Abstract
Purpose
Although the concept of "one airway, one disease," which includes the middle ear space as part of the united airway is well recognized, the role of allergens in otitis media with effusion (OME) is not clearly understood. We aimed to investigate the effect of the interaction between Dermatophagoides farinae (Der f) and lipopolysaccharide (LPS) on the induction of epithelial inflammatory response in vitro.
Methods
Primary human middle ear epithelial cells were exposed to Der f, LPS, or both in different sequences, and the magnitude of the immunologic responses was compared. The mRNA expressiona of mucin (MUC) 4, 5AC, 5B, 8, GM-CSF, TNF-α, TLR4, and MD-2 were evaluated using real-time PCR. MUC levels before and after siRNA-mediated knockout of TLR4 and MD-2 were assessed. Lastly, the involved cell signaling pathway was evaluated.
Results
The expressiona of cytokines, and the MUC 4, 5AC, 5B, and 8 genes were augmented by pretreatment with Der f followed by LPS; however, reverse treatment or combined treatment did not induce the same magnitude of response. Increased MUC expression was decreased by TLR4 knockdown, but not by MD-2 knockdown. The signal intensity of MUC 8 was higher in MD-2 over-expressed cells than in those exposed to LPS only. The translocation of nuclear factor-κB was observed in cells pretreated with Der f followed by LPS.
"One airway, one disease" is a well-established concept that links the upper and lower airways. Anatomically, these 2 airways have common respiratory epithelium and similar mucosal susceptibility to disease. Epidemiologic studies have consistently shown that asthma and rhinitis often coexist in the same patients. Between 19% and 38% of patients with allergic rhinitis have coexisting asthma, and the prevalence rate of asthma is much higher in patients with allergic rhinitis than in the general population.12 In Korea, the prevalence of bronchial hyper-responsiveness was 55.7% and 25.5% in 6- to 15-year-old patients with allergic and non-allergic rhinitis, respectively.3
Currently, this concept is applied to the middle ear space, given that the middle ear space is an anatomical extension of the airway through the Eustachian tube and capable of mounting an allergic inflammatory response. A previous study showed the incidence of atopy was 24% in the study population comprising patients who had otitis media with effusion (OME) persisting for more than 3 months and unresponsiveness to antibiotics.4 Another study suggested that 35% of patients with recurrent OME have allergic rhinitis.5 Significantly higher numbers of eosinophils and T lymphocytes, as well as significantly higher levels of IL-4 and IL-5 mRNA+ cells, have been reported in patients with atopic OME than in non-atopic controls.6 Furthermore, other studies have shown increased expression of IL-5 and major basic proteins in the middle ear mucosa of patients with OME than that in normal controls, as well as higher levels of eosinophilic cationic proteins in the supernatant of middle ear effusion of atopic patients with OME than in that of non-atopic controls.78 These findings support the concept that the middle ear may be part of the united airway and may behave similarly to the nose or lung under allergic inflammatory conditions. Although allergy has been considered one of the most important factors of OME based on clinical observations, the role of allergy has not been investigated extensively and is easily ignored. In general, the possible integration of the middle ear as part of the united airway concept will have major clinical implications for the diagnosis and management of allergic airway disease.
Dermatophagoides pteronyssinus (Der p) and Dermatophagoides farinae (Der f) are the most common house dust mite (HDM) species, which produce allergens that are widely distributed in Korea. Recently, Der p and Der f have been shown to activate toll-like receptors (TLRs) 2 or 4 and thereby stimulate the innate immune response. TLRs recognize repetitive patterns in diverse microbes, including gram-positive and gram-negative bacteria and viruses, and they are key components of innate immunity.910 In the innate immune response, airway epithelial cells aid the immune system by inducing recruitment of immune-competent cells to local tissues and thereby modulating their activity.11 In addition, lipopolysaccharide (LPS), a major component of the outer membrane of gram-negative bacteria, is a virulence factor that can induce inflammatory responses. LPS may induce the release of multiple pro-inflammatory cytokines and chemokines from airway epithelial cells, and this initial inflammatory response plays a key role in the containment of infection. The inflammatory response induced by LPS in epithelial cells has been linked to TLR 4.12
The airway mucosa is lined with ciliated columnar epithelium interspersed with goblet cells. Accumulation of secretions and mucostasis in the airway mucosa due to allergic airway disease can alter the viscoelasticity of the mucus, ciliary motility, and epithelial defense mechanisms, thereby increasing the susceptibility to various stimuli, such as bacteria and viruses.13 Viruses are clinically linked to allergy: viral infection can induce asthma/allergic exacerbations, and viral clearance and persistence of symptoms are prolonged in allergic individuals.141516 Conversely, chronic allergic inflammation may impair anti-viral reaction to acute rhinovirus infection, as indicated by the suppression of IFN-α, IFN-γ, and IL-12 induction.17 Meanwhile, airborne LPS might adversely affect asthmatic individuals by enhancing an established airway inflammation and airway obstruction.1819
Considering the above findings, it is natural to think that epithelial cells of the middle ear might be involved in recognizing and formulating an innate immune response to Der f and LPS, and a kind of reciprocal effect may be developed by subsequent exposure to Der f and LPS. Although allergy or allergen sensitization could be a concealed, potent stimulant of inflammation in sinus and middle ear disease, the effect of allergen sensitization on infection and its mechanism in airway epithelium is not clearly understood. Moreover, although allergy has been implicated in OME, the role of middle ear epithelial cells in the innate immune response has not been characterized. Thus, this study was design to investigate whether allergen pre-sensitization within airway mucosa can affect subsequent viral or bacterial infection. For this, we performed in vitro experiments using primary human middle ear epithelial cells (HMEECs) and assessed the signaling pathways activated by Der f and LPS as well as the production of pro-inflammatory cytokines and the expression of the mucin (MUC) gene.
