Abstract
Interleukin (IL)-17 plays an important role in rhinitis and the level thereof correlates with the severity of disease. However, no mouse model for IL-17-dominant rhinitis has yet been developed. Our objective was to establish a mouse model of IL-17-dominant rhinitis via intranasal application of polyinosinic-polycytidylic acid (abbreviated as Poly(I:C)). Mice were divided into 6 groups (n=8 for each group); 1) 1 negative control group, 2) 1 positive control group (OVA/alum model), 3) 2 Poly(I:C) groups (10 or 100 µg), and 4) 2 OVA/Poly(I:C) groups (10 or 100 µg). The positive control group was treated with the conventional OVA/alum protocol. In the Poly(I:C) and OVA/Poly(I:C) groups, phosphate-buffered saline or an OVA solution plus Poly(I:C) were administered. The OVA/Poly(I:C) groups exhibited significantly greater neutrophil infiltration and increased IL-17/interferon γ expression compared with the other groups. However, the levels of total immunoglobulin E (IgE), OVA-specific IgE, eosinophil infiltration, IL-4, IL-5, IL-6, and IL-10 were significantly lower in the OVA/Poly(I:C) groups than in mice subjected to conventional Th2-dominant OVA/alum treatment (the positive control group). IL-17 and neutrophil measurement, chemokine (C-X-C motif) ligand 1 immunohistochemistry, and confocal microscopy revealed increased numbers of IL-17-secreting cells in the nasal mucosa of the OVA/Poly(I:C) groups, which included natural killer cells, CD4 T cells, and neutrophils. In conclusion, we developed a mouse model of IL-17-dominant rhinitis using OVA together with Poly(I:C). This model will be useful in research on neutrophil- or IL-17-dominant rhinitis.
Rhinitis is a common upper airway disease in which the pathogenesis reflects a complex interaction of genetic and environmental factors. In recent years, the classic paradigm of Th1/Th2 immunity has evolved to include a novel Th-cell subset expressing interleukin (IL)-17. These Th17 cells are involved in several immune-mediated diseases, including inflammatory bowel disease, asthma, and rhinitis.1
Increased IL-17 expression has been reported in many allergic diseases, including allergic rhinitis (AR). The IL-17A level and the Th17 cell number in the peripheral blood are increased in patients with AR and the serum IL-17 level correlates with symptom severity.23 In addition, IL-17A has been suggested to contribute to the development of allergic diseases by enhancing neutrophil recruitment to sites of airway inflammation.4
As human experimentation is ethically difficult, many animal models have been developed to provide insights into disease pathogenesis and drug development. A well-established murine model featuring nasal delivery of ovalbumin (OVA)/alum is commonly used to study Th2 inflammation of both the upper and lower airways. However, no model of Th17-mediated inflammatory rhinitis is yet available, although an established asthma model uses polyinosinic-polycytidylic acid (abbreviated as Poly(I:C)) to trigger lower airway disease.567
Here, we hypothesized that allergen sensitization with Poly(I:C) would induce an IL-17-dominant immune response in the upper airway. We developed an IL-17- and neutrophil-dominant model of mouse rhinitis and characterized its immunological features.
Forty-eight female BALB/c mice (4 weeks of age 18-20 g; Nara BioTech, Seoul, Korea) were divided into 6 groups (n=8 for each group); 1) 1 negative control group, 2) 1 positive control group (OVA/alum model), 3) 2 Poly(I:C) groups (10 or 100 µg), and 4) 2 OVA/Poly(I:C) groups (10 or 100 µg) (Fig. 1A). The positive control group was injected with OVA and alum mixtures intraperitoneally on day 1, 7, and 14 for sensitization. The negative control group was injected with phosphate-buffered saline (PBS) instead of OVA and alum. In the Poly(I:C) or OVA/Poly(I:C) groups, PBS or OVA/Poly(I:C) mixtures were administered via the intranasal route on days 1, 2, 3, 7, and 14 and PBS or OVA were administered intranasally on days 14 to 21 for challenge. Mice were sacrificed via cervical dislocation on day 21. The committee on the use and care of animal approved all animal experiments and we followed strict governmental and international guidelines on animal experimentation (DKU-2014-039).
