Abstract
Purpose
It remains unknown whether local inhibition of Nuclear factor-kappa B (NF-κB) could have therapeutic value in the treatment of allergic rhinitis (AR). This study aimed to evaluate the effect of selective NF-κB inhibition using NF-κB decoy oligodeoxynucleotides (ODNs) for the local treatment of AR in ovalbumin (OVA)-sensitized wild-type mice.
Methods
BALB/c mice were sensitized with OVA and alum, and then challenged intranasally with OVA. NF-κB decoy ODNs were given intranasally to the treatment group, and NF-κB scrambled ODNs were given to the sham treatment group. Allergic symptom scores, eosinophil infiltration, cytokine levels in the nasal mucosa, nasal lavage fluid, and spleen cell culture, serum total and OVA-specific immunoglobulins, as well as intercellular adhesion molecure-1 (ICAM-1) in the nasal mucosa, were analyzed.
Results
NF-κB decoy ODNs significantly reduced allergic symptoms and eosinophil infiltration in the nasal mucosa. They also suppressed serum levels of total IgE, OVA-specific IgE, and IgG1. IL-5 and TNF-α levels and the expression of ICAM-1 were decreased in the nasal mucosa of the treatment group compared to the positive control and sham treatment groups. In addition, IL-6 levels were significantly decreased in the nasal lavage fluid of the treatment group. Furthermore, NF-κB decoy ODNs significantly reduced expression of the systemic Th2 cytokines, IL-4 and IL-5 in spleen cell culture.
Nuclear factor-kappa B (NF-κB) is a multicellular transcription factor, and it plays an important role in inflammatory and immune responses by regulating immune and inflammation-related cytokine and inflammatory mediator genes expressions.1 In addition, the NF-κB pathway is involved in the proliferation and pathogenesis of tumors via the regulation of cell proliferation, control of apoptosis, promotion of angiogenesis, and stimulation of invasion/metastasis.2 Because of its pivotal role in inflammation and cell proliferation, several studies have reported that inhibition of NF-κB shows potential for the treatment of several human diseases.
Transfection of decoy oligodeoxynucleotides (ODNs) has been reported to inhibit NF-κB activation and to show potential as a therapeutic strategy.3 NF-κB decoy ODNs bind the transcription factor and interfere with the binding of NF-κB to the promotor regions of genes, thereby reducing the expression of its target genes.4 Topical application of NF-κB decoy ODNs induces antigen-specific peripheral tolerance in delayed-type hypersensitivity to ovalbumin (OVA).5
A study of a murine asthma model revealed that specific local NF-κB inhibition, achieved using NF-κB decoy ODNs, is associated with strong attenuation of allergic lung inflammation, airway hyper-responsiveness, local production of mucus, and cytokine expression, and therefore shows therapeutic potential in the control of pulmonary allergy.6 However, information regarding the association of local NF-κB inhibition with allergic inflammation in AR is limited, although it has been demonstrated in humans and animal models that NF-κB is up-regulated in the nasal mucosa of patients with allergic rhinitis (AR).789
The objective of the present study was to evaluate the effect of selective NF-κB inhibition, using NF-κB decoy ODNs, for local treatment of AR in OVA-sensitized wild-type mice.
Four-week-old female BALB/c mice were used as the experimental animals. Each mouse weighed 18-22 g and was maintained under specific pathogen-free conditions. All animal experiments in the present study followed the guidelines and ethics of the Institutional Animal Care and Use Committee of the Biomedical Research Institute of our hospital, and were approved (IACUC number: 12-0098&13-0088).
Double-stranded NF-κB decoy and scrambled ODNs were synthesized by Cosmo GENETECH (Seoul, Korea). Double-stranded NF-κB decoy ODNs containing the consensual NF-κB binding site (GGGATTTCCC) were generated using equimolar amounts of single-stranded sense and antisense phosphorothioate-modified ODNs (sense strand: 5'-CCT TGA AGG GAT TTC CCT CC-3'). Double-stranded NF-κB scrambled ODNs were used as controls (sense strand: 5'-TTG CCG TAC CTG ACT TAG CC-3').