LPS, SB203580, and Bay were obtained from Sigma-Aldrich (Munich, Germany). Der f crude body extract was purchased from Arthropods of Medical Importance Resource Bank (Seoul, Korea). Other chemicals used were of the purest grade available from Sigma (St. Louis, MO, USA).
HMEECs (kindly provided by Dr. David J. Lim, House Ear Institutes, Los Angeles, CA, USA) were maintained in a mixture of Dulbecco's modified Eagle's medium (DMEM, Invitrogen, Carlsbad, CA, USA) and bronchial epithelial basal medium (Lonza, Walkersville, MD, USA) (1:1).202122 Cells were grown to 60% confluence in 100-mm culture plates and kept at 37℃ in a carbon dioxide-enriched (95% air, 5% CO2) humidified atmosphere.
HMEECs were seeded onto 60-mm culture plates, with 2.2×105 cells per well for the experimental condition. Predeter-mined non-cytotoxic doses of Der f or LPS by MTT (3-(4,5-dimethylthiazol-2-yl)-2,5-diphenyltetrazolium bromide) assay (data not shown) were applied to HMEECs. By performing the pilot study using real-time PCR, treatment with 1.0 µg/mL LPS for 24 hours was identified to be the optimal and effective in that it not only elicits a moderate level of inflammatory response but also has an augmentative effect following the administration of 2.0 µg/mL Der f. Cells were then stimulated in several ways: 1) Der f 2.0 µg/mL only for 48 hours (Df48h); 2) LPS 1.0 µg/mL only for 24 hours (LPS24h); 3) Der f 2.0 µg/mL for 48 hours and add LPS 1.0 µg/mL for 24 hours (Df48h/LPS24h); 4) LPS 1.0 µg/mL for 48 hours and add Der f 2.0 µg/mL for 24 hours (LPS48h/Df24h); or 5) Der f 2.0 µg/mL and LPS 1.0 µg/mL both simultaneously for 48 hours (Df48h/LPS48h) (Fig. 1).
Total RNA was isolated from the epithelial cells using TRIzol (Invitrogen) as per the manufacturer's instructions and reverse transcribed into cDNA using the Quantitect Reverse Transcription kit (Qiagen, Venlo, Netherlands). Then qRT-PCR analyses were performed using a 7,500 FAST qRT-PCR System (Applied Biosystems, Foster City, CA, USA). Each reaction mixture contained 10 µL of SYBR® Green PCR Master Mix (Applied Biosystems), 4 pmol each of the forward and reverse primers, and 1 µL of cDNA in a final volume of 20 µL. Reaction mixtures were incubated at 95℃ for 5 minutes to activate FastStartTaq DNA Polymerase, followed by 40 cycles of amplification. Data were analyzed using Sequence Detection Software version 1.9.1 (Applied Biosystems). Target mRNA expression was normalized to glyceraldehyde-3-phosphate dehydrogenase (GAPDH) expression and calculated using the comparative Ct method. Primers used in this study are shown in Table.
Cells were transfected with control small interfering (si) RNA, TLR 4 siRNA or myeloid differentiation (MD)-2 siRNA, and 10 µL of Lipofectamine RNAiMAX (Invitrogen) in 60-mm plates as per the manufacturer's protocol. The plasmid pFlag-CMV1-hMD2 was a kind gift from Doug Golenbock (Addgene plasmid #13028). After cells were washed with OPTI-MEM (Gibco, Carlsbad, CA, USA), DNA was transfected to cells using X-treme GENE HP-DNA transfection reagent (Roche Diagnostics, Indianapolis, IN, USA) as per the supplier's protocol. After 4 hours of incubation, the medium was exchanged for a complete medium containing 10% serum and antibiotics. Cells were incubated for an additional 24 hours and treated with Df or LPS. Cell viability was measured by light microscopy, and the gene-silencing or expression efficacy was evaluated by evaluating the mRNA levels. The siRNAs were NM_138554.2 for TLR4 and NM_014364.2 for MD-2 (Bioneer, Daejeon, Korea).
At specific time points after the Der f or LPS treatment, the medium was removed, and cells were washed with phosphate-buffered saline (PBS; 10 mM, pH 7.4). Cells were then lysed with lysis buffer (50 mM Tris pH 7.7, 150 mM NaCl, 1% NP-40, 5 mM EGTA, 50 mM -glycerophosphate, 20 mM NaF, 1 mM Na3VO4, 2 mM phenylmethylsulfonyl fluoride, 10 mg/mL leupeptin, and 10 mg/mL aprotinin) and incubated for 20 minutes at 4℃. Briefly after sonication, cells were centrifuged at 13,000×g for 10 minutes at 4℃. The supernatant containing the total cell lysate was collected. Protein concentration of the lysates was measured using the Bio-Rad Protein Assay (Bio-Rad, Hercules, CA, USA). Equal amounts of protein were mixed with sample buffer (62.5 mM Tris–HCl [pH 6.8], 1% sodium dodecyl sulfate, 2.5% glycerol, 0.5% 2-β-mercaptoethanol, and bromophenol blue), boiled for 5 minutes, and separated by electrophoresis on 10%-12% Tris-HCl gels. Protein content of the gels was transferred to a PVDF membrane (Amersham, Buckinghamshire, UK), and the membranes were blocked with TBS-T (20 mM Tris, 500 nM NaCl, with 0.1% Tween-20) containing 5% (w/v) skim milk for 1 hour at room temperature. Membranes were probed with antibodies against MUC 8 (Sigma–Aldrich, St. Louis, MO, USA), TLR-4, p-p38, p38, p-CREB (Santa Cruz Biotechnology, Dallas, TX, USA) and GAPDH (Santa Cruz Biotechnology) followed by peroxidase-conjugated anti-mouse IgG or anti-rabbit IgG (Jackson Immuno Research, West Grove, PA, USA).Membranes were developed using the enhanced chemiluminescent analysis system (SuperSignal® West Pico Chemiluminescent Substrate, Pierce, Waltham, MA, USA) and the signal was captured using an image reader (LAS4000; Fuji Photo Film, Tokyo, Japan). Results were obtained from three independent experiments.