After the final OVA challenge on day 21, a blinded observer recorded the frequencies of sneezing and nasal rubbing for 15 minutes. The mice were sacrificed 24 hours after the last OVA challenge and tissue samples were collected (nasal mucosa sampling n=4; histologic samples n=4 for each group). The detailed procedure was described in our previous report.8
The serum levels of total and OVA-specific IgE were measured by solid-phase enzyme-linked immunosorbent assay (ELISA). The detailed procedure was described in our previous report.8
The nasal septum histology was evaluated using standard Sirius red staining. The detailed procedure was described in our previous report.8
Cytokines were measured using ELISA. The detailed procedure was described in our previous report.8
Nasal mucosa sections were immunostained with natural killer (NK) cell marker (NKp46, 1:200; Biorbyt, Cambridge, UK), neutrophil marker (NIMP-R14, 1:100; Abcam, Cambridge, MA, USA), IL-17 (1:100; LSbio, Seattle, WA, USA), chemokine ligand 1 (CXCL1, GRO alpha, 1:1,000; Abcam), CD4 (1:200; Santa Cruz Biotechnology, Santa Cruz, CA, USA) using the avidin-biotinylated-horseradish peroxidase (HRP) complex kits (Vector Laboratories, Burlingame, CA, USA). The detailed procedure was described in our previous report.8
Dual immunofluorescent staining was performed to identify IL-17 expressing cells using IL-17, NKp46, NIMP-R14, CD4, and major basic protein (MBP) for eosinophil antibody. The following primary antibodies were used: IL-17 (1:100; LSbio and 1:100; Abcam), NKp46 (1:200; Biorbyt), neutrophil (1:100; Abcam), CD4 (1:100; Santa Cruz Biotechnology), MBP (1:100; Abcam), Alexa Fluor 488 (1:200; Life Technologies, Carlsbad, CA, USA), biotinylated anti rat (1:100; Vector Laboratories), and streptavidin-Cy3 (1:100; Sigma-Aldrich, St. Louis, MO, USA).
All statistical analyses were performed using GraphPad Prism 4 software (GraphPad Software, San Diego, CA, USA). Results are expressed as the mean±standard error of mean (SEM). A Mann-Whitney U test was used to compare results between groups. A P value of <0.05 was considered statistically significant.
The rubbing and sneezing symptom scores were significantly higher in the positive control group than in the negative control group. The Poly(I:C)-alone and OVA/Poly(I:C) (10 µg) groups had lower symptom scores than the positive control group. However, the OVA/Poly(I:C) (100 µg) group had symptom scores similar to those of the positive control group (P>0.05; Fig. 1B).
We assessed the effects of Poly(I:C) treatment on humoral immunity (Fig. 1C). The positive control group had higher serum total IgE, OVA-specific IgE, IgG1, and IgG2a levels than the negative control group. The total and OVA-specific IgE levels were significantly lower in the Poly(I:C) and OVA/Poly(I:C) groups than in the positive control group (P<0.001 for both comparisons). However, the OVA/Poly(I:C) (100 µg) group had an OVA-specific IgG1 level similar to that of the positive control group and an elevated OVA-specific IgG2a level (P=0.04 for both). Together, the data showed that neither Poly(I:C) nor OVA/Poly(I:C) had a notable effect on the levels of total or OVA-specific IgE.
The eosinophil count was significantly higher in the positive control group than in the negative control group. The eosinophil counts were significantly lower in the Poly(I:C) and OVA/Poly(I:C) groups than in the positive control group (Fig. 2A). However, the neutrophil counts in the OVA/Poly(I:C) groups increased significantly, in a dose-dependent manner (Fig. 2B). As IL-17A is known to induce neutrophil infiltration, we counted the numbers of IL-17A-producing cells. Such cells increased significantly in number in the OVA/Poly(I:C) (100 µg) group (Fig. 2C), showing that OVA/Poly(I:C) induced neutrophil infiltration and IL-17A production in the nasal mucosa.
