Abstract
Current strategies to accelerate hematopoietic reconstitution after transplantation include transplantation of greater numbers of hematopoietic stem/progenitor cells (HSPCs) or ex vivo expansion of harvested HSPCs before transplant. However, the number of cells available for transplantation is usually low, and strategies to expand HSPCs and maintain equivalent engraftment capability ex vivo are limited. We noted that activated granulocyte-derived cationic peptides positively primed responsiveness of HSPCs to a CXCL12 gradient. Accordingly, we noted that accelerated homing/engraftment of β-defensin-2, a well-known antimicrobial cationic peptide, primed bone marrow nucleated cells (BMNCs) compared to normal BMNCs after transplantation into lethally irradiated recipients. We envision that small cationic peptides, which primarily possess antimicrobial functions and are harmless to mammalian cells, could be applied to prime HSPCs before transplantation. This novel approach would be particularly important in cord blood transplantation, where the number of HSPCs available for transplantation is usually limited.
Hematopoietic stem/progenitor cells (HSPCs) circulate in peripheral blood (PB) under steady state conditions at very low levels to keep a pool of stem cells in balance in the bone marrow (BM) microenvironment [1-4]. Therefore, the bloodstream might be the "highway" by which HSPCs relocate between circulation in blood vessels and lodgment in BM endosteal and endothelial niches.
HSPCs can be mobilized from BM into PB during tissue injury [5,6], as well as after treatment of several mobilizing agents. Granulocyte colony-stimulating factor (G-CSF) is currently the most frequently used drug that may efficiently mobilize HSPCs after a few consecutive daily injections [7-9]. On the other hand, the collective term 'homing' refers to the early stages of BM seeding by HSPCs after transplantation, which precedes engraftment, proliferation, and differentiation of these cells into the hematopoietic lineage. In general, homing, engraftment, and expansion of HSPCs after transplantation are closely related processes [10,11].
Transplantation of HSPCs is dependent on the successful homing, engraftment, and repopulation of stem cells in the BM [12,13]. Stem cell homing through the circulatory system to the BM is the critical first step in this process.
The interaction of CXCL12 with its receptor CXCR4, which is expressed in HSPCs, has been implicated as a primary axis governing HSPC homing and engraftment into the BM following transplantation [14-16]. Current strategies to accelerate hematopoietic reconstitution after transplantation include transplantation of greater numbers of HSPCs or ex vivo expansion of harvested HSPCs before transplant. However, the number of cells available for transplantation is usually low, and strategies to expand HSPCs and maintain equivalent engraftment capability ex vivo are limited [17,18].
The migration of HSPCs toward a CXCL12 gradient has been correlated with the repopulating potential of transplanted HPSCs. Indeed, it has been demonstrated that successful homing and engraftment of HSPCs is significantly reduced if the CXCR4-CXCL12 axis is impeded [19].
Of note, it has been shown that leukapheresis products (i.e., C3 liquid-phase cleavage fragments, C3a) are known to enhance responsiveness of HSPCs to a CXCL12 gradient [20,21]. The molecular explanation of this phenomenon was that C3a increases CXCR4 incorporation into membrane lipid rafts, thus facilitating its more effective assembly with downstream signaling molecules [22].
In fact, C3a is one of the antimicrobial cationic peptides (AMPs), which have antimicrobial activity [23]. AMPs have been demonstrated to kill bacteria, enveloped viruses, fungi, and even transformed or cancerous cells without affecting the viability of eukaryotic cells. Selective effects of AMPs, like membrane perturbation of eukaryotic cells and killing of prokaryotic cells, are known to be dependent on the characteristics of membranes, such as the presence of cholesterol and membrane electric charge [24].
In addition, leukapheresis products which are enriched in activated granulocytes are known to release several cationic peptides and activate complement cascades [13]. We recently noted that activated granulocyte-derived cationic peptide positively primes responsiveness of murine HSPCs to a CXCL12 gradient [25].