HMEECs were transfected with siRNA-TLR4, MD-2, and control, and treated with Der f 24h and then with LPS24h on a cover slide in 12-well plates. Cells were rinsed 3 times with PBS, fixed with 4% paraformaldehyde for 10 minutes at room temperature, and rinsed again. Cells were then blocked with 1% bovine serum albumin, followed by the addition of the primary antibodies anti-Der f and anti-TLR4. After extensive washing with PBS, fluorescein isothiocyanate-conjugated IgG was added. Following incubation, the slides were rinsed, mounted, and viewed at 488 nm on a confocal microscope (FV1000, Olympus, Japan).
Statistical analyses were performed using SPSS for Windows (Ver. 12.0, SPSS Inc., Chicago, IL, USA). All data are expressed as mean ± standard deviation (SD). A one-way analysis of variance (ANOVA) was used to determine statistical significance differences between control and groups at each time or dose point. Scheffe's F test was used to correct for multiple comparisons when statistically significant differences were identified in the ANOVA. A P value of <0.05 was considered statistically significant.
Treatment with Df48h increased the level of MUC 8 mRNA expression. The expression level was significantly augmented when cells were exposed to Df48h/LPS24h. We further analyzed the interaction of Der f and LPS under different treatment conditions. When we treated the cells in either with 1) LPS48h/Df24h or 2) Df48h/LPS48h, MUC8 mRNA expression did not reach the levels induced by Df48h/LPS24h (Fig. 2A). With regard to the MUC 4, 5AC and 5B mRNA expression levels increased in a similar fashion, showing the greatest increase in the cells treated Df48h/LPS24h without statistical significance (Fig. 2B). This pattern was confirmed at the protein level. Immunoblot assay showed that Df48h/LPS24h synergistically elevated the MUC 8 protein production; however, this was not observed in Df48h or LPS24h (Fig. 3A). Next, we analyzed the magnitude of the synergistic effect of both Der f and LPS by evaluating the MUC 8 and TLR 4 protein expression levels, because both Der f and LPS are known to bind to TLR 4 to trigger the inflammatory signaling pathway. To further demonstrate the synergistic effect of Der f and LPS, we treated HMEECs with LPS 2, 4, 6, 8, and 10 µg/mL each for 24 hours, and then compared the intensity of MUC8 and TLR 4 proteins produced by Df48LPS24 treatment. Df48h/LPS24h showed a higher intensity than that produced by LPS24h only (>10 µg/mL) (Fig. 3B). In terms of mRNA expression of pro-inflammatory cytokines, such as IL-1ß, GM-CSF, IL-33, and TNF-α, the augmented pattern was also similar to that of MUC, although they did not reach statistical significance (Fig. 4). These results suggest a possibility that Der f and LPS can act synergistically.
To elucidate the mechanism underlying the difference in mucin gene expression in cells treated with LPS48h/Df24h or Df24h/LPS48h, we further determined the roles of TLR 4 and MD-2. We therefore transfected HMEECs with siRNAs to knock down the expression of TLR4 or MD-2. Suppression of TLR4 and MD-2 by each siRNA were confirmed by real-time PCR. CD14 expression was also suppressed by both siRNA without statistical significance (Fig. 5A, upper panel). Expressions of MUC 4, 5B and 8 mRNAs by the treatment with Df48h/LPS24h decreased significantly upon siRNA-TLR4 transfection. However, a significant amount of mRNAs were still expressed in siRNA-MD-2 transfected HMEECs (Fig. 5A, lower panel). Immunoblot analysis further confirmed decreased or sustained increase in MUC 8 protein signals in siRNA-TLR4- and siRNA-MD-2-transfected cells, respectively (Fig. 5B). These results indicated that TLR 4 could be partially activated in the absence of MD-2 protein.
Our above mentioned results suggest the possibility of Der f being a functional substitute for the MD-2 protein; thus, the TLR 4 signal could be activated without the help of MD-2 protein. Therefore, to determine the exact role of MD-2 in Der f and LPS synergism, a mammalian expression vector containing MD-2 (pCMV1-hMD-2) was transfected into HMEECs for transient expression studies. MD-2 was over-expressed in HMEECs subsequently exposed to LPS for 24 hours, which showed a higher MUC 8 protein signal density than those exposed to LPS only and similar MUC 8 protein signals compared to those exposed to Df48h/LPS24h (Fig. 5C). Moreover, we conducted the co-immunofluorescence staining of TLR 4 and Der f in siRNA-treated cells. Der f and TLR 4 were co-localized not only in the siRNA-negative control transfected cells but also in the siRNA-MD-2 transfected cells (Fig. 6A and B). These results suggest that the MD-2 protein may play an important role in the pro-inflammatory Der f and LPS synergism.
