Abstract
Purpose
Asthma exacerbation from human rhinovirus (HRV) infection is associated with deficient antiviral interferon (IFN) secretion. Although chronic rhinosinusitis (CRS), an inflammatory upper airway disease, is closely linked to asthma, IFN-β responses to HRV infections in human nasal epithelial cells (HNECs) from CRS patients remain to be studied. We evaluated inflammatory and antiviral responses to HRV infection in HNECs from CRS patients.
Methods
HNECs, isolated from turbinate tissue of 13 patients with CRS and 14 non-CRS controls, were infected with HRV16 for 4 hours. The HRV titer, LDH activity, production of proinflammatory cytokines and IFN-β proteins, and expression levels of RIG-I and MDA5 mRNA were assessed at 8, 24, and 48 hours after HRV16 infection.
Results
The reduction in viral titer was slightly delayed in the CRS group compared to the non-CRS control group. IL-6 and IL-8 were significantly increased to a similar extent in both groups after HRV infection. In the control group, IFN-β production and MDA5 mRNA expression were significantly increased at 8 and 24 hours after HRV16 infection, respectively. By contrast, in the CRS group, IFN-β was not induced by HRV infection; however, HRV-induced MDA5 mRNA expression was increased, but the increase was slightly delayed compared to the non-CRS control group. The RIG-I mRNA level was not significantly increased by HRV16 infection in either group.
Conclusions
HRV-induced secretion of proinflammatory cytokines in CRS patients was not different from that in the non-CRS controls. However, reductions in viral titer, IFN-β secretion, and MDA5 mRNA expression in response to HRV infection in CRS patients were slightly impaired compared to those in the controls, suggesting that HRV clearance in CRS patients might be slightly deficient.
The airway epithelium, which plays a central role in defense against respiratory viruses, regulates both innate and adaptive immunity. The innate immune responses of the epithelium control the invasion and replication of viruses, while the adaptive immune system acts to effectively eliminate viruses.1 Human rhinoviruses (HRVs), positive-sense single-stranded RNA (ssRNA) viruses belonging to the Picornaviridae family, are the most frequent pathogenic cause of upper respiratory tract infections (URIs) in adults and children.23 HRV infections complicate sinusitis and otitis media, exacerbate asthma, cystic fibrosis, and chronic obstructive pulmonary disease, and cause lower respiratory tract infections in neonates, elderly, and immunocompromised patients.4
In human bronchial epithelial cells (BECs), HRV is initially recognized through pattern recognition receptors, such as Toll-like receptors (TLRs) and RNA helicases, including retinoic acid inducible gene-I (RIG-I) and melanoma differentiation-associated gene 5 (MDA5).56 RIG-I and MDA5 detect double-stranded RNA (dsRNA) generated during the replication of RNA viruses and induce antiviral responses.3 RIG-I is required for type I interferon (IFN) production in response to paramyxovirus, influenza virus, and Japanese encephalitis virus, whereas MDA5 is critical not only for IFN-β and IFN-γ-induced protein-10 (IP-10) secretion, but also for interleukin (IL)-6 production in response to picornavirus.7 IFN-β is a central component of the early antiviral response in virus-infected cell, and associated with apoptotic responses to virus infections in antiviral defense.48 HRV-infected airway epithelial cells release cytokines and chemokines, including IL-6 and IL-8, which recruit inflammatory cells of the innate immune system.910
Chronic rhinosinusitis (CRS) is one of the most common chronic airway diseases.11 HRV has been detected in 21% of epithelial scraping samples from the inferior turbinate of CRS patients.12 It was also found to be the most prevalent respiratory virus in CRS patients and the only virus with a significantly different infection rate between CRS patients and controls.13 Thus, HRV infections may have significant implications for the mucosal inflammation of CRS.
The pathophysiological features of CRS are strongly associated with those of asthma.14 Some studies have demonstrated that atopic characteristics among asthmatic children may be a critical risk factor for acute wheezing attacks provoked by viral respiratory tract infections, especially HRV infections.1516 A profound increase in HRV release and impaired production of HRV-induced IFN-β protein have been observed in asthmatic BECs.17 In another study, asthmatic BECs showed a delayed and deficient HRV16-induced type I IFN response, which was associated with a hyperresponsive airway and a positive response to the skin prick test.18 These results indicate that increased susceptibility to HRV infections coupled with impaired antiviral IFN production in asthmatic patients may be responsible for asthma exacerbation by RV infection. However, the innate immune response to HRV in patients with CRS remains to be studied. We therefore investigated deficient proinflammatory cytokine secretion and IFN-β responses to HRV in patients with CRS.