Based on these data, we hypothesized that the use of cationic peptides as priming drugs might also enhance homing and engraftment of HSPCs after transplantation. In this study, we tested if human β defensin-2 (hBD-2), a well-known antimicrobial cationic peptide released from activated granulocytes, enhances the homing and engraftment of HSPCs after transplantation.
C57BL/6 mice were adopted at 1-2 weeks and used for experiments at age 6 to 8 weeks. Animal studies were approved by the Animal Care and Use Committee of the Institute of Laboratory Animal Resources, Seoul National University.
BMNCs were prepared by flushing femurs and tibias of pathogen-free, 6- to 8-week-old mice without enzymatic digestion. They were lysed with BD Pharm Lyse buffer (BD Biosciences, San Jose, CA, US) to remove red blood cells (RBCs), washed, and resuspended in appropriate media for further analysis.
The trans-well migration assay was performed as described elsewhere [25]. Briefly, unless otherwise indicated, RBC-lysed BMNCs from C57BL/6 mice were resuspended in assay media (RPMI containing 0.5% bovine serum albumin) and equilibrated for 30 min at 37℃. Six hundred-fifty microliters of assay media in the presence or absence of CXCL12 (50-300 ng/mL, PeproTech, Rocky Hill, NJ, USA) was added to the lower chambers of a Trans-well 24-well plate (Costar Corning, Cambridge, MA, USA). hBD-2 was purchased from Prospec (East Brunswick, NJ, USA). Aliquots of normal or 30 min hBD-2-exposed cell suspension (1×106 cells/100 µL) were loaded onto the upper chambers with 5 µm-pore filters, which were incubated for 3 hours at 37℃, 95% humidity, and 5% CO2. Cells from the lower chambers were scored using FACS analysis for migration of BMNCs to test reagents. The results are presented as a migration index, i.e., fold increase of cell numbers migrated toward the lower well compared to control media. In some experiments, migrated cells harvested from the lower chambers were plated in semisolid clonogenic assays supplemented with cytokines and were stimulated to grow colony-forming units-granulocyte-macrophage (CFU-GM) and colony-forming units-macrophage (CFU-M) as described elsewhere [25].
Red blood cells were lysed with BD Pharm Lyse buffer. Nucleated cells were subsequently washed twice and used for CFU-GM and/or CFU-M colonies. BMNCs were resuspended in human methylcellulose base media provided by the manufacturer (R&D Systems, Minneapolis, MN, USA) supplemented with 25 ng/mL recombinant murine granulocyte macrophage colony-stimulating factor (mGM-CSF) and 10 ng/mL recombinant murine interleukin-3 (mIL-3) for CFU-GM, 10 ng/mL recombinant murine macrophage stimulating factor (mM-CSF) for CFU-M. Cultures were incubated for 7 days, at which time they were scored for the number of CFU-GM colonies under an inverted microscope.
Western blot analysis was performed on extracts prepared from mouse EL4 cell lines that had been kept in RPMI medium containing 0.5% bovine serum albumin (BSA) to render the cells quiescent. Cells were stimulated with either a low or high concentration of CXCL12 (50 ng/mL or 300 ng/mL) in the presence or absence of hBD-2 for 90 sec at 37℃ and then lysed for 10 min on ice in M-Per lysing buffer (Pierce, Rockford, IL, USA) containing protease and phosphatase inhibitors (Sigma, St. Louis, MO, USA). Extracted proteins were separated on a 10% sodium dodecyl sulfate polyacrylamide gel electrophoresis (SDS-PAGE) gel and then transferred to a nitrocellulose membrane (Hybond ECL; Amersham Life Sciences, Little Chalfont, United Kingdom). Phosphorylation of 44/42 MAPK protein was detected by protein immunoblotting using mouse monoclonal 44/42 phospho-specific MAPK antibody (New England Biolabs, Beverly, MA, USA), with horseradish peroxidase conjugated goat anti-mouse immunoglobulin G (Santa Cruz Biotechnology, Santa Cruz, CA, USA) as secondary antibody. Equal loading in the lanes was evaluated by stripping the blots and reprobing them with the appropriate monoclonal or polyclonal antibodies. The membranes were developed with an ECL reagent (Amersham Life Sciences), dried, and exposed to film (HyperFilm; Amersham Life Sciences).
Wild-type BM stromal cells were grown to confluence, and normal or 30 min hBD-2-exposed BMNCs were subsequently plated over BM-derived stroma feeder layers established in well plates. Five minutes later, plates were gently shaken, and non-adherent cells were discarded. Subsequently, the cultures were trypsinized and stimulated to grow CFU-GM colonies as mentioned earlier. The number of colonies was scored under an inverted microscope.