Mice were divided into 4 groups, each consisting of 6 mice (Group A: negative control group, Group B: positive control group, Group C: NF-κB decoy ODN treatment group, and Group D: NF-κB scrambled ODN sham treatment group). In-dependent experiments for each group were repeated twice. Allergen sensitization, challenge for development of the AR murine model, and treatment are summarized in Fig. 1. Briefly, the mice were sensitized by intraperitoneal injection with 25 µg of OVA (grade V; Sigma, St. Louis, MO, USA) complexed with 2 mg of aluminum hydroxide (alum) on days 0, 7, and 14. The mice were then subjected to an intranasal challenge with 100 µg of OVA on 7 consecutive days from days 20 to day 26. The negative control mice were intraperitoneally injected and intranasally challenged with phosphate-buffered saline (PBS) instead of OVA on the same schedule. NF-κB decoy and scrambled ONDs were given by intranasal instillation (15 nmol in 30 µL of TE buffer/mouse) on days 20, 22, 24, and 26 (6 hours before intranasal OVA challenge) to mice in groups C and D, respectively.
On day 27, after intranasal allergen provocation with 100 µg of OVA, the frequencies of sneezing and nasal rubbing behaviors were recorded during a 15-minute period to evaluate early allergic responses by blinded observers. Mice were then killed 24 hours after the last OVA challenge, and nasal tissues/cells and nasal lavage fluid were obtained for analysis.
For the evaluation of nasal histology, the heads of 6 mice in each group were fixed with 10% formaldehyde solution. The nasal tissues were decalcified with ethylenediaminetetracetic acid (EDTA) solution, embedded in paraffin, sectioned coronally into 4-µm slices, and stained with Sirius red for the visualization of eosinophils. Under a light microscope (×400 magnification), infiltrating eosinophils were counted in 4 fields of the nasal septal mucosa by a single-blinded observer. Eosinophils were morphologically defined by the presence of eosinophilic granules that were stained by Sirius red in the cytoplasm and the presence of a two-lobed nucleus.1011
After removing the nasal cavity, the nasal mucosa of 6 mice in each group was carefully taken out using a curette. Total RNA was isolated from the nasal mucosa using the TriZol reagent (Invitrogen, Carlsbad, CA, USA). Complementary DNA (cDNA) was synthesized using SuperScript II reverse transcriptase (Invitrogen, Carlsbad, CA, USA) and oligo (dT) primers (Fermentas, Burlington, ON, Canada). For the analysis of interleukin (IL)-4 (Mm 00445258_g1), IL-5 (Mm 01290072_g1), IL-6 (Mm00446190_m1), tumor necrosis factor-α (TNF-α) (Mm 00443259_g1), interferon-gamma (IFN-γ) (Mm 99999071_m1), and glyceraldehyde-3-phosphate dehydrogenase (GAPDH) (Mm 03302249_g1), pre-developed assay reagent (PDAR) kits of primers and probes were purchased from Applied Biosystems (Foster City, CA, USA). Amplification of IL-4, IL-5, IL-6, TNF-α, IFN-γ, and GAPDH cDNA was performed in MicroAmp optical 96-well reaction plates (Applied Biosystems). The reaction was performed using an ABI PRISM 7000 Sequence Detection System (Applied Biosystems). The average transcript levels of genes were then normalized to GAPDH.
A 24-gauge catheter was inserted into the nasopharynx through the tracheal opening at the time of killing. The nasal passages were gently perfused twice with 200 µL of PBS from the choana to the nostril, and the nasal lavage fluid was collected. The lavage fluid was centrifuged and supernatants were frozen at -70℃ until analysis. IL-6 levels in the nasal lavage fluid were measured using a Quantikine enzyme-linked immunosorbent assay (ELISA) kit (R&D Systems, Minneapolis, MN, USA), which had a sensitivity of 1.6 pg/mL.
Spleen single-cell suspensions were plated in 24-well cell culture plates at a final concentration of 5×106 cells/well using RPMI 1640 containing 10% fetal bovine serum (FBS) supplemented with 100 units/mL penicillin and 100 µg/mL streptomycin (Gibco, Grand Island, NY, USA). The cells were incubated in a CO2 incubator at 37℃ for 72 hours and stimulated with OVA for 72 hours. The culture supernatant was collected and stored at -70℃ until cytokines were measured. Cytokine levels in the culture supernatant were assayed using a DuoSet ELISA kit (R&D Systems), according to the manufacturer's protocol. After measuring the optical density (OD) at 450 nm, the concentrations of IL-4, IL-5, IL-10, and IFN-γ were determined by interpolation from a standard curve, and all data are expressed in pg/mL.