To further investigate the mechanism underlying the synergistic functions of Der f and LPS, we treated cells with various inhibitors, such as SB203580 (a bicyclic inhibitor of p38), PD98059 (a specific inhibitor of MAPK/MEK-1), Bay (a NF-κB inhibitor), LY294002 (PI3K inhibitor), and a JNK inhibitor (data not shown). Cells were treated with each inhibitor 2 hours prior to LPS treatment. Of these, the mRNA expression levels of MUC 2, 5AC, and 8 decreased significantly under SB203580 or Bay treatment (Fig. 7A). MUC 8 protein signal intensity was lower in SB203580-or Bay-treated cells than in Df48h/LPS24h cells and most lowest in Df48h/LPS48h cells (Fig. 7B). This suggests that p38 MAPK and NF-κB transcriptional factors may be engaged in the synergism of Der f and LPS. The p-p38 signal post LPS treatment peaked at 5 minutes, and the expression of p-p38 and p-CREB was inhibited by SB203580 (Fig. 8A). GAPDH was constitutively expressed and not affected by SB203580. Moreover, NF-κB translocation was seen in Df48h/LPS24h by confocal microscopy (Fig. 8B).
We investigated the synergistic effect of Der f and LPS on MUC gene expression and pro-inflammatory cytokine production in HMEECs. LPS is known to induce and potentiate MUC gene expression;3 however, the interaction between LPS and Der f has not been studied so far. Before starting the experiment, to rule out possible endotoxin contamination of Der f crude body extract, we first measured the endotoxin level via the LAL method (Toxin Sensor chromogenic LAL Endotoxin assay kit, Gen Script, Piscataway, NJ, USA). The endotoxin level in Der f crude body extract was found to be 0.16 EU/mL, which was considered negligible. In this study, Der f and LPS synergistically induced MUC 8 gene expression and pro-inflammatory cytokines, and this effect depends on the sequence of exposure, i.e., it only occurs when Der f stimuli precedes LPS stimuli. Additionally, pre-treated Der f is likely to function as an MD-2 protein and induce p-p38 and p-CREB expressions. These findings provide evidence for the interaction between 2 environmental stimuli associated with allergic airway disease and infectious diseases in the airway epithelium.
We measured mucin gene production as the final product because it is one of the major molecules that the airway epithelium produces in large amounts in response to several stimuli, such as TNF-α, IL-1β, LPS, oxidative stress, and neutrophil elastase, and because it has been implicated in numerous airway diseases.24 Mucins are broadly classified as either cell membrane-bound or secreted; notably, MUC2, MUC5AC, MUC5B, MUC6, MUC7, MUC8, MUC9, and MUC19 are categorized as secreted mucins.2526 MUC5AC and MUC5B are predominant in the sinus and nasal cavity, respectively, and considered primarily responsible for the gel-like characteristics of mucoid middle ear fluids.27282930 The MUC 8 gene is over-expressed in chronic rhinosinusitis and nasal polyp epithelium, and its expression levels are also increased by inflammatory mediators in nasal epithelial cells.3132 Airway mucus obstruction is a shared feature among lower airway diseases, such as cystic fibrosis, asthma, and chronic obstructive lung disease.33 Hence, investigating the mucin gene expression in HMEECs is considered an appropriate approach to evaluating the "extended one airway concept."
Interactions between allergens, viruses, bacteria and/or other pro-inflammatory stimuli have been previously reported.3 The synergistic effects of human rhinovirus and nitric oxide 2 on IL-8 release and an additive effect on ICAM-1 expression have been demonstrated.34 The interaction between the human rhinovirus or respiratory syncytial virus and Der p I has been studied, and it has been found that they synergistically induce IL-8 expression in bronchial epithelial cells.35 With regard to the interaction between bacteria and allergens, a previous study has shown that systemic LPS administration suppresses early and late allergic reactions in vivo via the TLR4-dependent pathway that triggers nitric oxide synthase 2 activity in a murine model of asthma;36 the generation of Th1 or Th2 responses is found to be dependent on the dose of LPS. At a low LPS level, inhaled LPS induces a Th2 response to inhaled antigens, whereas at a high level, inhaled LPS results in a Th1 response to the antigen.37 In an HDM allergen-evoked asthma murine model, LPS dose-dependently inhibits HDM-induced eosinophilic recruitment into the lungs, mucus production in the airways, and production of Th2 cytokines (IL-4, IL-5, IL-10, and IL-13).38 However, the interaction between Der f and LPS at a cellular level has not been addressed previously, especially in the middle ear epithelium in vivo or in vitro, despite being frequently implicated in middle ear disease pathogenesis.
