Abstract
Cyclooxygenase-2 (COX-2) is believed to be a multifunctional neural modulator that affects synaptic plasticity in the hippocampus. In the present study, we investigated the differential effects of treadmill exercise on COX-2 immunoreactivity in the dentate gyrus in early and chronic diabetic stages in Zucker diabetic fatty (ZDF) rats and lean control (ZLC) rats. To this end, ZLC and ZDF rats at 6 or 23 weeks of age were put on a treadmill with or without running for 1 h/day for 5 consecutive days at 16-22 m/min for 5 weeks or 12-16 m/min for 7 weeks, respectively. Treadmill exercise in prediabetic and chronic diabetic rats significantly reduced blood glucose levels. In particular, exercise in the prediabetic rat blocked the onset of diabetes. COX-2 immunoreactivity was mainly detected in the granule cell layer of the dentate gyrus and stratum pyramidale of the CA3 region in all groups. COX-2 immunoreactivity was significantly increased in these regions of ZLC and ZDF rats after treadmill exercise in the early diabetic stage. However, COX-2 immunoreactivity was not changed in these regions in ZDF rats after treadmill exercise in the chronic stage. These results suggest that treadmill exercise in diabetic animals in the chronic stage has limited ability to cause plasticity in the dentate gyrus.
Cyclooxygenase (COX), also known as prostaglandin (PG) H synthase, is a rate-limiting enzyme in the production of PG from two isozymes, constitutive COX-1 and inducible COX-2 [1]. COX-2, which is expressed in response to inflammatory stimuli in peripheral tissue [2], plays an essential role in neuroinflammation. COX-2 is also expressed at high levels in discrete populations of neurons within cortical and limbic regions, such as the hippocampus and amygdala [1,3,4]. In this regard, COX-2 is an inflammatory mediator [2], as well as a multifunctional neural modulator that affects synaptic plasticity [5,6].
Diabetes may directly affect the brain because brain insulin receptors, as well as insulin-sensitive glucose transporters, are regionally distributed throughout the central nervous system with differential levels of expression in brain regions subserving affective and cognitive functions [7]. We previously reported that diabetes significantly decreases cellular proliferation and neuroblast differentiation in the dentate gyrus of type-2 diabetic rats [8,9]. Among diabetic animal models, Zucker diabetic fatty (ZDF) rats are characterized by a mutation in the leptin receptor, which results in high levels of circulating leptin, glucose, insulin, and lipids. Diabetic phenotypes in this animal, which appear between 7 and 10 weeks of age, are maintained for at least 6 months [10,11].
Physical exercise is associated with decreases in the risk of cognitive impairment, improvements in learning and memory capability, and improvements in the recovery of brain functions after brain damage [12-15]. Nuclear factor-kappa B (NF-κB) activity was profoundly reduced after chronic exercise in an exercise intensity-dependent manner in Alzheimer's disease mice [16]. Our previous study showed that the effects of exercise on glucose levels in the blood and on cellular proliferation and neuroblast differentiation in the dentate gyrus were different in the early and chronic stages of diabetes [9]. However, there are no comparative studies on the effects of exercise on COX-2 immunoreactivity in rats in the early and chronic stages of diabetes. In the present study, therefore, we investigated changes in COX-2 immunoreactivity in the hippocampus of ZDF rats and lean control (ZLC) rats.
Male and female heterozygote type (Leprfa/+) ZDF rats were purchased from Genetic Models (Indianapolis, ME, USA) and mated. They were housed in a conventional state under adequate temperature (23℃) and humidity (60%) control with a 12-h light/12-h dark cycle, and free accesses to food and water. Purina 5008 rodent diet (7.5% fat) was provided as recommended by Genetic Models Co. (Purina, St. Louis, MO, USA). The procedures for the care and handling of animals conformed to guidelines that are in compliance with current international policies on the handling and caring of animals. These guidelines follow the Guide for the Care and Use of Laboratory Animals issued by the Institute of Laboratory Animal Resources, USA, 1996, and a protocol for it was approved by the Institutional Animal Care and Use Committee (IACUC) of Seoul National University. All of the experiments were designed to minimize the number of animals used and the suffering caused by the procedures used in the present study.