Serum samples from mice were obtained at the time of killing. Serum levels of total IgE and OVA-specific IgE, IgG1, and IgG2a were measured by ELISA. For the analysis of total IgE, 96-well flat-bottom plates were coated overnight with anti-mouse IgE monoclonal antibody (mAb) (BD PharMingen, San Jose, CA, USA) at 4℃. The plate was washed with PBST (PBS containing 0.05% Tween-20) 3 times and nonspecific antigen-anti-body reactions were blocked with 300 µL of 3% bovine serum albumin (BSA) per well for 1 hour at room temperature. Serum samples were added to the 96-well plates along with purified mouse IgE isotype (BD PharMingen) used as a standard, and the plates were incubated for 3 hours at 4℃. For the analysis of OVA-specific IgE, 96-well flat-bottom plates were coated with OVA 100 µg/mL in coating buffer (0.05M carbonate-bicarbonate) overnight at 4℃. The plates were washed 3 times with PBST, and blocked with 3% BSA in PBS for 1 hour at 37℃. Serum samples were added to OVA-coated plates along with serial dilutions of a high-titer OVA IgE standard and incubated for 2 hours at 37℃. After washing, 100 µL of biotin-conjugated rat anti-mouse IgE mAb (BD PharMingen, San Jose, CA, USA) was added to each well and incubated for 1 hour at 37℃. For the analysis of OVA-specific IgG1 and IgG2a, 96-well plates were coated with 100 µg/mL OVA in coating buffer overnight at 4℃. Serially diluted serum samples were incubated with biotinylated rat anti-mouse IgG1 and IgG2a (BD PharMingen), respectively. After washing three times, the plates were then incubated with 100 µL of horseradish peroxidase (HRP)-conjugated secondary Ab (BD PharMingen) for 30 minutes at 37℃. The reactions were developed using 3, 3', 5, 5'-tetramethylbenzidine (TMB) (Moss Inc., Belfast, ME, USA) and terminated by adding 1N HCL. OD was measured in a microplate reader at 450 nm.
Protein was obtained from the nasal mucosa of 6 mice in each group 24 hours after the final nasal challenge using a commercial lysis buffer (TransAM kit, Active Motif, Carlsbad, CA, USA). Protein concentrations were determined using BCA protein assay reagent (Thermo Fisher Scientific, Waltham, MA, USA). Samples (40 µg protein per lane) were separated on Nupage 4%-12% Bis-Tris mini gels (NOVEX, San Diego, CA, USA) and transferred onto polyvinylidene fluoride membranes (Amersham Biosciences, Piscataway, NJ, USA). ICAM-1 and β-actin were immunoblotted with a primary goat polyclonal anti-ICAM-1 Ab (R&D Systems) and anti-β-actin Ab (Cell signaling, Danvers, MA, USA). The membrane was then immunoblotted with a secondary anti-goat IgG-HRP (Santa Cruz Biotechnology, Santa Cruz, CA, USA). The blots were visualized using SuperSignal West Pico Chemiluminescent Substrate (Pierce, Rockford, IL, USA). Quantification of Western blots was performed using the TINA 2.0 software from the National Institutes of Health.
The data are presented as means±standard error mean (SEM). A Mann-Whitney U test was used to compare results between negative and positive controls, and treatment groups and positive control. A P value of <0.05 was considered statistically significant. Statistical analysis was performed using SPSS 18.0 software (SPSS Inc., Chicago, IL, USA).
Fig. 2 shows symptom scores for each group after nasal challenge with OVA. Mice in group B (positive control) sneezed (P=0.002) and rubbed (P<0.001) their noses significantly more frequently than those in group A (negative control). Sneezing (P=0.009) and nasal rubbing scores (P=0.002) were significantly lower in group C (NF-κB decoy ODN treatment group) than in group B. Group D (NF-κB scrambled ODN sham treatment group) did not differ significantly from group B in sneezing (P=0.449) or nasal rubbing scores (P=0.383).