The levels of mRNAs encoding IL-4 and IL-6 in the nasal mucosa were significantly lower in the OVA/Poly(I:C) groups compared with the positive control group (Fig. 3A). The levels of mRNA encoding IL-5 were similar in the OVA/Poly(I:C) (100 µg) and the positive control groups. The levels of mRNA encoding IL-10 did not differ significantly among the groups. Notably, the levels of mRNA encoding IL-17 was significantly increased in the OVA/Poly(I:C) (100 µg) group, consistent with the immunohistochemical findings (Figs. 2C and 3A). The levels of mRNA encoding interferon (IFN)-γ were somewhat increased in the OVA/Poly(I:C) groups compared with the other groups, but statistical significance was not attained (P>0.05 for all).
The systemic cytokine profiles derived from spleen culture were similar to those of the nasal mucosa (Fig. 3B). The levels of IL-4, IL-5, IL-6, and IL-10 were significantly increased in the OVA/Poly(I:C) groups, but remained lower than those of the positive control group. The OVA/Poly(I:C) groups exhibited significant increases in IL-7 and IFN-γ levels compared with the positive control. Although the differences between the nasal mucosal and splenocytic expression levels were small, the OVA/Poly(I:C) groups synthesized less Th2 cytokines and more Th1 and Th17 cytokines compared with the group challenged with OVA only.
IL-17 is produced principally by natural killer (NK) cells6 and CXCL1 is an important neutrophil chemoattractant.9 We thus evaluated NK cell infiltration and CXCL1 expression immunohistochemically. The positive control expressed little of the NK cell marker NKp46, whereas the OVA/Poly(I:C) (100 µg) group exhibited markedly higher expression (Fig. 4A), suggesting that NK cells might be associated with OVA/Poly(I:C)-induced inflammation, being the source of IL-17. We next showed that the OVA/Poly(I:C) high-dose group expressed a high level of the neutrophil chemoattractant CXCL1 (Fig. 4B).
We used double immunofluorescence staining with antibodies against NKp46 (Fig. 5A), CD4 (Fig. 5B), NIMP-R14 (Fig. 5C), and the major basic protein (MBP) of eosinophils (Fig. 5D). Confocal microscopy showed that NK cells, neutrophils, and CD4+ cells colocalized with IL-17. However, few MBP-expressing cells colocalized with IL-17. Thus, IL-17 may be produced by NK cells, CD4+ T cells, and/or neutrophils.
We developed a neutrophil-dominant murine model of AR via nasal instillation of Poly(I:C)-OVA, associated with increased IL-17 expression. Compared with the conventional OVA/alum model, our new model exhibits less of a Th2 phenotype (eosinophil infiltration and expression of Th2 cytokines, including IL-4 and IL-5) and a greater Th17-associated inflammatory response.
To date, most murine AR models have been Th2-driven eosinophil-dominant in nature;101112 few studies have described Th1- or Th17-dominant models. The OVA/alum combination is the most commonly used allergen/adjuvant pair driving the development of Th2-predominant AR in animals. However, AR is a disease spectrum; the etiologies vary. Both Th1 and Th17 responses may play important roles.1314 In addition, the etiology of non-allergic rhinitis remains unclear. Our new Th17-driven neutrophil-dominant model will aid in exploration of the mechanisms of rhinitis.
Poly(I:C) is a synthetic analog of double-stranded RNA, thus mimicking the replication intermediates present in cells infected with RNA viruses.15 Generally, Poly(I:C) induces significant increases in mucosal neutrophil levels in animal models of asthma,1617 hepatitis,18 and cholangitis.19 However, to the best of our knowledge, our rhinitis model is the first to use Poly(I:C). OVA/Poly(I:C) administration increased IL-17 production, consistent with other studies.20212223 IL-17 plays an important role in chronic inflammatory diseases, including upper airway conditions,20 triggering neutrophilic inflammation by inducing the production of neutrophil chemoattractants including CXCL8, CXCL1, and CXCL6.212223 We found that the level of CXCL1 was elevated in the OVA/Poly(I:C) groups; CXCL1 may recruit neutrophils to the nasal airway.