The genotype of Leprfa was determined using the strategy described in our previous study [8]. For effects of exercise on COX-2 immunoreactivity at early and chronic stages, 20 male ZLC and ZDF rats were randomly divided into 4 groups (n=5 per group): sedentary and exercise groups. Running speed and exercise duration was determined according to Lawler's protocol to correspond to 75% of maximal oxygen uptake [17]. At 6 or 23 weeks of age, exercise groups were familiarized with treadmill running on a motorized treadmill (Model 1050 LS Exer3/6; Columbus Instruments, Columbus, OH, USA) for 15 min/day at 15 or 12 m/min for 5 consecutive days, respectively. After familiarization, electrical stimulation to encourage the rats to run was disconnected to avoid pain stress. For early stage diabetes experiments, rats were run for 1 h/day for 5 consecutive days at 16 m/min for 5 weeks and the speeds accelerated 2 m/min per day over 2 weeks. For chronic stage diabetes experiment, rats were run for 1 h/day for 5 consecutive days at 12 m/min for 7 weeks; speeds were accelerated 1 m/min per day over 2 weeks. The control group was put on the treadmill without running for 1 h/day for 5 consecutive days for 5 or 7 weeks. All animals were euthanized at 12 or 30 weeks of age.
Blood (fed glucose, not fast glucose) was sampled by "tail nick" between 9 and 11 AM using a 27G needle before sacrifice. Blood was analyzed immediately after anesthesia using a blood glucose monitor (Ascensia Elite XL Blood Glucose Meter; Bayer, Toronto, ON, Canada).
For histological analysis, animals in each group were anesthetized with 30 mg/kg tiletamine and zolazepam (Zoletil 50®; Virbac, Carros, France) and perfused transcardially with 0.1 M phosphate-buffered saline (PBS, pH 7.4) followed by 4% paraformaldehyde in 0.1 M phosphate-buffer (PB, pH 7.4). Brains were removed and postfixed in the same fixative for 6 hr. The brain tissues were cryoprotected by infiltration with 30% sucrose overnight. The 30-µm-thick brain sections in the coronal plane were serially cut using a cryostat (Leica, Wetzlar, Germany). The sections were collected into six-well plates containing PBS for further process.
To obtain accurate data for immunohistochemistry, free-floating sections were carefully processed under the same conditions. The tissue sections were selected at points from -3.00~ -4.08 mm to bregma [18]. Sections were sequentially treated with 0.3% hydrogen peroxide (H2O2) in PBS for 30 min and 10% normal goat serum in 0.05 M PBS for 30 min. They were then incubated with diluted rabbit anti-COX-2 (1:200, Cayman, Ann Arbor, MI, USA) overnight at room temperature and subsequently exposed to biotinylated goat anti-rabbit IgG and streptavidin peroxidase complex (1:200, Vector, Burlingame, CA, USA). They were then visualized by reaction with 3,3'-diaminobenzidine tetrachloride (Sigma, St. Louis, MO, USA) in 0.1 M Tris-HCl buffer (pH 7.2) and mounted on gelatin-coated slides. Sections were mounted in Canada Balsam (Kanto, Tokyo, Japan) following dehydration.
Analysis of a region of interest (ROI) in the hippocampus was performed using an image analysis system. Images were calibrated in an array of 512×512 pixels corresponding to a tissue area of 140×140 µm (×40 primary magnification). Each pixel resolution was 256 gray levels. The intensity of COX-2 immunoreactivity was evaluated by means of a relative optical density (ROD), which was obtained after the transformation of the mean gray level using the formula: ROD=log (256/mean gray level). The ROD of the background was determined in unlabeled portions and the value subtracted for correction, yielding high ROD values in the presence of preserved structures and low values after structural loss using NIH Image 1.59 software. A ratio of ROD values was calculated as a %.