To test whether hBD-2 priming enhanced homing and engraftment of transplanted HSPCs, we used a short-homing assay to evaluate lodging of HSPCs to the spleen and bone marrow. Normal recipient mice were lethally irradiated (950 cGy) and 24 hours later were transplanted with 5×105 normal or 30 min hBD-2-exposed BMNCs by tail vein injection. At 18 hours or 12 days after BMNC transplantation, spleens were removed and fixed for colony forming units-spleen (CFU-S) count. At the same time, BM were harvested from the femurs and plated in methylcellulose cultures and stimulated to grow CFU-GM colonies. CFU-S was also counted.
To optimize the trans-well migration assay we tested different doses of CXCL12 using a 5 µm pore sized trans-well. CXCL12 directly chemoattracted not only BMNCs (Figure 1A), but also CFU-GM progenitor cells (Figure 1B) in a dose-dependent manner. However, CXCL12 at a concentration lower than 25 ng/mL did not chemoattract either BMNCs or CFU-GM progentitor cells efficiently. Significant migrations of BMNCs to CXCL12 were shown in concentrations of 50 ng/mL or higher. Although 50 ng/mL of CXCL12 is relatively high compared to its physiological plasma concentration [26], we chose 50 ng/mL of CXCL12 as the standard concentration for our experiments based on the results obtained from our optimization assay.
The antimicrobial cationic peptide hBD-2, which is known to be released from activated human granulocytes, enhanced responsiveness of BMNCs to a CXCL12 gradient. As expected, the migration of hBD-2-exposed BMNCs to a CXCL12 gradient was significantly enhanced compared to that of normal BMNCs (Figures 2A and 2B). This accelerated migration of BMNCs and CFU-GM progenitor cells to a CXCL12 gradient was dependent on the hBD-2 concentration used. However, hBD-2 itself did not show a direct chemotactic effect on BMNCs (Figure 2C) and CFU-GM progenitor cells (Figure 2D). Considering hBD-2 is known to use CCR6 for signal transduction, the hBD-2 priming effect was not a receptor-mediated phenomenon. MIP-3α, which is known as an agonist for CCR6, did not enhance migration of BMNCs to the CXCL12 gradient (data not shown). These results strongly support that enhanced migration of hBD-2-primed cells might be mediated by enhancing incorporation of CXCR4 into lipid rafts as similar to what was shown in C3a primed cells [20,21].
We tested if enhanced CXCR4 incorporation into membrane lipid rafts in the presence of hBD-2 is better connected to downstream signaling machinery and can respond robustly to a CXCL12 gradient, as a previous report showed that the supernatants of leukapheresis products increased p42/44 MAPK phosphorylation mediated by enhancing incorporation of CXCR4 into membrane lipid rafts [20,21].
We noticed that hBD-2 itself does not induce phosphorylation of p42/44 MAPK but enhanced p42/44 MAPK phosphorylation induced by CXCL12 in EL4 cells. CXCL12-induced phosphorylation of p42/44 MAPK was strongly enhanced in hBD-2-primed cells (Figure 3A). This also strongly supports that the priming effect of hBD-2 is not mediated by receptor-ligand interactions.
We questioned the effects of priming agents on other processes essential for the engraftment of HSPCs, as they must adhere to the BM stroma to proliferate and differentiate. To address this question further, we carried out in vitro adhesion experiments with BMNCs. We found that hBD-2-exposed CFU-GM progenitor cells showed increased adhesion (Figure 3B). We suggest that hBD-2, by enhancing responsiveness of HSPCs to a CXCL12 gradient, may directly modulate HSPC homing.
Based on our in vitro results showing enhanced migration and adhesion, we examined whether hBD-2-primed BMNCs engraft faster than normal BMNCs. As summarized in Table 1, hBD-2-primed BMNCs homed better to the BM and spleen of lethally irradiated recipient mice. CFU-GM progenitor cells at 18 hours after BMNC transplantation were increased more than two fold in the femur of hBD-2-primed BMNC transplanted group compared to the normal BMNC transplanted group (data not shown). No CFU-GM or CFU-S was recovered from lethally irradiated control mice that did not receive BMNCs.