Fig. 3 shows eosinophil infiltration in the nasal mucosa for each group. The mice in group B had significantly more severe eosinophil infiltration than the those in group A (P=0.004). The number of eosinophils infiltrating the nasal mucosa per high-magnification field were significantly lower in group C (P=0.004), but not in group D (P=0.078), than in group B. These results show that local NF-κB inhibition using NF-κB decoy ODNs decreased eosinophil migration into the nasal mucosa. In contrast, treatment with scrambled ODNs had no significant effect on eosinophil influx.
The mRNA levels of the inflammatory cytokines, IL-5 (P=0.028) and TNF-α (P=0.034), were significantly lower in the nasal mucosa of the treatment group than in that of the positive control group (Fig. 4). Although transcription of IL-4 (P=0.289) and IL-6 (P=0.248) were lower in the nasal mucosa of the treatment group than in those of the positive control group, the differences were not statistically significant. IFN-γ mRNA levels in the nasal mucosa showed no significant differences between the groups. The concentration of IL-6 was significantly decreased in the nasal lavage fluid of the treatment group (P=0.027, Fig. 5), as compared to the positive control group. We only showed IL-6 in nasal lavage fluid. Although other cytokines, such as IL-4, IL-5, TNF-α, and IFN-γ were evaluated in nasal lavage fluid, they were not detected except for IL-6. In addition, levels of systemic Th2 cytokines (IL-4, P=0.039; IL-5, P=0.027) from spleen cell culture were significantly decreased in group C, as compared to group B (Fig. 6A and B). However, levels of systemic regulatory cytokine IL-10 (P=0.288) and the Th1 cytokine IFN-γ (P=0.895) did not change in the treatment group (Fig. 6C and D) as in controls.
The serum levels of total IgE (P=0.043; P=0.009, Fig. 7A), OVA-specific IgE (P=0.039; P=0.013, Fig. 7B), and OVA-specific IgG1, Th2-related Ig, (P=0.027; P=0.021, Fig. 7C) in group C were significantly lower than in groups B and D. Level of OVA-specific IgG2a, Th1-related Ig, was higher in group C than in groups B (P=0.051) and D (P=0.023) (Fig. 7D).
The expression levels of ICAM-1 protein in the nasal mucosa were significantly decreased in the NF-κB decoy ODNs treatment group, but not in the sham treatment group, as compared to the positive control group (Fig. 8).
The transcription factor NF-κB plays an important role in a wide range of inflammatory diseases.12 NF-κB not only induces the transcription of several cytokines (e.g., TNF-α, IL-1β, and IL-6) and chemokines (e.g., IL-8, macrophage inflammatory protein-1a, methyl-accepting chemotaxis protein 1 and eotaxin), but also regulates the expression of adhesion molecules (e.g. E-selectin, vascular cell adhesion molecule-1, and ICAM-1).13 In addition, several studies have indicated that NF-κB expression and activity are involved in the proliferation and pathogenesis of tumors.14 Because of its important role in inflammation and cell proliferation, much effort has been made in the development of therapeutics that inhibits NF-κB and regulates the expression of its target genes.
Decoy ODNs have been explored as tools for manipulating transcription factors. When a decoy ODNs containing the consensus sequence of a specific transcription factor is introduced into cells at high levels, the decoy ODNs will compete with the endogenous gene targets for transcription factor binding, which will alter transcription of target genes.15 Therapeutic use of the decoy ODNs was strongly hampered by their low bioavailability and short half-life. However, chemical ODNs modifications are being proposed to overcome these limits. The most frequently used modification is the introduction of phosphorothioate groups, in which the non-bridging oxygen of the phosphodiester backbone is replaced by sulfur. Phosphoro-thioate ODNs are characterized by an increased stability.16 Therefore, we used the phosphorothioate-modified ODNs.