OVA/Poly(I:C) also elevated the level of the Th1 cytokine IFN-γ, which is also thought to contribute to neutrophil infiltration of the nasal mucosa. In an asthma model, neutrophil infiltration induced by Poly(I:C) required both IFN-γ and IL-17, showing that IFN-γ contributed to such infiltration.24
Airway neutrophil numbers are associated with disease severity and treatment resistance of chronic inflammatory respiratory diseases including asthma.252627 In asthmatics, IL-17 increases disease severity by triggering neutrophilic tissue inflammation, mucus production, and angiogenesis. However, any role of IL-17 in AR remains poorly understood; any association between IL-17 and AR severity requires further research. Some authors claim that the serum IL-17 level is associated with the clinical severity of AR.2 Our new model can thus be used to study the pathogenesis of rhinitis.
We used confocal microscopy to show that NK cells, neutrophils, and CD4+ T cells produced IL-17, consistent with previous reports. Several immune cells have been shown to produce IL-17; these include Th2 cells,28 macrophages,29 mast cells,30 neutrophils,31 and NK cells.32
In summary, we used Poly(I:C) to establish an IL-17-dominant murine rhinitis model which will be useful for further study of rhinitis. The neutrophil infiltration observed was dependent on IL-17; inhibition of IL-17 production may aid in rhinitis treatment.
Figures and Tables
Fig. 1
(A) Protocol for the induction of AR with OVA and Poly(I:C) in mice. The mice were divided into 6 groups: 1) 1 negative control group, 2) 1 positive control group (OVA/alum model), 3) 2 Poly(I:C) groups (10 or 100 µg), and 4) 2 OVA/Poly(I:C) groups (10 or 100 µg). The positive control group was injected with OVA and alum mixtures intraperitoneally (i.p.) on days 0, 7, and 14 for sensitization. In Poly(I:C) or OVA/Poly(I:C) groups, PBS/Poly(I:C) or OVA/Poly(I:C) mixtures were treated intranasally (i.n.) on days 0, 1, 2, 7, and 14. For challenge, PBS or OVA were administered intranasally on days 14 to 21. (B) Symptom score of nasal rubbing and sneezing for 15 minutes. (C) The total and OVA-specific immunoglobulin (IgE, IgG1, and IgG2a) levels in the serum. AR, allergic rhinitis; OVA, ovalbumin; Poly(I:C), polyinosinic-polycytidylic acid; PBS, phosphate-buffered saline; Ig, immunoglobulin. *P<0.05; **P<0.01; ***P<0.001.
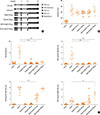
Fig. 2
Histological staining for inflammatory and IL-17A-producing cells in the nasal mucosa. (A) Eosinophil counts in the nasal mucosa (×400 magnification, Sirius red staining). (B) Neutrophil counts in the nasal mucosa (×400 magnification, IHC with NIMP-R14 antibody), and (C) IL-17A producing cell counts in nasal tissues (×400, IHC with IL-17A antibody). IL, interleukin; IHC, immunohistochemistry. *P<0.05; **P<0.01.
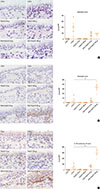
Fig. 3
(A) Local cytokine production in the nasal mucosa, mRNA expression (IL-4, IL-5, IL-6, IL-10, IL-17, and IFN-γ). (B) Systemic cytokine production from spleen cell culture. IL, interleukin; IFN, interferon. *P<0.05; **P<0.01; ***P<0.001.
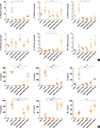
Fig. 4
IHC of (A) NKp46 and (B) CXCL1 (both ×400 magnification). IHC, immunohistochemistry; CXCL1, chemokine ligand 1. *P<0.05; **P<0.01; ***P<0.001.
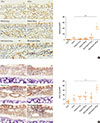
Fig. 5
Immunofluorescent double staining with (A) IL-17 (red)/NKp46 (green), (B) IL-17 (green)/CD4 (red), (C) IL-17 (green)/NIMP-R14 (red), and (D) IL-17 (red)/MBP (green) in the nasal mucosa. Blue indicates DAPI nuclear counterstaining. Orange colored portion in merged image means double positive cells. IL, interleukin; MBP, major basic protein; DAPI, 4′,6-diamidino-2-phenylindole.