The data shown here represent the means of experiments performed for each experimental area. Differences among means were statistically analyzed by two-way analysis of variance followed by Duncan's post-hoc analysis in order to elucidate differences between 1) sedentary vs exercise groups, 2) 12 vs 30 weeks old groups, and 3) ZLC vs ZDF groups.
In 12-week-old ZLC and ZDF rats that were sedentary (Co12-ZLC and Co12-ZDF), blood glucose levels were 5.89 mmol/L and 21.3 mmol/L, respectively. In 12-week-old rats in that exercised (Ex12-ZLC and Ex12-ZDF), blood glucose levels were similar to those in the Co12-ZLC group (Figure 1).
In 30-week-old ZLC and ZDF rats that were sedentary (Co30-ZLC and Co30-ZDF), blood glucose levels were 6.31 mmol/L and 29.9 mmol/L, respectively. In 30-week-old rats that exercised (Ex30-ZLC and Ex30-ZDF), blood glucose levels were 6.18 mmol/L and 20.9 mmol/L, respectively (Figure 1).
In the Co12-ZLC group, COX-2 immunoreactivity was detected in the granule cells and in the CA4 region of the dentate gyrus (Figure 2A). COX-2 immunoreactivity was mainly observed in the dorsal blade of the dentate gyrus. In the Co12-ZDF group, a few COX-2-immunoreactive cells were detected in the granule cell layer and CA4 region (Figure 2B). Overall, COX-2 immunoreactivity was significantly decreased in the Co12-ZDF group compared to the Co12-ZLC group (Figure 4A). In the Ex12-ZLC group, COX-2 immunoreactivity was significantly increased in the dentate gyrus and CA4 region compared to the Co12-ZLC group (Figures 2C and 4A). In the Ex12-ZLC group, COX-2-immunoreactive cells were few in the ventral blade of the dentate gyrus (Figure 2C). In the Ex12-ZDF group, COX-2 immunoreactivity was significantly decreased in the dentate gyrus and CA4 region compared to the Co12-ZDF group (Figures 2D and 4A), but COX-2 immunoreactivity was significantly increased compared to the Co12-ZDF group (Figure 4A). In this group, COX-2 immunoreactivity was similar to the Co12-ZLC group (Figure 4A).
In the Co30-ZLC group, COX-2 immunoreactivity in the dentate gyrus and CA4 region was similar to that in the Co12-ZLC group (Figures 2E and 4A). In the Co30-ZDF group, COX-2 immunoreactivity in the dentate gyrus and CA4 region was significantly decreased compared to that in the Co30-ZLC group (Figures 2F and 4A). In this group, COX-2 immunoreactivity was similar to that in the Co12-ZDF group (Figure 4A). In the Ex30-ZLC group, COX-2 immunoreactivity in the dentate gyrus and CA4 region was slightly, but not significantly, increased compared to that in the Co30-ZLC group (Figures 2G and 4A). In this group, COX-2 immunoreactivity was significantly decreased compared to that in the Ex12-ZLC group (Figure 4A). In the Ex30-ZDF group, COX-2 immunoreactivity was similar to that in the Co30-ZLC and Ex30-ZLC group as well as in the Ex12-ZDF group (Figures 2H and 4A).
In the Co12-ZLC group, COX-2 immunoreactivity was mainly detected in the pyramidal cells of the stratum pyramidale (Figure 3A). In the Co12-ZDF group, COX-2 immunoreactivity in the hippocampal CA3 region was significantly decreased compared to that in the Co12-ZLC group (Figures 3B and 4B). In the Ex12-ZLC group, COX-2 immunoreactivity was significantly increased compared to that in the Co12-ZLC group (Figures 3C and 4B). In the Ex12-ZDF group, COX-2 immunoreactivity in the hippocampal CA3 region was significantly decreased compared to that in the Ex12-ZLC group, but COX-2 immunoreactivity was significantly increased compared to that in the Co12-ZDF group (Figures 3D and 4B).
In the Co30-ZLC group, COX-2 immunoreactivity in the hippocampal CA3 region was similar to that in the Co12-ZLC group (Figures 3E and 4B). In the Co30-ZDF group, COX-2 immunoreactivity in the hippocampal CA3 region was significantly decreased compared to that in the Co30-ZLC group, and it was similar to that in the Co12-ZDF group (Figures 3F and 4B). In the Ex30-ZLC group, COX-2 immunoreactivity was significantly increased compared to that in the Co30-ZLC group and decreased compared to that in the Ex12-ZLC group (Figures 3G and 4B). In the Ex30-ZDF group, COX-2 immunoreactivity in the hippocampal CA3 region was significantly decreased compared to that in the Ex30-ZLC group as well as in the Ex12-ZDF group. In this group, COX-2 immunoreactivity was similar to that in the Co30-ZLC group (Figures 3H and 4B).