Finally, we evaluated the safety of hBD-2 exposure on hematopoietic progenitor cells. BMNCs (5×105/mL) were incubated for 60 min at 37 CO2 incubator in the absence or presence of hBD-2 at 0.12, 0.25, or 0.50 µg/mL concentrations. These cells were subsequently examined via in vitro colony forming assays for the number of CFU-GM and CFU-M progenitor cells. As expected, hBD-2 exposure did not affect the viability and the clonogeneic potential of HSPCs (Figures 4A and 4B).
Homing is the first step into BM seeding by HSPCs after transplantation, which precedes their engraftment, proliferation, and differentiation. It is also well established that the CXCL12-CXCR4 axis plays a pivotal role in directing the homing of HSPCs from the PB to the BM [10,27,28]. While CXCL12 is expressed in the BM stroma, CXCR4 is present on the surface of HSPCs [29,30]. It is not surprising that proper functioning of the CXCL12-CXCR4 axis is crucial in directing the homing and engraftment of HSPCs into the BM after transplantation.
It has been recently demonstrated that CXCR4 requires an association with membrane lipid rafts for proper signaling function [31-34]. Additionally, it has been shown that membrane lipid rafts are important for T-cell polarization and chemotaxis [35]. Increased responsiveness of HSPCs to a CXCL12 gradient in the presence of other factors was named as the priming effect [36]. Previous work revealed that several small molecules (i.e., fibronectin and fibrinogen fragments, hyaluronic acid, granulocyte-derived cationic peptides, and C3a) that are present in supernatants from leukapheresis products may modulate the responsiveness of HSPCs to a CXCL12 gradient by enhancing the incorporation of CXCR4 into membrane lipid rafts [37-39].
In this study, we evaluated if the cationic peptide hBD-2 released from activated granulocytes will enhance homing and engraftment of transplanted HSPCs. We found that HSPCs stimulated by hBD-2 better responded to a CXCL12 gradient. Moreover, we found for the first time that transplantation of hBD-2-primed BMNCs into lethally irradiated recipients results in an increase in the number of donor-derived CFU-granulocyte-macrophage progenitors in the bone marrow cavities, as well as an increase in formation of CFU-S.
hBD-2 itself did not show chemotactic activity but an enhanced migration of HSPCs to a CXCL12 gradient compared to normal cells. Pretreatment of HSPCs with hBD-2 enhanced CXCL12-CXCR4-mediated intracellular signaling, adhesion to stromal cells, and their engraftment into irradiated mice, further emphasizing that hBD-2 plays an essential role in promoting the homing and engraftment of HSPCs to the bone marrow through its synergistic interaction with the CXCL12-CXCR4 signaling axis.
Considering the fact that CXCL12, secreted by bone marrow stromal cells, regulates the migration of HSPCs and thereby plays a central role in anchoring stem cells to the bone marrow microenvironment after transplantation, the sensitization of the CXCL12-CXCR4 axis by hBD-2 could be used to accelerate the homing and migration of transplanted HSPCs into the BM.
Activated granulocyte-derived AMPs might be safer than previously described priming agents (C3a, angiopoietin etc.) [20,21,40], because AMPs have been demonstrated to kill bacteria, enveloped viruses, fungi, and even transformed or cancerous cells without affecting the viability of eukaryotic cells. Selective effects of AMPs, like membrane perturbation of eukaryotic cells and killing of prokaryotic cells, are known to be dependent on the characteristics of membranes, such as cholesterol content or membrane electric charge. In agreement with our hypothesis, hBD-2 exposure did not affect the viability and differentiation potential of HSPCs.
Taken together, we suggest that hBD-2 might be employed as a new agent to prime ex vivo HSPCs before transplantation. This novel approach would be particularly important in umbilical cord blood transplantation, where the number of HSPCs available for reconstitution of recipient is often limited.
Acknowledgment
This study was supported by a grant from the Innovative Research Institute for Cell Therapy (A062260) by the Ministry of Health and Welfare, Republic of Korea.
References
1. Shi Q, Rafii S, Wu MH, Wijelath ES, Yu C, Ishida A, Fujita Y, Kothari S, Mohle R, Sauvage LR, Moore MA, Storb RF, Hammond WP. Evidence for circulating bone marrow-derived endothelial cells. Blood. 1998; 92(2):362–367. PMID: 9657732.