The inferior turbinate mucosal tissues were harvested from 13 adult patients with CRS who underwent endoscopic sinus surgery and turbinoplasty for the treatment of CRS and chronic hypertrophic rhinitis between August 2011 and March 2012 at Asan Medical Center, Seoul, Korea. All CRS patients fulfilled the established diagnostic criteria for CRS, such as having 2 or more of the following signs and symptoms: mucopurulent nasal drainage, nasal obstruction, facial pressure-fullness, hyposmia for more than 12 weeks, and inflammation documented by nasal endoscopic and computed tomography findings.11 Patients with fungal sinusitis, odontogenic sinusitis, current immunotherapy, or cystic fibrosis were excluded from the CRS group. The 14 non-CRS controls were adult volunteers who underwent turbinoplasty for the treatment of chronic hypertrophic rhinitis. Controls suffered from nasal congestion lasting more than 12 weeks in association with enlarged inferior turbinates. The presence of sinusitis in the control group was ruled out based on the individual's history and the results of nasal endoscopy, plain sinus X-ray, and/or computed tomography (CT) imaging. The CRS group included 10 CRS patients with nasal polyps (CRSwNP) (73.9%) and 3 CRS patients without nasal polyps (CRSsNP) (26.1%).
All subjects had no history of asthma or viral URI and had not received intranasal or oral corticosteroids during the 4-week period before surgery. A positive result to the allergy test was found in 2 of the 13 CRS patients (15.4%) and in 4 of the 14 non-CRS controls (28.6%). This study was approved by the Institutional Review Board of Asan Medical Center, and all participants gave written informed consent.
Cell isolation and preparation of inferior turbinate mucosal tissue were performed as previously described.20 Briefly, the inferior turbinate mucosa tissues were minced into 2-3 mm3 pieces and incubated overnight in Dulbecco's Modified Eagle's Medium: Nutrient Mixture F-12K (DMEM: F12K; GIBCO, Grand Island, NY, USA) containing 0.1% (w/v) dispase (Godo Shusei, Tokyo, Japan). Fetal bovine serum (GIBCO) was added to the separated cells to inactivate dispase. The cells were isolated by centrifugation, washed with phosphate-buffered saline (PBS), and grown in airway epithelial growth medium (AEGM; PromoCell, Heidelberg, Germany), with medium replacement every other day. After reaching 80% to 90% confluence, human nasal epithelial cells (HNECs) were trypsinized with a 0.1% trypsin-ethylene diamine tetraacetic acid solution, washed with PBS, and seeded onto 12-well plates at a density of 2×105 cells/mL.
HRV16 was obtained from the American Type Culture Collection (ATCC, Manassas, VA) and viral stocks were generated by infection of susceptible cells (Ohio HeLa cells), as previously described.19 HNECs were infected with HRV16 at a 1:1 multiplicity of infection or exposed to PBS alone for 4 hours at 33℃, washed with PBS, and incubated at 33℃ in AEGM. Successful HRV16 infections in HRV16-infected cells were confirmed by the semi-nested reverse transcriptase-polymerase chain reaction (RT-PCR), as previously described.2021 Briefly, picornavirus RNA was reverse transcribed and amplified using primers OL26 (5'-GCACTTCTGTTTCCCC-3') and OL27 (5'-CGGACACCCAAAGTAG-3'). Subsequently, the 388-bp picornavirus PCR product was amplified using the primers OL26 and JWA-1b (5'-CATTCAGGGGCCGGAGGA-3'). The PCR products were detected by gel electrophoresis on 1.8% agarose containing 0.5 g/mL ethidium bromide in Tris-acetate-ethylene diamine tetraacetate buffer and visualized under UV light. Successful infection with HRV16 was confirmed by identifying of a 292-bp RV-specific RT-PCR band.
The virus titer in the treated cell supernatants was calculated with the tissue culture infection dose50 (TCID50). MRC-5 human fetal lung fibroblasts (ATCC) were treated with a serial 10-fold dilution of each viral supernatant from separate wells at 8, 24, and 48 hours after HRV infection, incubated at 33℃ in minimum essential medium (GIBCO) for 7 days, and checked daily. Lactate dehydrogenase (LDH) release was measured to determine cell lysis, an indicator of the degree of cell death caused by viral infection. LDH activity was measured using the LDH assay kit (BioVision, Mountain View, CA, USA) according to the manufacturer's protocol.
The secretion of IL-6, IL-8, and IFN-β into culture supernatants was quantified using enzyme-linked immunosorbent assay kits for IL-6 and IL-8 (R&D Systems, Minneapolis, MN, USA), and IFN-β (PBL Interferon Source, Piscataway, NJ, USA), according to the manufacturers' protocols. The protein levels were measured at 8, 24, and 48 hours after HRV16 infection in HNECs from patients with CRS and control subjects.