COX-2 is a key enzyme in the conversion of arachidonic acid to PGs and thromboxanes, and it is a target enzyme for nonsteroidal anti-inflammatory drugs [1,19]. Recently, COX-2 has been shown to participate in synaptic transmission and plasticity through its downstream metabolite PGE2 [20-24] and through both 2-arachidonoylglycerol and PGE2 [25]. In the present study, we observed the effects of diabetes and/or exercise on COX-2 immunoreactivity in rats because diabetes has been shown to impair synaptic plasticity and learning and memory [8,26-28]. COX-2 immunoreactivity was mainly detected in the hippocampal CA3 region and dentate gyrus. The staining profiles in the rat brains were similar to those in previous reports [1,29-31].
In the present study, COX-2 immunoreactivity was prominently decreased in these regions in the early stage of diabetes, but not in the chronic stage of diabetes. This result is contradictory to a previous study that showed that COX-2 was upregulated in the cerebral cortex of rats fed a high-fat diet [32]. This difference may be associated with different functions of COX-2 in the brain. COX-2 that is expressed in the hippocampus is closely related to neurogenesis in the brain. COX-2 was shown to participate in the proliferation of neural progenitor cells following ischemic insults [33,34]. COX-2 inhibitors significantly reduced cellular proliferation and neuroblast differentiation in mice [35] and rats [31] and suppressed increases in neurogenesis in the hippocampus that followed acute global ischemia [36]. COX-2-knockout mice exhibited impaired neurogenesis in the hippocampus [34]. In addition, the administration of COX-2 inhibitor significantly reduced microglia activation and decreased hippocampal neurogenesis in epilepsy model [37]. This result is supported by our previous study that showed that a reduction of neuroblast differentiation was more pronounced at the early stage of diabetes than at the chronic stage of diabetes [9]. However, COX-2 immunoreactivity was increased in hippocampus of Alzheimer diseases [38,39]. This discrepancy may be associated with the functions of COX-2 in the hippocampus. Several studies demonstrated that COX-2 immunoreactivity was significantly increased in the hippocampus of neurological disorders such as stroke, depression, epilepsy, schizophrenia, and amyotrophic lateral sclerosis [40-46]. In these models, COX-2 may function as the inflammatory mediators, while in the present study, COX-2 is closely related to synaptic plasticity in the hippocampus.
In this study, COX-2 immunoreactivity was significantly increased in the dentate gyrus and hippocampal CA3 region in all groups. However, there were some differences between control and diabetic animals. Control rats at 12 or 30 weeks of age showed similar increases in the levels of COX-2 immunoreactivity due to treadmill exercise, while in the diabetic rats, COX-2 immunoreactivity was only increased at 12 weeks of age. At 30 weeks of age, COX-2 immunoreactivity was similar between sedentary and exercised groups. This suggests that exercise in the chronic diabetic stage has limited effects on COX-2 immunoreactivity. Exercise at an early stage of diabetes did not increase blood glucose levels, while exercise in the chronic stage of diabetes only decreased the blood glucose levels.
In conclusion, treadmill exercise significantly increases COX-2 immunoreactivity in the dentate gyrus and hippocampal CA3 region in the early stages of diabetes but not in the chronic stage of diabetes. These results suggest that treadmill exercise enhances synaptic plasticity in the hippocampus in the early stage of diabetes and not in the chronic stage of diabetes.
Acknowledgments
The authors would like to thank Mr. Seung Uk Lee and Ms. Hyun Sook Kim for their technical help in this study. This work was supported by a National Research Foundation of Korea Grant funded by the Korean Government (MEST), Republic of Korea (2010-0007712).
References
1. Yamagata K, Andreasson KI, Kaufmann WE, Barnes CA, Worley PF. Expression of a mitogen-inducible cyclooxygenase in brain neurons: regulation by synaptic activity and glucocorticoids. Neuron. 1993; 11:371–386. PMID: 8352945.