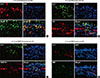
ACKNOWLEDGMENTS
This research was supported by Basic Science Research Program through the National Research Foundation of Korea (NRF) funded by the Ministry of Education (No. NRF-2016R1A2B4010407) and by a grant of the Korea Health Technology R&D Project through the Korea Health Industry Development Institute(KHIDI), funded by the Ministry of Health & Welfare, Republic of Korea (grant number : HI15C1524).
References
1. Weaver CT, Elson CO, Fouser LA, Kolls JK. The Th17 pathway and inflammatory diseases of the intestines, lungs, and skin. Annu Rev Pathol. 2013; 8:477–512.
2. Ciprandi G, De Amici M, Murdaca G, Fenoglio D, Ricciardolo F, Marseglia G, et al. Serum interleukin-17 levels are related to clinical severity in allergic rhinitis. Allergy. 2009; 64:1375–1378.
3. Quan SH, Zhang YL, Han DH, Iwakura Y, Rhee CS. Contribution of interleukin 17A to the development and regulation of allergic inflammation in a murine allergic rhinitis model. Ann Allergy Asthma Immunol. 2012; 108:342–350.
4. Halwani R, Al-Muhsen S, Hamid Q. T helper 17 cells in airway diseases: from laboratory bench to bedside. Chest. 2013; 143:494–501.
5. Vultaggio A, Nencini F, Pratesi S, Petroni G, Romagnani S, Maggi E. Poly(I:C) promotes the production of IL-17A by murine CD1d-driven invariant NKT cells in airway inflammation. Allergy. 2012; 67:1223–1232.
6. Lunding LP, Webering S, Vock C, Behrends J, Wagner C, Hölscher C, et al. Poly(inosinic-cytidylic) acid-triggered exacerbation of experimental asthma depends on IL-17A produced by NK cells. J Immunol. 2015; 194:5615–5625.
7. Jeon SG, Oh SY, Park HK, Kim YS, Shim EJ, Lee HS, et al. TH2 and TH1 lung inflammation induced by airway allergen sensitization with low and high doses of double-stranded RNA. J Allergy Clin Immunol. 2007; 120:803–812.
8. Samivel R, Kim DW, Son HR, Rhee YH, Kim EH, Kim JH, et al. The role of TRPV1 in the CD4+ T cell-mediated inflammatory response of allergic rhinitis. Oncotarget. 2016; 7:148–160.
9. Barnes PJ. New molecular targets for the treatment of neutrophilic diseases. J Allergy Clin Immunol. 2007; 119:1055–1062.
10. Saito H, Matsumoto K, Denburg AE, Crawford L, Ellis R, Inman MD, et al. Pathogenesis of murine experimental allergic rhinitis: a study of local and systemic consequences of IL-5 deficiency. J Immunol. 2002; 168:3017–3023.
11. Hellings PW, Hessel EM, Van Den Oord JJ, Kasran A, Van Hecke P, Ceuppens JL. Eosinophilic rhinitis accompanies the development of lower airway inflammation and hyper-reactivity in sensitized mice exposed to aerosolized allergen. Clin Exp Allergy. 2001; 31:782–790.
12. Hussain I, Randolph D, Brody SL, Song SK, Hsu A, Kahn AM, et al. Induction, distribution and modulation of upper airway allergic inflammation in mice. Clin Exp Allergy. 2001; 31:1048–1059.
13. Albano GD, Di Sano C, Bonanno A, Riccobono L, Gagliardo R, Chanez P, et al. Th17 immunity in children with allergic asthma and rhinitis: a pharmacological approach. PLoS One. 2013; 8:e58892.
14. Oboki K, Ohno T, Saito H, Nakae S. Th17 and allergy. Allergol Int. 2008; 57:121–134.
15. Stowell NC, Seideman J, Raymond HA, Smalley KA, Lamb RJ, Egenolf DD, et al. Long-term activation of TLR3 by poly(I:C) induces inflammation and impairs lung function in mice. Respir Res. 2009; 10:43.