Several studies have shown that NF-κB decoy ODNs suppress the transcriptional activity of NF-κB by specifically blocking NF-κB binding sites, resulting in the inhibition of endogenous gene promoter. Fang et al.17 reported that NF-κB decoy ODNs suppressed proliferation and induced apoptosis of androgen-independent prostate cancer by local NF-κB inhibition. Isomura et al.18 reported that NF-κB decoy ODNs suppressed OVA-induced delayed-type hypersensitivity by suppressing the migration and maturation of dendritic cells, which are associated with activated NF-κB.
AR and asthma show similar pathophysiology and immunopathology, and also share common treatments, suggesting that inflammatory responses in the upper and lower respiratory tracts are consistent.19 Various therapeutic strategies targeting the NF-κB pathway have been documented in experimental asthma models. Desmet et al.6 reported that intratracheal administration of NF-κB decoy ODNs leads to NF-κB inhibition, which has therapeutic potential in the treatment of asthma. Bao et al.20 demonstrated the potential therapeutic value of andrographolide in the treatment of asthma by NF-κB inhibition. However, therapeutic challenge by NF-κB inhibition has not yet been established in AR. To the best of our knowledge, the current study is the first to show inhibition of the allergic response by local NF-κB inhibitor, NF-κB decoy ODNs, in the murine AR model.
NF-κB is commonly found in the cytoplasm of various cells in its non-active state. NF-κB is activated by multiple stimuli, including allergens and transferred into the nucleus, where it combines with specific DNA sequences of related gene promoters or enhancers to regulate and control expression of a variety of genes.14 The main activated form of NF-κB is a heterodimer, consisting of a p50 or p52 subunit and the trans-activating subunit p65. It has been reported that when NF-κB is activated, resulting in the nuclear localization of NF-κB p65, that secretion of inflammatory cytokines, such as IL-1β, IL-6, and TNF-α, is increased.821 Furthermore, several of these gene products, such as IL-1β and TNF-α, also directly activate the NF-κB pathway to amplify and increase the inflammatory response.13 In the present study, mRNA expression of IL-5 and TNF-α in the nasal mucosa and IL-6 protein level in the nasal lavage fluid were lower in the NF-κB decoy ODNs treatment group than in the positive control group, suggesting that decreased inflammatory cytokine expression via NF-κB inhibition is a key mechanism in its anti-allergic effect.
NF-κB decoy ODNs significantly decreased the allergic symptom scores and levels of the Th2 cytokines IL-4 and IL-5 in spleen cell culture in the treatment group. In addition, serum total and OVA-specific IgEs and IgG1 also decreased. IL-4 is well known to be an important cytokine not only for the Th2 phenotype but also for IgE production.22 These findings are consistent with our results that NF-κB decoy ODNs inhibited IL-4 secretion, resulting in decreased serum total and OVA-specific IgEs. OVA-specific IgG1 has been used as a Th2 marker and is correlated with total and OVA-specific IgE levels.23 IFN-γ, a Th1 cytokine, enhances IgG2a secretion.24 Levels of OVA-specific IgG1 significantly decreased and levels of OVA-specific IgG2a increased, but not significantly, in the treatment group, demonstrating that local NF-κB inhibition decreased Th2 immune response and increased Th1 immune response. Allergic disease is dominated by Th2 immune response and exhibiting unbalanced Th1/Th2 responses. This diminished Th2 predominance indicates the anti-allergic effect of local NF-κB inhibition. The limitation of the present study is that we only focused on the Th1 and Th2 cytokines, although Th17 or innate cytokines,25 such as IL-25, IL-33, and TSLP are a very important topic in the field of immunology. However, allergic diseases are still typically evaluated in terms of the Th1/Th2 paradigm, and Th2 predominance is a characteristic of allergic diseases.
The present study showed that the expression level of ICAM-1 protein and eosinophil infiltration in nasal mucosa decreased in the NF-κB decoy ODNs treatment group compared to the positive control group. Although a previous study reported that the expression levels of ICAM-1 did not increase in the positive control group or decrease in the TNF-α treatment group,26 ICAM-1 has been reported to play an important role in local inflammation and eosinophil infiltration, and gene expression is regulated by NF-κB.27 Wang et al.7 showed that the expression of NF-κB p50 and p65 has a significant positive correlation with that of ICAM-1 mRNA, and NF-κB plays an important role in ICAM-1 transcription in the nasal mucosa of AR patients. The decrease in the expression of ICAM-1 protein suggests that local inhibition of NF-κB leads to a decrease in the transcription of ICAM-1 and prevents adhesion of eosinophils on endothelial cells, thus inhibiting eosinophil infiltration in the nasal mucosa. For the limitation of the present study, ICAM-1 expression was increased by less than 1.2-fold in the positive control group and the reduction was very weak in the NF-κB treatment group, although there was statistical significance. Further studies would be needed to describe significance of ICAM-1 expression more clearly.