It is well known that co-molecules, such as MD-2, CD14, myD88, and LPS-binding protein are needed to activate the TLR 4 signaling pathway; in particular, MD-2, a glycoprotein co-expressed with TLR4 at the surface of various cell types, has received recent attention. Theoretically, to transmit the LPS-driven inflammatory signals, LPS bound to LBP is shuttled to CD14, which transfers the LPS to an MD-2 molecule. Activation of MD-2 occurs in 2 ways: it combines with endogenously expressed TLR-4 to increase the surface expression of TLR-4 and MD-2,39 and participates directly in endotoxin recognition by extracting the endotoxin from monomeric endotoxin:CD14 complex.40
Previous studies demonstrate that MD-2 is essential for LPS-driven inflammatory responses, and that lack of MD-2 protein expression in human corneal epithelial cells account for the lack of response to LPS exposure in the cornea.41 MD-2 binds to TLR 4 and greatly enhances the response of TLR4-transfected cells to LPS exposure.42 Patients with the single nucleotide polymorphisms of MD-2 promoter increase mRNA expressions of MD-2 and tended to have high levels of IL-1β, IL-6, IL-8, IL-10, and tumor necrosis factor (TNF)-α after Der p 2 and LPS stimulation.43 These facts prompted us to investigate whether the MD-2 protein might have a specific role in allergen and LPS synergism in HMEECs. In our study, to evaluate the role of MD-2, we knocked-down the MD-2 gene by transfecting siRNA-MD-2. Interestingly, the production of MUC genes was not completely decreased in the absence of MD-2. Moreover, pre-treated Der f did not significantly alter the MD-2 mRNA expression level (data not shown). Overexpression of the MD-2 gene using the pCMV-hMD-2 vector had a similar induction effect of MUC 8 protein when followed by LPS exposure for 24 hours. This implies that Der f may combine with LPS, TLR4, and CD14, and have a role in endotoxin recognition in cases of deficiency of the MD-2 protein. Notably, the crystal structures of MD-2 and Der p 2 exhibit structural homology with 2 anti-parallel β-pleated sheets stabilized by disulfide bonds.444546 In an in vivo study, airway sensitization and Der p 2 challenge resulted in allergic asthma in wild-type and MD-2-deficient mice, but not in TLR4-deficient mice.47 A previous study stated that human airway epithelium internalizes Der p2, which in turn, increases the endogenous cellular expression of MD-2.48 Der p 2 has not only structural homology but also functional homology with MD-2, facilitating signaling through direct interactions with the TLR4 complex and reconstituting LPS-driven TLR4 signaling in the absence of MD-2.47 Taken together, these facts suggest that Der f may have functional homology with MD-2.
Meanwhile, there are several additional factors that may influence augmented mucin gene expression induced by Der f and LPS. Notably, at least 3 different microbial pathogen-associated molecular patterns can be detected in mite feces and/or in the mite environment: LPS, β-glucan, and chitin. Both LPS and β-glucan mediate innate immunity through reactive oxygen species production and can subsequently affect the mucin gene production.49 The endotoxin level of Der f crude body extract used in this study was 0.16 EU/mL, and thus the contribution might not be significant. Chitin can polarize Th1, Th2, and Th17 immune responses, and recruit IL-4 positive innate immune cells, including eosinophils and basophils;5051 however, its precise contribution in the HDM-LPS synergism remains to be elucidated.
HDM allergenic proteins can be categorized into 4 main families: proteases, proteins displaying affinity for lipids, non-proteolytic enzymes, and non-enzymatic components.52 In the context of innate immunity activation, all these protein and non-protein compounds could putatively participate in stimulation. Among them, group 2 HDM allergen is described as an MD2-like lipid binding protein based on its structural/sequence homology,53 supporting the hypothesis of our study.
Finally, the lack of synergism when the HMEECs were treated with Der f and LPS simultaneously (Df48h/LPS48h) or LPS48h/Df24h may be attributed to the following aspects. First, it has been noted that Der f binds to LPS with a molar ratio of 1:1.54 Thus, when treated simultaneously, a fraction of these molecules may bind before reaching and binding to TLR4 and thus cannot activate the signaling pathway as expected. Second, an insufficient number of TLR 4 on the cell surface or endogenous MD-2 protein may interact with Der f and LPS when they are added at the same time. Third, the "hygiene hypothesis" states that the high levels of exposure to bacterial products, such as LPS, early in life are inversely correlated with the development of atopy and allergic disease.555657 Thus, it is thought that LPS exposure leads to the exhaustion of TLR 4 and the development of a counter-regulatory response.58 Finally, unlike in LPS48h/Df24-treated cells, Der f would act like MD-2 protein in Df48h/LPS24h-treated cells, more signal activity could be triggered.
In summary, these experiments demonstrate that Der f and LPS can act synergistically when Der f exposure precedes LPS exposure in HMEECs. Based on our findings, the likely underlying mechanism is that Der f has functions similar to those of MD-2, combining with TLR-4 and participating in endotoxin recognition. Such synergy suggests its important role in the development of OME in patients with concealed allergen-sensitized airway epithelium. Thus, targeted inactivation of innate immune signals to allergen exposure may be useful for the development of specific therapeutics for otitis media in concealed allergic sensitization.
