ. Bacterial translocation in multiple organ failure: cause or epiphenomenon still unproven. Br J Surg. 1997; 84(10):1340–1350. PMID: 9361586.


Table 2
Cytokine levels (pg/mL) quantitated by ELISA in the abdominal lavage fluid after intraperitoneal administration at various time points
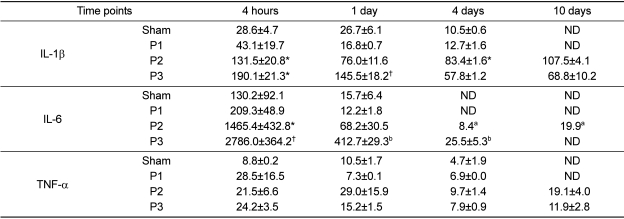