2. Rafii S, Lyden D. Therapeutic stem and progenitor cell transplantation for organ vascularization and regeneration. Nat Med. 2003; 9(6):702–712. PMID: 12778169.


3. Wang X, Ge S, McNamara G, Hao QL, Crooks GM, Nolta JA. Albumin-expressing hepatocyte-like cells develop in the livers of immune-deficient mice that received transplants of highly purified human hematopoietic stem cells. Blood. 2003; 101(10):4201–4208. PMID: 12560238.


4. Cogle CR, Yachnis AT, Laywell ED, Zander DS, Wingard JR, Steindler DA, Scott EW. Bone marrow transdifferentiation in brain after transplantation: a retrospective study. Lancet. 2004; 363(9419):1432–1427. PMID: 15121406.


5. Kassirer M, Zeltser D, Gluzman B, Leibovitz E, Goldberg Y, Roth A, Keren G, Rotstein R, Shapira I, Arber N, Berliner AS. The appearance of L-selectin(low) polymorphonuclear leukocytes in the circulating pool of peripheral blood during myocardial infarction correlates with neutrophilia and with the size of the infarct. Clin Cardiol. 1999; 22(11):721–726. PMID: 10554687.
6. Kyne L, Hausdorff JM, Knight E, Dukas L, Azhar G, Wei JY. Neutrophilia and congestive heart failure after acute myocardial infarction. Am Heart J. 2000; 139(1 Pt 1):94–100. PMID: 10618568.


7. Matsunaga T, Sakamaki S, Kohgo Y, Ohi S, Hirayama Y, Niitsu Y. Recombinant human granulocyte colony-stimulating factor can mobilize sufficient amounts of peripheral blood stem cells in healthy volunteers for allogeneic transplantation. Bone Marrow Transplant. 1993; 11(2):103–108. PMID: 7679596.
8. Sato N, Sawada K, Takahashi TA, Mogi Y, Asano S, Koike T, Sekiguchi S. A time course study for optimal harvest of peripheral blood progenitor cells by granulocyte colony-stimulating factor in healthy volunteers. Exp Hematol. 1994; 22(10):973–978. PMID: 7522184.
9. Papayannopoulou T, Nakamoto B, Andrews RG, Lyman SD, Lee MY. In vivo effects of Flt3/Flk2 ligand on mobilization of hematopoietic progenitors in primates and potent synergistic enhancement with granulocyte colony-stimulating factor. Blood. 1997; 90(2):620–629. PMID: 9226162.
10. Lapidot T, Dar A, Kollet O. How do stem cells find their way home? Blood. 2005; 106(6):1901–1910. PMID: 15890683.


12. Devine SM, Lazarus HM, Emerson SG. Clinical application of hematopoietic progenitor cell expansion: current status and future prospects. Bone Marrow Transplant. 2003; 31(4):241–252. PMID: 12621458.


13. Papayannopoulou T. Current mechanistic scenarios in hematopoietic stem/progenitor cell mobilization. Blood. 2004; 103(5):1580–1585. PMID: 14604975.


14. Zou YR, Kottmann AH, Kuroda M, Taniuchi I, Littman DR. Function of the chemokine receptor CXCR4 in haematopoiesis and in cerebellar development. Nature. 1998; 393(6685):595–599. PMID: 9634238.


15. Moore MA, Hattori K, Heissig B, Shieh JH, Dias S, Crystal RG, Rafii S. Mobilization of endothelial and hematopoietic stem and progenitor cells by adenovector-mediated elevation of serum levels of SDF-1, VEGF, and angiopoietin-1. Ann N Y Acad Sci. 2001; 938:36–45. PMID: 11458524.


16. Lapidot T, Kollet O. The essential roles of the chemokine SDF-1 and its receptor CXCR4 in human stem cell homing and repopulation of transplanted immune-deficient NOD/SCID and NOD/SCID/B2m(null) mice. Leukemia. 2002; 16(10):1992–2003. PMID: 12357350.


17. Kushida T, Inaba M, Hisha H, Ichioka N, Esumi T, Ogawa R, Iida H, Ikehara S. Intra-bone marrow injection of allogeneic bone marrow cells: a powerful new strategy for treatment of intractable autoimmune diseases in MRL/lpr mice. Blood. 2001; 97(10):3292–3299. PMID: 11342461.


18. Wang J, Kimura T, Asada R, Harada S, Yokota S, Kawamoto Y, Fujimura Y, Tsuji T, Ikehara S, Sonoda Y. SCID-repopulating cell activity of human cord blood-derived CD34- cells assured by intra-bone marrow injection. Blood. 2003; 101(8):2924–2931. PMID: 12480697.