Total RNA was extracted from cells with TRIzol (Invitrogen, Gaithersburg, MD, USA) and qualified and quantified with a spectrophotometer (NanoDrop® ND-1000; NanoDrop Technologies, Wilmington, DE, USA). cDNA was synthesized from 500 ng total RNA with the RevertAid™ First Strand cDNA synthesis kit (Fermentas, Vilnius, Lithuania), according to the manufacturer's protocol. Real-time PCR was performed with a LightCycler® 480 (Roche Diagnostics, Rotkreuz, Switzerland); each reaction mixture consisted of 0.5 µL RNA and 0.5 µL of 10 pmol forward and reverse primers for RIG-I and MDA5 (Table). The results were normalized to the β-actin level.
Statistical analysis was performed using the SPSS statistical software package (version 12.0; SPSS, Chicago, IL, USA). Data is expressed as the mean±standard deviation. Differences between the groups were analyzed with a Mann-Whitney test or a Wilcoxon signed-rank test. A P value of less than 0.05 was considered statistically significant.
In HNECs from control subjects, the viral titers in the culture supernatant at 8, 24, and 48 hours after HRV16 infection were 3.95±0.58, 3.31±0.64, and 2.54±0.10 logTCID50/mL, respectively, demonstrating a decrease in viral titers over time (P=0.011 from 8 to 24 hours and P=0.007 from 24 to 48 hours; Fig. 1A). By contrast, the viral titers in the culture supernatant from patients with CRS did not significantly change between 8 (3.77±0.58) and 24 hours (3.70±0.71) (P=0.553), but decreased between 24 and 48 hours (2.72±0.28) (P=0.007; Fig. 1A). However, there were no significant overall differences in the HRV16 titers between control subjects and patients with CRS at each sampling time point (P>0.05 for each).
LDH activities were significantly higher at 8 and 24 hours after HRV16 infection in the control group, whereas LDH activities were significantly higher at 48 hours after HRV16 infection in the CRS group (Fig. 1B). There were no differences in LDH activity between the control and CRS groups at each sampling time point (P>0.05 for each).
IL-6 and IL-8 release was significantly induced in both CRS patients and controls at 8, 24, and 48 hours after HRV16 infection (Fig. 2A and B). There were no differences in IL-6 secretion at 8 and 24 hours after HRV16 infection between the 2 groups. The IL-6 levels were significantly higher from CRS patients in HRV16-infected HNECs than from controls at 48 hours after infection (P=0.028; Fig. 2A). However, IL-8 levels were significantly lower from CRS patients in HRV16-infected HNECs than from controls at 8 hours after infection (P=0.028; Fig. 2B). Otherwise there were no significant differences in the IL-8 levels at the different time points between HNECs from HRV16-infected CRS patients and those from controls (Fig. 2B).
IFN-β protein was induced in HNECs from controls at 24 hours after HRV16 infection (P=0.028). On the other hand, there was no significant increase in the IFN-β protein level in HNECs from patients with CRS at 48 hours (Fig. 3).
The basal mRNA expression levels of RIG-I and MDA5 were measured in unstimulated HNECs at 8 hours. No significant differences were found in mRNA expression levels of RIG-I (P=0.766) and MDA5 (P=0.710) between uninfected HNECs from controls and those from CRS patients.
In each group, RIG-I and MDA5 mRNA expression levels were compared between the PBS and HRV subgroups (Fig. 4). RIG-I mRNA levels did not increase significantly following HRV16 infection, either in controls or in patients with CRS, at each sampling time point (Fig. 4A). MDA5 mRNA expression was higher in control HNECs at 8 hours after HRV16 infection (from 3.28±1.21×10-4 to 5.19±1.58×10-4, P=0.037; Fig. 4B). In patients with CRS, MDA5 mRNA expression was significantly higher at 24 hours after HRV16 infection (from 1.76±0.37×10-4 to 2.79±0.76×10-4, P=0.028; Fig. 4B).
CRS, a significant health problem with a large socioeconomic burden, causes considerable physical symptoms and markedly impacts quality of life.2223 Clinically, respiratory viral infections have been implicated in the exacerbation of CRS. However, innate immune responses to HRV infection, the most common cause of URI, in CRS patients have not been well studied. Here, we report slightly slower viral clearance and milder impairment of IFN-β production after HRV infections in HNECs from patients with CRS than in those from non-CRS controls. This is the first report to show that antiviral responses to HRV infections in patients with CRS are slightly deficient.