In this study, local treatment of NF-κB decoy ODNs suppressed both local and systemic allergic responses. In asthma model by Desmet et al.,6 local NF-κB inhibition did not change the systemic response, such as serum OVA-specific IgE or IgG1. They explained that NF-κB decoys did not affect the secondary immune response that takes place in thoracic lymph nodes upon OVA challenge. On the contrary, another study by Isomura et al.18 reported that topical application of NF-κB decoy ODN delayed dendritic cells' migration from peripheral tissue to draining lymph node in OVA sensitized mice. This result suggests that local NF-κB inhibition can affect secondary immune response that takes place in lymph nodes, and thus demonstrate systemic effects.
We selected intranasal instillation, rather than intraperitoneal injection, as the treatment method to prevent systemic adverse effects. Morishita et al.28 showed that systemic inhibition of NF-κB is likely to be harmful, given that knockout mice for various NF-κB signaling components suffer from immune deficiency or lack lymphocyte activation. In addition, Desmet et al.6 presented that local delivery of NF-κB decoy ODNs only partially inhibits NF-κB activity as assessed by a supershift assay. However, for the development of new therapeutic agents, additional studies are required to clarify the systemic side effects.
We did not find exact mechanism underlying NF-κB inhibition for anti-allergic effect. Although we suggest that decreased inflammatory cytokine expression via NF-κB inhibition is a key mechanism in its anti-allergic effect, we need further studies, including regulatory T cell studies for evaluating possible mechanism.
Even though the present study has some limitations, we have demonstrated, for the first time, which local NF-κB inhibition using NF-κB decoy ODNs suppressed allergic responses, such as Th2 cytokine production, total and OVA-specific IgE levels, adhesion molecule (ICAM-1) expression, and eosinophil infiltration into the nasal mucosa in a murine AR model. Therefore, local NF-κB inhibition should be considered a potential therapeutic target in the treatment of AR.
Figures and Tables
Fig. 1
Experimental protocol. BALB/c mice were sensitized with intraperitoneal injection of ovalbumine (OVA) and alum on days 0, 7, and 14. Intranasal challenges were administered via daily OVA instillation from days 20 to 26. The control mice (group A) were intraperitoneally injected and intranasally chal-lenged with phosphate-buffered saline (PBS) instead of OVA on the same schedule. In addition to sensitization and challenge, mice of group C were treated with nasal instillation of the nuclear factor-kappa B (NF-κB) decoy oligodeoxynucleotides (ODNs) and those of group D were sham treated with NF-κB scrambled ODNs on days 20, 22, 24, and 26 (6 hours before nasal challenge). On day 27, after intranasal allergen provocation with 100 µg of OVA, the frequencies of sneezing and nasal rubbing behaviors were recorded during 15 minutes. Mice were killed 24 hours after the final OVA challenge.
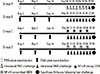
Fig. 2
Symptom scores. Local treatment of NF-κB inhibitor (Group C) suppressed allergic symptoms. Sneezing symptom score (A) and nasal rubbing symptom score (B) of each group. Data are expressed as mean±standard error mean (SEM). *P<0.05.
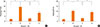
Fig. 3
Local treatment of NF-κB inhibitor (Group C) suppressed eosinophil infiltration in the nasal mucosa. Sirius red staining (×400 magnification) of each group (A). Photographs of representative nasal mucosa in each group. Eosinophil count of the septal mucosa of each group (B, n=6). Data are expressed as mean±standard error mean (SEM). *P<0.05.