19. Kahn J, Byk T, Jansson-Sjostrand L, Petit I, Shivtiel S, Nagler A, Hardan I, Deutsch V, Gazit Z, Gazit D, Karlsson S, Lapidot T. Overexpression of CXCR4 on human CD34+ progenitors increases their proliferation, migration, and NOD/SCID repopulation. Blood. 2004; 103(8):2942–2949. PMID: 15070669.


20. Ratajczak MZ, Reca R, Wysoczynski M, Yan J, Ratajczak J. Modulation of the SDF-1-CXCR4 axis by the third complement component (C3)-Implications for trafficking of CXCR4+ stem cells. Exp Hematol. 2006; 34(8):986–995. PMID: 16863905.


21. Reca R, Mastellos D, Majka M, Marquez L, Ratajczak J, Franchini S, Glodek A, Honczarenko M, Spruce LA, Janowska-Wieczorek A, Lambris JD, Ratajczak MZ. Functional receptor for C3a anaphylatoxin is expressed by normal hematopoietic stem/progenitor cells, and C3a enhances their homing-related responses to SDF-1. Blood. 2003; 101(10):3784–3793. PMID: 12511407.


22. Ratajczak MZ, Reca R, Wysoczynski M, Kucia M, Baran JT, Allendorf DJ, Ratajczak J, Ross GD. Transplantation studies in C3-deficient animals reveal a novel role of the third complement component (C3) in engraftment of bone marrow cells. Leukemia. 2004; 18(9):1482–1490. PMID: 15284858.


23. Malmsten M, Schmidtchen A. Antimicrobial C3a--biology, biophysics, and evolution. Adv Exp Med Biol. 2007; 598:141–158. PMID: 17892210.
24. Ganz T. Defensins: antimicrobial peptides of innate immunity. Nat Rev Immunol. 2003; 3(9):710–720. PMID: 12949495.


25. Lee HM, Wysoczynski M, Liu R, Shin DM, Kucia M, Botto M, Ratajczak J, Ratajczak MZ. Mobilization studies in complement-deficient mice reveal that optimal AMD3100 mobilization of hematopoietic stem cells depends on complement cascade activation by AMD3100-stimulated granulocytes. Leukemia. 2010; 24(3):573–582. PMID: 20033053.


26. Ratajczak MZ, Lee H, Wysoczynski M, Wan W, Marlicz W, Laughlin MJ, Kucia M, Janowska-Wieczorek A, Ratajczak J. Novel insight into stem cell mobilization-plasma sphingosine-1-phosphate is a major chemoattractant that directs the egress of hematopoietic stem progenitor cells from the bone marrow and its level in peripheral blood increases during mobilization due to activation of complement cascade/membrane attack complex. Leukemia. 2010; 24(5):976–985. PMID: 20357827.


27. Quesenberry PJ, Becker PS. Stem cell homing: rolling, crawling, and nesting. Proc Natl Acad Sci USA. 1998; 95(26):15155–15157. PMID: 9860935.


28. Lapidot T, Kollet O. The essential roles of the chemokine SDF-1 and its receptor CXCR4 in human stem cell homing and repopulation of transplanted immune-deficient NOD/SCID and NOD/SCID/B2m(null) mice. Leukemia. 2002; 16(10):1992–2003. PMID: 12357350.


29. Aiuti A, Webb IJ, Bleul C, Springer T, Gutierrez-Ramos JC. The chemokine SDF-1 is a chemoattractant for human CD34+ hematopoietic progenitor cells and provides a new mechanism to explain the mobilization of CD34+ progenitors to peripheral blood. J Exp Med. 1997; 185(1):111–120. PMID: 8996247.


30. Möhle R, Bautz F, Rafii S, Moore MA, Brugger W, Kanz L. The chemokine receptor CXCR-4 is expressed on CD34+ hematopoietic progenitors and leukemic cells and mediates transendothelial migration induced by stromal cell-derived factor-1. Blood. 1998; 91(12):4523–4530. PMID: 9616148.
31. Yang FC, Atkinson SJ, Gu Y, Borneo JB, Roberts AW, Zheng Y, Pennington J, Williams DA. Rac and Cdc42 GTPases control hematopoietic stem cell shape, adhesion, migration, and mobilization. Proc Natl Acad Sci USA. 2001; 98(10):5614–5618. PMID: 11320224.