16. Clarke DL, Davis NH, Majithiya JB, Piper SC, Lewis A, Sleeman MA, et al. Development of a mouse model mimicking key aspects of a viral asthma exacerbation. Clin Sci (Lond). 2014; 126:567–580.
17. Takayama S, Tamaoka M, Takayama K, Okayasu K, Tsuchiya K, Miyazaki Y, et al. Synthetic double-stranded RNA enhances airway inflammation and remodelling in a rat model of asthma. Immunology. 2011; 134:140–150.
18. Rhee SH, Im E, Riegler M, Kokkotou E, O’Brien M, Pothoulakis C. Pathophysiological role of Toll-like receptor 5 engagement by bacterial flagellin in colonic inflammation. Proc Natl Acad Sci U S A. 2005; 102:13610–13615.
19. Ambrosini YM, Yang GX, Zhang W, Tsuda M, Shu S, Tsuneyama K, et al. The multi-hit hypothesis of primary biliary cirrhosis: polyinosinic-polycytidylic acid (poly I:C) and murine autoimmune cholangitis. Clin Exp Immunol. 2011; 166:110–120.
20. Bullens DM, Decraene A, Seys S, Dupont LJ. IL-17A in human respiratory diseases: innate or adaptive immunity? Clinical implications. Clin Dev Immunol. 2013; 2013:840315.
21. Jones CE, Chan K. Interleukin-17 stimulates the expression of interleukin-8, growth-related oncogene-alpha, and granulocyte-colony-stimulating factor by human airway epithelial cells. Am J Respir Cell Mol Biol. 2002; 26:748–753.
22. Prause O, Laan M, Lötvall J, Lindén A. Pharmacological modulation of interleukin-17-induced GCP-2-, GRO-alpha- and interleukin-8 release in human bronchial epithelial cells. Eur J Pharmacol. 2003; 462:193–198.
23. Laan M, Lötvall J, Chung KF, Lindén A. IL-17-induced cytokine release in human bronchial epithelial cells in vitro: role of mitogen-activated protein (MAP) kinases. Br J Pharmacol. 2001; 133:200–206.
24. Shin TS, Lee BJ, Tae YM, Kim YS, Jeon SG, Gho YS, et al. Role of inducible nitric oxide synthase on the development of virus-associated asthma exacerbation which is dependent on Th1 and Th17 cell responses. Exp Mol Med. 2010; 42:721–730.
25. Gernez Y, Tirouvanziam R, Chanez P. Neutrophils in chronic inflammatory airway diseases: can we target them and how? Eur Respir J. 2010; 35:467–469.
26. Tillie-Leblond I, Gosset P, Tonnel AB. Inflammatory events in severe acute asthma. Allergy. 2005; 60:23–29.
27. Bafadhel M, McCormick M, Saha S, McKenna S, Shelley M, Hargadon B, et al. Profiling of sputum inflammatory mediators in asthma and chronic obstructive pulmonary disease. Respiration. 2012; 83:36–44.
28. Wang YH, Voo KS, Liu B, Chen CY, Uygungil B, Spoede W, et al. A novel subset of CD4(+) T(H)2 memory/effector cells that produce inflammatory IL-17 cytokine and promote the exacerbation of chronic allergic asthma. J Exp Med. 2010; 207:2479–2491.
29. Song C, Luo L, Lei Z, Li B, Liang Z, Liu G, et al. IL-17-producing alveolar macrophages mediate allergic lung inflammation related to asthma. J Immunol. 2008; 181:6117–6124.
30. Liu X, Jin H, Zhang G, Lin X, Chen C, Sun J, et al. Intratumor IL-17-positive mast cells are the major source of the IL-17 that is predictive of survival in gastric cancer patients. PLoS One. 2014; 9:e106834.
31. Taylor PR, Leal SM Jr, Sun Y, Pearlman E. Aspergillus and Fusarium corneal infections are regulated by Th17 cells and IL-17-producing neutrophils. J Immunol. 2014; 192:3319–3327.
32. Passos ST, Silver JS, O’Hara AC, Sehy D, Stumhofer JS, Hunter CA. IL-6 promotes NK cell production of IL-17 during toxoplasmosis. J Immunol. 2010; 184:1776–1783.