2. Vane JR, Bakhle YS, Botting RM. Cyclooxygenases 1 and 2. Annu Rev Pharmacol Toxicol. 1998; 38:97–120. PMID: 9597150.


3. Kaufmann WE, Andreasson KI, Isakson PC, Worley PF. Cyclooxygenases and the central nervous system. Prostaglandins. 1997; 54:601–624. PMID: 9373877.


4. Yamashita A, Kunimatsu T, Yamamoto T, Yoshida K. Hypothermic, but not normothermic, ischemia causes a drastic increase in cyclooxygenase-2 immunoreactive granule cells in rat dentate gyrus after 4 hours of ischemic reperfusion. Arch Histol Cytol. 2007; 70:197–205. PMID: 18079588.


5. Kaufmann WE, Worley PF, Pegg J, Bremer M, Isakson P. COX-2, a synaptically induced enzyme, is expressed by excitatory neurons at postsynaptic sites in rat cerebral cortex. Proc Natl Acad Sci USA. 1996; 93:2317–2321. PMID: 8637870.


6. Bazan NG. COX-2 as a multifunctional neuronal modulator. Nat Med. 2001; 7:414–415. PMID: 11283664.


7. Craft S, Watson GS. Insulin and neurodegenerative disease: shared and specific mechanisms. Lancet Neurol. 2004; 3:169–178. PMID: 14980532.


8. Hwang IK, Yi SS, Kim YN, Kim IY, Lee IS, Yoon YS, Seong JK. Reduced hippocampal cell differentiation in the subgranular zone of the dentate gyrus in a rat model of type II diabetes. Neurochem Res. 2008; 33:394–400. PMID: 17712629.


9. Hwang IK, Yi SS, Song W, Won MH, Yoon YS, Seong JK. Effects of age and treadmill exercise in chronic diabetic stages on neuroblast differentiation in a rat model of type 2 diabetes. Brain Res. 2010; 1341:63–71. PMID: 20005869.


10. Vora JP, Zimsen SM, Houghton DC, Anderson S. Evolution of metabolic and renal changes in the ZDF/Drt-fa rat model of type II diabetes. J Am Soc Nephrol. 1996; 7:113–117. PMID: 8808117.


11. Etgen GJ, Oldham BA. Profiling of Zucker diabetic fatty rats in their progression to the overt diabetic state. Metabolism. 2000; 49:684–688. PMID: 10831184.


12. Cotman CW, Berchtold NC. Exercise: a behavioral intervention to enhance brain health and plasticity. Trends Neurosci. 2002; 25:295–301. PMID: 12086747.


13. Tillerson JL, Caudle WM, Reveron ME, Miller GW. Exercise induces behavioral recovery and attenuates neurochemical deficits in rodent models of Parkinson's disease. Neuroscience. 2003; 119:899–911. PMID: 12809709.


14. Gobbo OL, O'Mara SM. Exercise, but not environmental enrichment, improves learning after kainic acid-induced hippocampal neurodegeneration in association with an increase in brain-derived neurotrophic factor. Behav Brain Res. 2005; 159:21–26. PMID: 15794993.


15. Van Praag H. Exercise and the brain: something to chew on. Trends Neurosci. 2009; 32:283–290. PMID: 19349082.


16. Leem YH, Lee YI, Son HJ, Lee SH. Chronic exercise ameliorates the neuroinflammation in mice carrying NSE/htau23. Biochem Biophys Res Commun. 2011; 406:359–365. PMID: 21329662.
17. Song W, Kwak HB, Lawler JM. Exercise training attenuates age-induced changes in apoptotic signaling in rat skeletal muscle. Antioxid Redox Signal. 2006; 8:517–528. PMID: 16677096.


18. Paxinos G, Watson C. The Rat Brain in Stereotaxic Coordinates. 2007. Amsterdam: Elsevier Academic Press.
19. Graham SH, Hickey RW. Cyclooxygenases in central nervous system diseases: a special role for cyclooxygenase 2 in neuronal cell death. Arch Neurol. 2003; 60:628–630. PMID: 12707081.
20. Chen C, Magee JC, Bazan NG. Cyclooxygenase-2 regulates prostaglandin E2 signaling in hippocampal long-term synaptic plasticity. J Neurophysiol. 2002; 87:2851–2857. PMID: 12037188.
21. Murray HJ, O'Connor JJ. A role for COX-2 and p38 mitogen activated protein kinase in long-term depression in the rat dentate gyrus in vitro. Neuropharmacology. 2003; 44:374–380. PMID: 12604095.