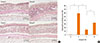
Fig. 4
Expression of IL-4 (A), IL-5 (B), IL-6 (C), TNF-α (D), and IFN-γ (E) in the nasal mucosa by real time PCR. The transcriptional levels of IL-5 and TNF-α were decreased in treatment group C than positive control group B. Data are expressed as mean±standard error mean (SEM). *P<0.05.
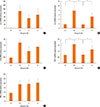
Fig. 5
Concentrations of IL-6 in nasal lavage fluid of each group by ELISA. The levels of IL-6 were significantly decreased in treatment group C than positive control group B. Data are expressed as mean±standard error mean (SEM). *P<0.05.
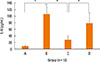
Fig. 6
Concentrations of IL-4 (A), IL-5 (B), IL-10 (C), and IFN-γ (D) in spleen cell culture of each group by ELISA. Systemic Th2 cytokines (IL-4 and IL-5) were significantly decreased in treatment group C than in positive control group B. However, systemic regulatory cytokine (IL-10) or Th1 cytokine (IFN-γ) did not change in treatment group C. Data are expressed as mean±standard error mean (SEM). *P<0.05.
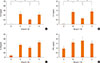
Fig. 7
Serum immunoglobulin levels in each group. Local treatment with NF-κB decoy ODNs (Group C) significantly suppressed levels of serum total IgE (A), OVA-specific IgE (B), and IgG1 (C). The serum levels of OVA-specific IgG2a (D) were increased. Data are expressed as mean±standard error mean (SEM). *P<0.05.
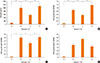
Fig. 8
Local treatment with NF-κB decoy ODNs (Group C) significantly reduced the expression of ICAM-1 protein in the nasal mucosa by Western blot. Immunoblot of ICAM-1 and β-actin in each group (A). Photographs of representative sample in each group. Quantitative expression level of ICAM-1/β-actin in the nasal mucosa of each group (B, n=6). Data are expressed as mean±standard error mean (SEM). *P<0.05.
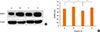
ACKNOWLEDGMENTS
This work was supported by grant No. 03-2012-0260 from the Seoul National University Hospital research fund.
References
1. Ghosh S, May MJ, Kopp EB. NF-kappa B and Rel proteins: evolutionarily conserved mediators of immune responses. Annu Rev Immunol. 1998; 16:225–260.
2. Kim HJ, Hawke N, Baldwin AS. NF-kappaB and IKK as therapeutic targets in cancer. Cell Death Differ. 2006; 13:738–747.
3. Yamasaki K, Asai T, Shimizu M, Aoki M, Hashiya N, Sakonjo H, et al. Inhibition of NFkappaB activation using cis-element 'decoy' of NFkappaB binding site reduces neointimal formation in porcine balloon-injured coronary artery model. Gene Ther. 2003; 10:356–364.
4. Crinelli R, Bianchi M, Gentilini L, Magnani M. Design and characterization of decoy oligonucleotides containing locked nucleic acids. Nucleic Acids Res. 2002; 30:2435–2443.
5. Isomura I, Shintani Y, Yasuda Y, Tsujimura K, Morita A. Induction of regulatory dendritic cells by topical application of NF-kappaB decoy oligodeoxynucleotides. Immunol Lett. 2008; 119:49–56.
6. Desmet C, Gosset P, Pajak B, Cataldo D, Bentires-Alj M, Lekeux P, et al. Selective blockade of NF-kappa B activity in airway immune cells inhibits the effector phase of experimental asthma. J Immunol. 2004; 173:5766–5775.
7. Wang SZ, Ma FM, Zhao JD. Expressions of nuclear factor-kappa B p50 and p65 and their significance in the up-regulation of intercellular cell adhesion molecule-1 mRNA in the nasal mucosa of allergic rhinitis patients. Eur Arch Otorhinolaryngol. 2013; 270:1329–1334.
8. Wang W, Zheng M. Nuclear factor kappa B pathway down-regulates aquaporin 5 in the nasal mucosa of rats with allergic rhinitis. Eur Arch Otorhinolaryngol. 2011; 268:73–81.
9. Kim ST, Oh SC, Kim CW, Park C, Jang IH, Cha HE, et al. Expression of NF-κB and I-kB in allergic rhinitis. Korean J Otolaryngol-Head Neck Surg. 2000; 43:1191–1195.