Figures and Tables
![]() | Fig. 1Study design. Cells were treated with medium only, a pre-determined, non-cytotoxic dose of Der f for 24/48 h or LPS for 24/48 h, or both in different sequences or simultaneously as indicated. UT, untreated; Df, Dermatophagoides farina; LPS, lipopolysaccharide; HMEEC, human middle ear epithelial cell. |
![]() | Fig. 2Real-time polymerase chain reaction to measure mucin mRNA level. (A) The expression of MUC 8 mRNA level was higher in cells exposed to Der f first and then to LPS than in cells exposed to Der f or LPS alone. The mRNA level did not increase when the cells were first exposed to LPS and then to Der f or were exposed to both agents simultaneously. (B) The levels of MUC 4, 5AC, and 5B mRNA showed similar patterns to MUC 8. (*P<0.05). |
![]() | Fig. 3Immunoblotting to determine MUC 8 and Toll-like receptor 4 signal intensity. (A) Treatment with Der f followed by LPS had a synergistic effect and increased MUC 8 production. (B) The signal intensity of MUC 8 and Toll-like receptor 4 induced by combined treatment with Der f and LPS was higher than that induced by treatment with LPS alone. |
![]() | Fig. 4Real-time PCR to measure the levels of various cytokine mRNAs. The mRNA levels of pro-inflammatory cytokines IL-1b, IL-33, GM-CSF, and TNF-α tended to increase when the cells were exposed to the Der f initially and then to LPS. |
![]() | Fig. 5mRNA and protein levels in cells transfected with small interfering RNA or in those overexpressing MD-2. (A, upper panel) The level of MD-2 or TLR4 mRNA was decreased in cells transfected with small interfering (si) RNA-MD-2 and siRNA-TLR4, respectively. (A, lower panel) The expressions of MUC 4, 5B, and 8 mRNA were also decreased in si-RNA-transfected cells. However, unlike in the cells transfected with si-RNA-TLR4, the expression of the target genes was not completely abolished in cells transfected with si-RNA-MD-2. (B) The decreased expression level of TLR4 and MUC 8 was confirmed at the protein level. The signal of MUC 8 protein was observed not only in the negative control, but also in the si-RNA-MD-2–transfected cells. (C) Overexpression of MD-2 enhanced the LPS-driven production of MUC 8. The signal intensity of MUC 8 was higher when LPS was added to the cell transfected with the Flag-pCMV-hMD-2 vector than with vector only. The pattern of increase when the cells were treated with LPS only for 24 hours was similar to that observed when those were treated first with Der f and then with LPS (Df48h/LPS24h). |
![]() | Fig. 6Confocal laser scanning image. (A) Red fluorescence indicates Der f expression; green, TLR4 expression; and blue, nuclear location. The lower right panel is a merged image of the other 3 panels. TLR4 was mainly expressed in the cell membrane, and it can be seen as yellow fluorescence after the overlap, suggesting that Der f and TLR4 co-localized in the cell membrane. Scale bar, 40 µm. (B) In high magnification, yellow fluorescence is seen not only in the negative control, but also in the membrane of cells transfected with si-RNA MD-2. Scale bar, 10 µm. |
![]() | Fig. 7Inhibition of MUC gene mRNA expression by SB203580 and Bay. (A) Expression of MUC 2, 5AC, and 8 mRNA was down-regulated by treatment with SB 203580 and Bay. (B) Down-regulation of MUC8 protein expression was confirmed by immunoblotting. Results are representative of those obtained from 3 independent experiments. |
![]() | Fig. 8Inhibition of phosphorylation of p38 MAPK and CREB, and translocation of nuclear factor κB (NF-κB) by SB203580 and Bay in HMEECs stimulated with Der f + LPS. (A) HMEECs were treated with Der f for 24 hours and then with SB203580 2 hours prior to LPS treatment. Subsequently, the cells were treated with LPS for 5, 15, and 30 minutes in the presence of Der f. The phosphorylation of p38 MAPK and CREB was analyzed using Western blotting. (B) Localization of NF-κB p65 was visualized under a fluorescence microscope after immunofluorescence staining with NF-κB p65 antibody (green). Cells were stained with DAPI to visualize nuclei (blue). NF-κB translocation in HMEECs stimulated with Der f + LPS was inhibited by treatment with SB203580 and Bay. Results are representative of those obtained from 2 independent experiments. |
Table
Primers for real-time polymerase chain reaction
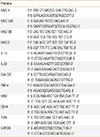
References
1. Blair H. Natural history of childhood asthma. 20-year follow-up. Arch Dis Child. 1977; 52:613–619.
2. Pedersen PA, Weeke ER. Asthma and allergic rhinitis in the same patients. Allergy. 1983; 38:25–29.
3. Kim SW, Han DH, Lee SJ, Lee CH, Rhee CS. Bronchial hyperresponsiveness in pediatric rhinitis patients: the difference between allergic and nonallergic rhinitis. Am J Rhinol Allergy. 2013; 27:e63–e68.
4. Döner F, Yariktas M, Demirci M. The role of allergy in recurrent otitis media with effusion. J Investig Allergol Clin Immunol. 2004; 14:154–158.
5. Bernstein JM, Lee J, Conboy K, Ellis E, Li P. Further observations on the role of IgE-mediated hypersensitivity in recurrent otitis media with effusion. Otolaryngol Head Neck Surg. 1985; 93:611–615.
6. Sobol SE, Taha R, Schloss MD, Mazer BD, Manoukian JJ, Tewfik TL, et al. T(H)2 cytokine expression in atopic children with otitis media with effusion. J Allergy Clin Immunol. 2002; 110:125–130.
7. Wright ED, Hurst D, Miotto D, Giguere C, Hamid Q. Increased expression of major basic protein (MBP) and interleukin-5(IL-5) in middle ear biopsy specimens from atopic patients with persistent otitis media with effusion. Otolaryngol Head Neck Surg. 2000; 123:533–538.
8. Hurst DS, Venge P. Evidence of eosinophil, neutrophil, and mast-cell mediators in the effusion of OME patients with and without atopy. Allergy. 2000; 55:435–441.
9. Ozinsky A, Underhill DM, Fontenot JD, Hajjar AM, Smith KD, Wilson CB, et al. The repertoire for pattern recognition of pathogens by the innate immune system is defined by cooperation between toll-like receptors. Proc Natl Acad Sci U S A. 2000; 97:13766–13771.
10. Akira S, Takeda K, Kaisho T. Toll-like receptors: critical proteins linking innate and acquired immunity. Nat Immunol. 2001; 2:675–680.
11. Kitajima T, Muroi M, Yamashita N, Tanamoto K. Toll-like receptors required for dermatophagoides farinae to activate NF-κB. Biol Pharm Bull. 2014; 37:74–80.