22. Chen C, Bazan NG. Endogenous PGE2 regulates membrane excitability and synaptic transmission in hippocampal CA1 pyramidal neurons. J Neurophysiol. 2005; 93:929–941. PMID: 15653788.
23. Sang N, Zhang J, Marcheselli V, Bazan NG, Chen C. Postsynaptically synthesized prostaglandin E2 modulates hippocampal synaptic transmission via a presynaptic PGE2 EP2 receptor. J Neurosci. 2005; 25:9858–9870. PMID: 16251433.
24. Akaneya Y, Tsumoto T. Bidirectional trafficking of prostaglandin E2 receptors involved in long-term potentiation in visual cortex. J Neurosci. 2006; 26:10209–10221. PMID: 17021176.


25. Yang H, Zhang J, Andreasson K, Chen C. COX-2 oxidative metabolism of endocannabinoids augments hippocampal synaptic plasticity. Mol Cell Neurosci. 2008; 37:682–695. PMID: 18295507.


26. Grillo CA, Piroli GG, Wood GE, Reznikov LR, McEwen BS, Reagan LP. Immunocytochemical analysis of synaptic proteins provides new insights into diabetes-mediated plasticity in the rat hippocampus. Neuroscience. 2005; 136:477–486. PMID: 16226381.


27. Artola A. Diabetes-, stress- and ageing-related changes in synaptic plasticity in hippocampus and neocortex - the same metaplastic process? Eur J Pharmacol. 2008; 585:153–162. PMID: 18395200.


28. Reisi P, Babri S, Alaei H, Sharifi MR, Mohaddes G, Lashgari R. Effects of treadmill running on short-term pre-synaptic plasticity at dentate gyrus of streptozotocin-induced diabetic rats. Brain Res. 2008; 1211:30–36. PMID: 18433735.


29. Breder CD, Dewitt D, Kraig RP. Characterization of inducible cyclooxygenase in rat brain. J Comp Neurol. 1995; 355:296–315. PMID: 7608344.


30. Kim SK, Choi JW, Hwang IK, Yoo DY, Yoo KY, Lee CH, Choi JH, Shin HC, Seong JK, Yoon YS, Won MH. Species-difference of cyclooxygenase-2 in the hippocampus of rodents. J Vet Med Sci. 2010; 72:1153–1158. PMID: 20424394.


31. Hwang IK, Yi SS, Yoo KY, Park OK, Yan B, Kim IY, Kim YN, Song W, Moon SM, Won MH, Seong JK, Yoon YS. Effects of treadmill exercise on cyclooxygenase-2 in the hippocampus in type 2 diabetic rats: correlation with the neuroblasts. Brain Res. 2010; 1341:84–92. PMID: 20219444.


32. Zhang X, Dong F, Ren J, Driscoll MJ, Culver B. High dietary fat induces NADPH oxidase-associated oxidative stress and inflammation in rat cerebral cortex. Exp Neurol. 2005; 191:318–325. PMID: 15649487.


33. Uchida K, Kumihashi K, Kurosawa S, Kobayashi T, Itoi K, Machida T. Stimulatory effects of prostaglandin E2 on neurogenesis in the dentate gyrus of the adult rat. Zoolog Sci. 2002; 19:1211–1216. PMID: 12499663.
34. Sasaki T, Kitagawa K, Sugiura S, Omura-Matsuoka E, Tanaka S, Yagita Y, Okano H, Matsumoto M, Hori M. Implication of cyclooxygenase-2 on enhanced proliferation of neural progenitor cells in the adult mouse hippocampus after ischemia. J Neurosci Res. 2003; 72:461–471. PMID: 12704808.


35. Goncalves MB, Williams EJ, Yip P, Yáñez-Muñoz RJ, Williams G, Doherty P. The COX-2 inhibitors, meloxicam and nimesulide, suppress neurogenesis in the adult mouse brain. Br J Pharmacol. 2010; 159:1118–1125. PMID: 20136845.