32. Nguyen DH, Taub D. CXCR4 function requires membrane cholesterol: implications for HIV infection. J Immunol. 2002; 168(8):4121–4126. PMID: 11937572.


33. Raulin J. Human immunodeficiency virus and host cell lipids. Interesting pathways in research for a new HIV therapy. Prog Lipid Res. 2002; 41(1):27–65. PMID: 11694268.


34. Gómez-Moutón C, Lacalle RA, Mira E, Jiménez-Baranda S, Barber DF, Carrera AC, Martínez-A C, Mañes S. Dynamic redistribution of raft domains as an organizing platform for signaling during cell chemotaxis. J Cell Biol. 2004; 164(5):759–768. PMID: 14981096.
35. Fernandis AZ, Cherla RP, Ganju RK. Differential regulation of CXCR4-mediated T-cell chemotaxis and mitogen-activated protein kinase activation by the membrane tyrosine phosphatase, CD45. J Biol Chem. 2003; 278(11):9536–9543. PMID: 12519755.


36. Reca R, Mastellos D, Majka M, Marquez L, Ratajczak J, Franchini S, Glodek A, Honczarenko M, Spruce LA, Janowska-Wieczorek A, Lambris JD, Ratajczak MZ. Functional receptor for C3a anaphylatoxin is expressed by normal hematopoietic stem/progenitor cells, and C3a enhances their homing-related responses to SDF-1. Blood. 2003; 101(10):3784–3793. PMID: 12511407.


37. Gu Y, Filippi MD, Cancelas JA, Siefring JE, Williams EP, Jasti AC, Harris CE, Lee AW, Prabhakar R, Atkinson SJ, Kwiatkowski DJ, Williams DA. Hematopoietic cell regulation by Rac1 and Rac2 guanosine triphosphatases. Science. 2003; 302(5644):445–449. PMID: 14564009.


38. Filippi MD, Harris CE, Meller J, Gu Y, Zheng Y, Williams DA. Localization of Rac2 via the C terminus and aspartic acid 150 specifies superoxide generation, actin polarity and chemotaxis in neutrophils. Nat Immunol. 2004; 5(7):744–751. PMID: 15170212.


39. Cancelas JA, Lee AW, Prabhakar R, Stringer KF, Zheng Y, Williams DA. Rac GTPases differentially integrate signals regulating hematopoietic stem cell localization. Nat Med. 2005; 11(8):886–891. PMID: 16025125.


40. Kim MS, Lee CS, Hur J, Cho HJ, Jun SI, Kim TY, Lee SW, Suh JW, Park KW, Lee HY, Kang HJ, Lee DS, Koh GY, Nakagami H, Morishita R, Park YB, Kim HS. Priming with angiopoietin-1 augments the vasculogenic potential of the peripheral blood stem cells mobilized with granulocyte colony-stimulating factor through a novel Tie2/Ets-1 pathway. Circulation. 2009; 120(22):2240–2250. PMID: 19917886.