12. Chow JC, Young DW, Golenbock DT, Christ WJ, Gusovsky F. Toll-like receptor-4 mediates lipopolysaccharide-induced signal transduction. J Biol Chem. 1999; 274:10689–10692.
13. Vlastos I, Athanasopoulos I, Mastronikolis NS, Panogeorgou T, Margaritis V, Naxakis S, et al. Impaired mucociliary clearance in allergic rhinitis patients is related to a predisposition to rhinosinusitis. Ear Nose Throat J. 2009; 88:E17–E19.
14. Papadopoulos NG, Xepapadaki P, Mallia P, Brusselle G, Watelet JB, Xatzipsalti M, et al. Mechanisms of virus-induced asthma exacerbations: state-of-the-art. A GA2LEN and InterAirways document. Allergy. 2007; 62:457–470.
15. Papadopoulos NG, Christodoulou I, Rohde G, Agache I, Almqvist C, Bruno A, et al. Viruses and bacteria in acute asthma exacerbations--a GA2 LEN-DARE systematic review. Allergy. 2011; 66:458–468.
16. Copenhaver CC, Gern JE, Li Z, Shult PA, Rosenthal LA, Mikus LD, et al. Cytokine response patterns, exposure to viruses, and respiratory infections in the first year of life. Am J Respir Crit Care Med. 2004; 170:175–180.
17. Rochlitzer S, Hoymann HG, Müller M, Braun A; U-BIOPRED consortium. No exacerbation but impaired anti-viral mechanisms in a rhinovirus-chronic allergic asthma mouse model. Clin Sci (Lond). 2014; 126:55–65.
18. Rizzo MC, Naspitz CK, Fernández-Caldas E, Lockey RF, Mimiça I, Solé D. Endotoxin exposure and symptoms in asthmatic children. Pediatr Allergy Immunol. 1997; 8:121–126.
19. Michel O, Kips J, Duchateau J, Vertongen F, Robert L, Collet H, et al. Severity of asthma is related to endotoxin in house dust. Am J Respir Crit Care Med. 1996; 154:1641–1646.
20. Song JJ, Lee JD, Lee BD, Chae SW, Park MK. Effect of diesel exhaust particles on human middle ear epithelial cells. Int J Pediatr Otorhinolaryngol. 2012; 76:334–338.
21. Preciado D, Kuo E, Ashktorab S, Manes P, Rose M. Cigarette smoke activates NFκB-mediated Tnf-α release from mouse middle ear cells. Laryngoscope. 2010; 120:2508–2515.
22. Jun HJ, Lim HW, Choi J, Jung HH, Chae SW. Ciglitazone inhibits cigarette smoke solution-induced inflammatory responses in human middle ear epithelial cells. Int J Pediatr Otorhinolaryngol. 2012; 76:1136–1139.
23. Smirnova MG, Guo L, Birchall JP, Pearson JP. LPS up-regulates mucin and cytokine mRNA expression and stimulates mucin and cytokine secretion in goblet cells. Cell Immunol. 2003; 221:42–49.
24. Voynow JA, Gendler SJ, Rose MC. Regulation of mucin genes in chronic inflammatory airway diseases. Am J Respir Cell Mol Biol. 2006; 34:661–665.
25. Rose MC. Mucins: structure, function, and role in pulmonary diseases. Am J Physiol. 1992; 263:L413–L429.
26. Rose MC, Voynow JA. Respiratory tract mucin genes and mucin glycoproteins in health and disease. Physiol Rev. 2006; 86:245–278.
27. Kerschner JE. Mucin gene expression in human middle ear epithelium. Laryngoscope. 2007; 117:1666–1676.
28. Giebink GS, Le CT, Paparella MM. Epidemiology of otitis media with effusion in children. Arch Otolaryngol. 1982; 108:563–566.
29. Thai P, Loukoianov A, Wachi S, Wu R. Regulation of airway mucin gene expression. Annu Rev Physiol. 2008; 70:405–429.
30. Alenmyr L, Herrmann A, Högestätt ED, Greiff L, Zygmunt PM. TRPV1 and TRPA1 stimulation induces MUC5B secretion in the human nasal airway in vivo. Clin Physiol Funct Imaging. 2011; 31:435–444.
31. Cho KN, Choi JY, Kim CH, Baek SJ, Chung KC, Moon UY, et al. Prostaglandin E2 induces MUC8 gene expression via a mechanism involving ERK MAPK/RSK1/cAMP response element binding protein activation in human airway epithelial cells. J Biol Chem. 2005; 280:6676–6681.
32. Lee HM, Kim DH, Kim JM, Lee SH, Hwang SJ. MUC8 mucin gene up-regulation in chronic rhinosinusitis. Ann Otol Rhinol Laryngol. 2004; 113:662–666.
33. Yoshida T, Tuder RM. Pathobiology of cigarette smoke-induced chronic obstructive pulmonary disease. Physiol Rev. 2007; 87:1047–1082.
34. Spannhake EW, Reddy SP, Jacoby DB, Yu XY, Saatian B, Tian J. Synergism between rhinovirus infection and oxidant pollutant exposure enhances airway epithelial cell cytokine production. Environ Health Perspect. 2002; 110:665–670.
35. Foster S, Bedford KJ, Gould ME, Coward WR, Hewitt CR. Respiratory syncytial virus infection and virus-induced inflammation are modified by contaminants of indoor air. Immunology. 2003; 108:109–115.