36. Kumihashi K, Uchida K, Miyazaki H, Kobayashi J, Tsushima T, Machida T. Acetylsalicylic acid reduces ischemia-induced proliferation of dentate cells in gerbils. Neuroreport. 2001; 12:915–917. PMID: 11303759.


37. Jung KH, Chu K, Lee ST, Kim J, Sinn DI, Kim JM, Park DK, Lee JJ, Kim SU, Kim M, Lee SK, Roh JK. Cyclooxygenase-2 inhibitor, celecoxib, inhibits the altered hippocampal neurogenesis with attenuation of spontaneous recurrent seizures following pilocarpine-induced status epilepticus. Neurobiol Dis. 2006; 23:237–246. PMID: 16806953.


38. Andreasson KI, Savonenko A, Vidensky S, Goellner JJ, Zhang Y, Shaffer A, Kaufmann WE, Worley PF, Isakson P, Markowska AL. Age-dependent cognitive deficits and neuronal apoptosis in cyclooxygenase-2 transgenic mice. J Neurosci. 2001; 21:8198–8209. PMID: 11588192.


39. Ho L, Purohit D, Haroutunian V, Luterman JD, Willis F, Naslund J, Buxbaum JD, Mohs RC, Aisen PS, Pasinetti GM. Neuronal cyclooxygenase 2 expression in the hippocampal formation as a function of the clinical progression of Alzheimer disease. Arch Neurol. 2001; 58:487–492. PMID: 11255454.


40. Cassano P, Hidalgo A, Burgos V, Adris S, Argibay P. Hippocampal upregulation of the cyclooxygenase-2 gene following neonatal clomipramine treatment (a model of depression). Pharmacogenomics J. 2006; 6:381–387. PMID: 16568149.


41. Choi JS, Kim HY, Chun MH, Chung JW, Lee MY. Differential regulation of cyclooxygenase-2 in the rat hippocampus after cerebral ischemia and ischemic tolerance. Neurosci Lett. 2006; 393:231–236. PMID: 16253424.


42. Lukiw WJ, Cui JG, Musto AE, Musto BC, Bazan NG. Epileptogenesis in diacylglycerol kinase epsilon deficiency up-regulates COX-2 and tyrosine hydroxylase in hippocampus. Biochem Biophys Res Commun. 2005; 338:77–81. PMID: 16137646.


43. Yokota O, Terada S, Ishihara T, Nakashima H, Kugo A, Ujike H, Tsuchiya K, Ikeda K, Saito Y, Murayama S, Ishizu H, Kuroda S. Neuronal expression of cyclooxygenase-2, a proinflammatory protein, in the hippocampus of patients with schizophrenia. Prog Neuropsychopharmacol Biol Psychiatry. 2004; 28:715–721. PMID: 15276698.


44. Yokota O, Terada S, Ishizu H, Ishihara T, Nakashima H, Kugo A, Tsuchiya K, Ikeda K, Hayabara T, Saito Y, Murayama S, Uda K, Checler F, Kuroda S. Increased expression of neuronal cyclooxygenase-2 in the hippocampus in amyotrophic lateral sclerosis both with and without dementia. Acta Neuropathol. 2004; 107:399–405. PMID: 14991384.