Figure 1
Migration of BMNCs and CFU-GM progenitor cells to a CXCL12 gradient. CXCL12 directly chemoattracts CFU-GM progenitor cells as well as BMNCs. Migration of BMNCs (A) and CFU-GM progenitor cells (B). Values are the fold increase of migrated cell numbers compared to media alone control. CXCL12 (ng/mL). *P<0.05 as compared with media alone control. The data shown represent the combined results from three independent experiments carried out in duplicate per group (n=6).
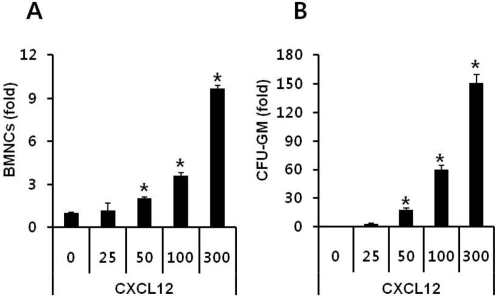
Figure 2
The cationic peptide hBD-2 enhances responsiveness of BM cells to a CXCL12 gradient. The migration of hBD-2-exposed BMNCs was significantly enhanced compared to that of normal BMNCs (A, BMNCs; B, CFU-GM progenitors) in response to a CXCL12 gradient. However, hBD-2 itself did not show direct chemotactic effect to BMNCs (C) and CFU-GM progenitor cells (D). Values are the fold increase of migrated cell numbers compared to control. CXCL12 (ng/mL), hBD-2 (µg/mL). *P<0.05 as compared to CXCL12 (50 ng/mL, A and B) or media alone control (C and D). The data shown represent the combined results from three independent experiments carried out in triplicate per group (n=9).
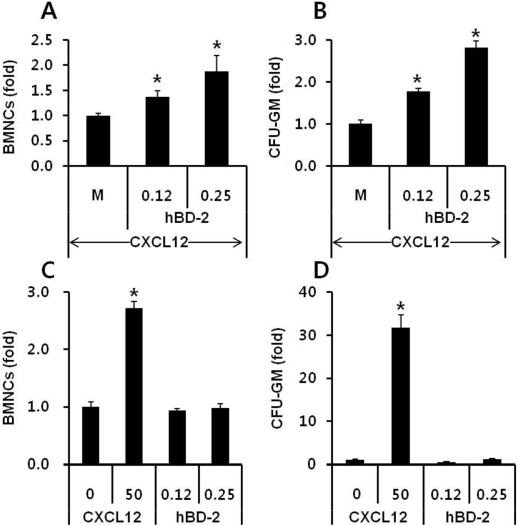
Figure 3
hBD-2 enhances p42/44 MAPK phosphorylation mediated by CXCL12. Downstream signaling mediated by the CXCL12-CXCR4 axis was strongly enhanced when CXCL12 was employed together with hBD-2 (A). hBD-2-primed CFU-GM progenitor cells showed enhance adhesion onto stromal cells (B). CXCL12 (L, 50 ng/mL; H, 300 ng/mL), hBD-2 (1 µg/mL). *P<0.05 as compared to non-primed CFU-GM progenitor cells. The data shown represent the combined results from three independent experiments carried out in duplicate per group (n=6).
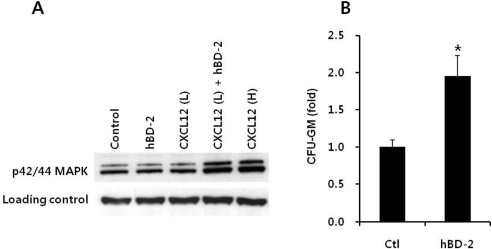
Figure 4
hBD-2 exposure on BMNCs does not affect the viability and differentiation potential of BM-derived HSPCs. BMNCs were exposed to different doses of hBD-2 for 60 minutes at 37℃ before the colony forming assay. CFU-GM (A) and CFU-M (B) differentiation of BMNCs. No significant differences were observed between normal or hBD-2 primed BMNCs. The data shown represent the combined results from two independent experiments carried out in duplicate per group (n=4).
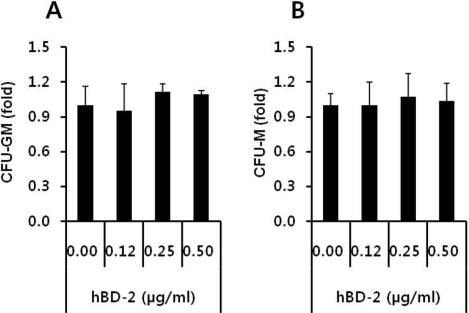
Figure 5
New strategy for the optimization of HSPC transplantation efficiency by employing cationic peptides. The homing and engraftment of BM-derived HSPCs (BM-HSPCs) and umbilical cord blood-derived HSPCs (UCB-HSPCs) might be accelerated by employing cationic peptides (i.e., hBD-2) as priming reagents. Mobilized or cationic peptide-primed HSPCs might home and engraft faster than BM-HSPCs or UCB-HSPCs. This novel approach would be particularly important in umbilical cord blood transplantation, where the number of HSPCs available for reconstitution of recipient is often limited.
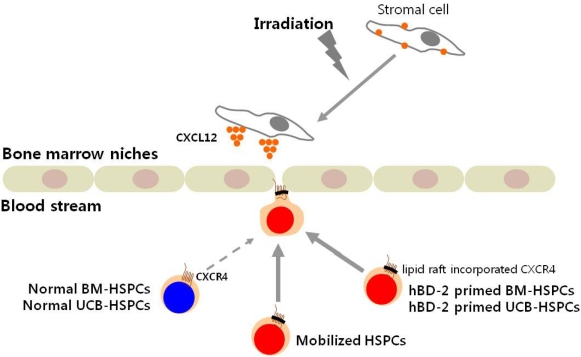