36. Rodríguez D, Keller AC, Faquim-Mauro EL, de Macedo MS, Cunha FQ, Lefort J, et al. Bacterial LPS signaling through TLR4 suppresses asthma -like response via NP synthase 2 activity. J Immunol. 2003; 171:1001–1008.
37. Eisenbarth SC, Piggott DA, Huleatt JW, Visintin I, Herrick CA, Bottomly K. Lipopolysaccharide-enhanced, toll-like receptor 4-dependent T helper cell type 2 responses to inhaled antigen. J Exp Med. 2002; 196:1645–1651.
38. Daan de Boer J, Roelofs JJ, de Vos AF, de Beer R, Schouten M, Hommes TJ, et al. Lipopolysaccharide inhibits Th2 lung inflammation induced by house dust mite allergens in mice. Am J Respir Cell Mol Biol. 2013; 48:382–389.
39. O'Neil DA, Porter EM, Elewaut D, Anderson GM, Eckmann L, Ganz T, et al. Expression and regulation of the human beta-defensins hBD-1 and hBD-2 in intestinal epithelium. J Immunol. 1999; 163:6718–6724.
40. Gioannini TL, Teghanemt A, Zhang D, Coussens NP, Dockstader W, Ramaswamy S, et al. Isolation of an endotoxin-MD-2 complex that produces Toll-like receptor 4-dependent cell activation at picomolar concentrations. Proc Natl Acad Sci U S A. 2004; 101:4186–4191.
41. Zhang J, Kumar A, Wheater M, Yu FS. Lack of MD-2 expression in human corneal epithelial cells is an underlying mechanism of lipopolysaccharide (LPS) unresponsiveness. Immunol Cell Biol. 2009; 87:141–148.
42. Shimazu R, Akashi S, Ogata H, Nagai Y, Fukudome K, Miyake K, et al. MD-2, a molecule that confers lipopolysaccharide responsiveness on Toll-like receptor 4. J Exp Med. 1999; 189:1777–1782.
43. Liao EC, Hsieh CW, Chang CY, Yu SJ, Sheu ML, Wu SM, et al. Enhanced Allergic Inflammation of Der p 2 Affected by Polymorphisms of MD-2 Promoter. Allergy Asthma Immunol Res. 2015; 7:497–506.
44. Ohto U, Fukase K, Miyake K, Satow Y. Crystal structures of human MD-2 and its complex with antiendotoxic lipid IVa. Science. 2007; 316:1632–1634.
45. Derewenda U, Li J, Derewenda Z, Dauter Z, Mueller GA, Rule GS, et al. The crystal structure of a major dust mite allergen Der p 2, and its biological implications. J Mol Biol. 2002; 318:189–197.
46. Kim HM, Park BS, Kim JI, Kim SE, Lee J, Oh SC, et al. Crystal structure of the TLR4-MD-2 complex with bound endotoxin antagonist Eritoran. Cell. 2007; 130:906–917.
47. Trompette A, Divanovic S, Visintin A, Blanchard C, Hegde RS, Madan R, et al. Allergenicity resulting from functional mimicry of a Toll-like receptor complex protein. Nature. 2009; 457:585–588.
48. Yin SC, Liao EC, Chiu CL, Chang CY, Tsai JJ. Der p2 internalization by epithelium synergistically augments toll-like receptor-mediated proinflammatory signaling. Allergy Asthma Immunol Res. 2015; 7:393–403.
49. Ryu JH, Yoo JY, Kim MJ, Hwang SG, Ahn KC, Ryu JC, et al. Distinct TLR-mediated pathways regulate house dust mite-induced allergic disease in the upper and lower airways. J Allergy Clin Immunol. 2013; 131:549–561.
50. Lee CG, Da Silva CA, Dela Cruz CS, Ahangari F, Ma B, Kang MJ, et al. Role of chitin and chitinase/chitinase-like proteins in inflammation, tissue remodeling, and injury. Annu Rev Physiol. 2011; 73:479–501.
51. Reese TA, Liang HE, Tager AM, Luster AD, Van Rooijen N, Voehringer D, et al. Chitin induces accumulation in tissue of innate immune cells associated with allergy. Nature. 2007; 447:92–96.
52. Thomas WR, Smith WA, Hales BJ, Mills KL, O'Brien RM. Characterization and immunobiology of house dust mite allergens. Int Arch Allergy Immunol. 2002; 129:1–18.
53. Thomas WR, Hales BJ, Smith WA. House dust mite allergens in asthma and allergy. Trends Mol Med. 2010; 16:321–328.
54. Ichikawa S, Takai T, Yashiki T, Takahashi S, Okumura K, Ogawa H, et al. Lipopolysaccharide binding of the mite allergen Der f 2. Genes Cells. 2009; 14:1055–1065.
55. Braun-Fahrländer C, Riedler J, Herz U, Eder W, Waser M, Grize L, et al. Environmental exposure to endotoxin and its relation to asthma in school-age children. N Engl J Med. 2002; 347:869–877.
56. Gehring U, Bischof W, Fahlbusch B, Wichmann HE, Heinrich J. House dust endotoxin and allergic sensitization in children. Am J Respir Crit Care Med. 2002; 166:939–944.
57. Riedler J, Braun-Fahrländer C, Eder W, Schreuer M, Waser M, Maisch S, et al. Exposure to farming in early life and development of asthma and allergy: a cross-sectional survey. Lancet. 2001; 358:1129–1133.
58. Wills-Karp M, Santeliz J, Karp CL. The germless theory of allergic disease: revisiting the hygiene hypothesis. Nat Rev Immunol. 2001; 1:69–75.