10. Percy DH, Barthold SW. Pathology of laboratory rodents and rabbits. 3rd ed. Ames (IA): Blackwell Publishing;2007.
11. Meyerholz DK, Griffin MA, Castilow EM, Varga SM. Comparison of histochemical methods for murine eosinophil detection in an RSV vaccine-enhanced inflammation model. Toxicol Pathol. 2009; 37:249–255.
12. Tak PP, Firestein GS. NF-kappaB: a key role in inflammatory diseases. J Clin Invest. 2001; 107:7–11.
13. Yamamoto Y, Gaynor RB. IkappaB kinases: key regulators of the NF-kappaB pathway. Trends Biochem Sci. 2004; 29:72–79.
14. Baldwin AS Jr. The NF-kappa B and I kappa B proteins: new discoveries and insights. Annu Rev Immunol. 1996; 14:649–683.
15. Wang T, Li QH, Hao GP, Zhai J. Antitumor activity of decoy oligodeoxynucleotides targeted to NF-kappaB in vitro and in vivo. Asian Pac J Cancer Prev. 2010; 11:193–200.
16. De Stefano D. Oligonucleotides decoy to NF-kappaB: becoming a reality? Discov Med. 2011; 12:97–105.
17. Fang Y, Sun H, Zhai J, Zhang Y, Yi S, Hao G, et al. Antitumor activity of NF-kB decoy oligodeoxynucleotides in a prostate cancer cell line. Asian Pac J Cancer Prev. 2011; 12:2721–2726.
18. Isomura I, Tsujimura K, Morita A. Antigen-specific peripheral tolerance induced by topical application of NF-kappaB decoy oligodeoxynucleotide. J Invest Dermatol. 2006; 126:97–104.
19. Meltzer EO. The relationships of rhinitis and asthma. Allergy Asthma Proc. 2005; 26:336–340.
20. Bao Z, Guan S, Cheng C, Wu S, Wong SH, Kemeny DM, et al. A novel antiinflammatory role for andrographolide in asthma via inhibition of the nuclear factor-kappaB pathway. Am J Respir Crit Care Med. 2009; 179:657–665.
21. Wang W, Zheng M. Mucin 5 subtype AC expression and upregulation in the nasal mucosa of allergic rhinitis rats. Otolaryngol Head Neck Surg. 2012; 147:1012–1019.
22. Ueda A, Chandswang N, Ovary Z. The action of interleukin-4 on antigen-specific IgG1 and IgE production by interaction of in vivo primed B cells and carrier-specific cloned Th2 cells. Cell Immunol. 1990; 128:31–40.
23. Lewkowich IP, Rempel JD, HayGlass KT. Antigen-specific versus total immunoglobulin synthesis: total IgE and IgG1, but not IgG2a levels predict murine antigen-specific responses. Int Arch Allergy Immunol. 2004; 133:145–153.
24. Bossie A, Vitetta ES. IFN-gamma enhances secretion of IgG2a from IgG2a-committed LPS-stimulated murine B cells: implications for the role of IFN-gamma in class switching. Cell Immunol. 1991; 135:95–104.
25. Fan D, Wang X, Wang M, Wang Y, Zhang L, Li Y, et al. Allergen-dependent differences in ILC2s frequencies in patients with allergic rhinitis. Allergy Asthma Immunol Res. 2016; 8:216–222.
26. Mo JH, Kang EK, Quan SH, Rhee CS, Lee CH, Kim DY. Anti-tumor necrosis factor-alpha treatment reduces allergic responses in an allergic rhinitis mouse model. Allergy. 2011; 66:279–286.
27. Yang CR, Hsieh SL, Ho FM, Lin WW. Decoy receptor 3 increases monocyte adhesion to endothelial cells via NF-kappa B-dependent up-regulation of intercellular adhesion molecule-1, VCAM-1, and IL-8 expression. J Immunol. 2005; 174:1647–1656.
28. Morishita R, Sugimoto T, Aoki M, Kida I, Tomita N, Moriguchi A, et al. In vivo transfection of cis element "decoy" against nuclear factor-kappaB binding site prevents myocardial infarction. Nat Med. 1997; 3:894–899.