45. Tu B, Bazan NG. Hippocampal kindling epileptogenesis upregulates neuronal cyclooxygenase-2 expression in neocortex. Exp Neurol. 2003; 179:167–175. PMID: 12618123.
46. Miettinen S, Fusco FR, Yrjänheikki J, Keinänen R, Hirvonen T, Roivainen R, Närhi M, Hökfelt T, Koistinaho J. Spreading depression and focal brain ischemia induce cyclooxygenase-2 in cortical neurons through N-methyl-D-aspartic acid-receptors and phospholipase A2. Proc Natl Acad Sci USA. 1997; 94:6500–6505. PMID: 9177247.
Figure 1
Change in blood glucose levels in 12-week-old Zucker lean control (ZLC) and Zucker diabetic fatty (ZDF) rats in the sedentary groups (Co12-ZLC and Co12-ZDF), in the 12-week-old rats in the exercised groups (Ex12-ZLC and Ex12-ZDF), in the 30-week-old rats in the sedentary groups (Co30-ZLC and Co30-ZDF), and in the 30-week-old rats in the exercised groups (Ex30-ZLC and Ex30-ZDF rats; n=5 per group; aP<0.05, significantly different from control group; bP<0.05, significantly different from the ZLC group; cP<0.05, significantly different from 12-week-old age group). The bars indicate the SEM.
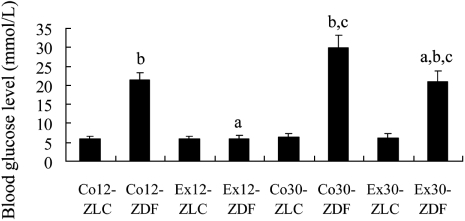
Figure 2
Cyclooxygenase-2 (COX-2) immunoreactivity in the dentate gyrus of Co12-ZLC (A), Co12-ZDF (B), Ex12-ZLC (C), Ex12-ZDF (D), Co30-ZLC (E), Co30-ZDF (F), Ex30-ZLC (G) and Ex30-ZDF (H) rats. COX-2 immunoreactivity is mainly detected in the granule cell layer (GCL, asterisk) of the dentate gyrus. Note that COX-2 immunoreactivity is strong in the exercised group except in the Ex30-ZDF group. PL, polymorphic layer; ML, molecular layer. Scale bar=100 µm.
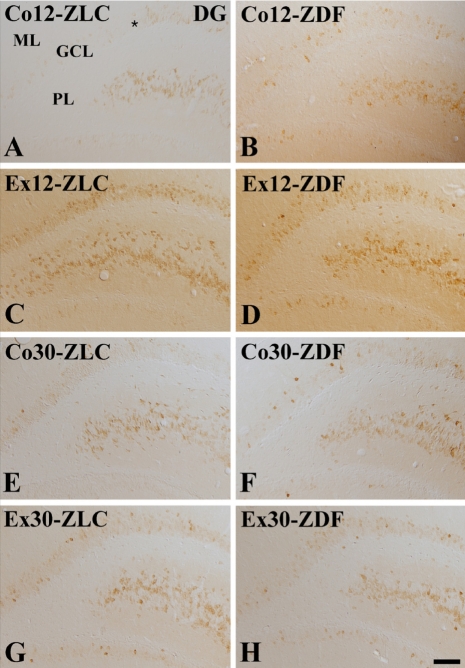
Figure 3
COX-2 immunoreactivity in the hippocampal CA3 region of Co12-ZLC (A), Co12-ZDF (B), Ex12-ZLC (C), Ex12-ZDF (D), Co30-ZLC (E), Co30-ZDF (F), Ex30-ZLC (G) and Ex30-ZDF (H) rats. COX-2 immunoreactivity is observed in the stratum pyramidal (SP, asterisk). Note that COX-2 immunoreactivity is strong in most exercised groups except the Ex30-ZDF group. SO, stratum oriens; SR, stratum radiatum. Scale bar=100 µm.

Figure 4
Relative optical densities (ROD) as percentage values of COX-2 in the dentate gyrus (A), and CA3 (B) of Co12-ZLC, Co12-ZDF, Ex12-ZLC, Ex12-ZDF, Co30-ZLC, Co30-ZDF, Ex30-ZLC and Ex30-ZDF rats. Differences among the means were analyzed statistically by two-way analysis of variance followed by Duncan's new multiple range method (n=5 per group; aP<0.05, significantly different from control group; bP<0.05, significantly different from the ZLC group; cP<0.05, significantly different from the 12-week-old group). Bars indicate the SEM.
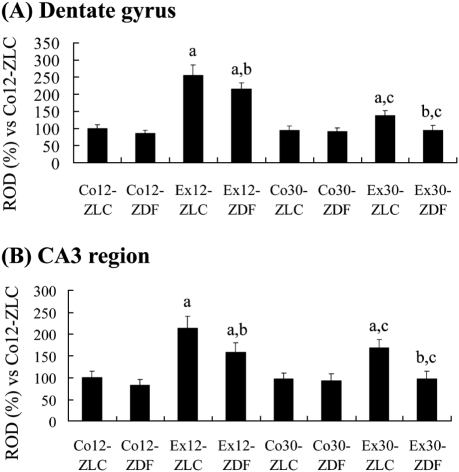