In our previous study, HRV16 infection stimulated IL-6 and IL-8 production in an organ culture model after 48 hours. However, no significant differences in the production of these cytokines or in the infection rate of HRV16 were observed between turbinate tissue from patients with CRS with nasal polyps and those from normal subjects.20 In our present study, although HRV induced higher IL-6 levels at 48 hours after HRV infection in HNECs from CRS patients and lower IL-8 levels at 8 hours than in non-CRS controls, there were no significant overall differences in HRV16-induced IL-6 and IL-8 protein levels between CRS patients and controls. These results are in agreement with those of our previous study,20 although the present study was conducted with a primary cell culture model that additionally assessed antiviral responses to HRV16 infection. Our results suggest that HNECs from patients with CRS and control subjects do not differ considerably in terms of proinflammatory cytokine secretion. This may be because HNECs are less responsive to HRV infection than human BECs.24
Intracellular viral RNA sensors, including RIG-I and MDA5, play a key role in HRV16 recognition and virus-induced IFN responses in human BECs,356725 and pattern recognition receptors, such as TLR3, TLR7, TLR9, RIG-1, and MDA-5, have been shown to be expressed in the epithelium of normal inferior turbinate.26 Moreover, RIG-1 and MDA-5 were reported to be expressed in nasal polyp tissue.27 In response to HRV infection, MDA5 recognizes the dsRNA produced upon viral replication and mainly mediates IFN-β release.526 RIG-I and MDA5 are also needed for maximal HRV-induced proinflammatory cytokine secretion and inhibition of these receptors increases HRV replication.6 Deficient HRV-induced IFN-β production has been reported in BECs from patients with asthma,171829 chronic obstructive pulmonary disease,30 and cystic fibrosis,31 and is related to chronic airway inflammation.17 IFN-β has a strong, long-lasting, and dose-dependent protective effect against HRV infection in BECs.32 However, little is known about the induction of RIG-I and MDA5 and the production of IFN-β after HRV16 infection in HNECs from CRS patients. In the present study, IFN-β protein levels and basal and HRV-induced RIG-I and MDA5 mRNA levels were measured 8, 24, and 48 hours after HRV16 infection in HNECs from patients with CRS and controls. In HNECs from patients with CRS, we found that IFN-β protein induction was impaired and that MDA5 mRNA expression was slightly delayed after HRV infection, suggesting that this may have been responsible for the slight delay in HRV clearance. However, there was no clear-cut difference between anti-HRV16 responses in HNECs from CRS patients and control subjects. This may be because the IFN-β response was very weak in both groups; IFN-β protein was only increased at 24 hours after HRV infection in HNECs from control subjects and remained unchanged at 8 and 48 hours. The weak IFN-β response to HRV in HNECs is in line with the result of other studies showing that IFN-β expression does not contribute to the main first-line defense against respiratory virus infection in HNECs and that IFN-β is not detectable in nasopharyngeal washes in infants with respiratory syncytial virus infection.3334 RIG-I and MDA5 were induced at 8-12 hours after HRV infection in BECs, but they were not constitutively expressed.6 In the present study, RIG-I was not significantly induced by HRV16 infection in either group. This result is in agreement with the finding that RIG-I is not important for IFN production in response to picornavirus.7 HNECs from CRS patients showed a similar basal level and only a slightly delayed induction of MDA5 mRNA expression compared to HNECs from control subjects. We hypothesized that HRV-induced aggravation of CRS may be attributable to a defect in the immune response to HRV infection in HNECs from CRS patients, as previously observed in BECs from asthmatics. However, our results showing that HNECs from CRS patients and non-CRS patients had similar proinflammatory cytokine secretion after HRV infection and that the antiviral responses in HNECs from CRS patients were only slightly impaired does not clearly support this hypothesis. Our results suggest that HNECs from CRS patients use different responses to HRV infection to those of BECs from asthmatic patients.
The present study has some limitations. We used the inferior turbinate tissue, but not the ethmoid sinus mucosa, from CRS and control subjects, although the ethmoid sinus mucosa may be more prone to HRV infection than the inferior turbinate mucosa. However, we were forced to employ the inferior turbinate tissue because the experiments required large amounts of nasal epithelial cells. It was practically impossible to obtain healthy sinus epithelium for the experiments. Inferior turbinate tissues from patients undergoing septoplasty have also been used as control tissue in several studies.3536 We analyzed the HRV-induced immune response of CRS patients regardless of their NP status because the proportion of patients with CRSsNP was small. Therefore, our results cannot be used to infer differences in immune responses to HRV infection between CRSwNP and CRSsNP patients. Also, we did not use differentiated HNECs cultured at an air-liquid interface; thus, the results may not completely reflect the full response to HRV infections in human nasal epithelium. Although the air-liquid interface is generally a better culture system for the investigation of inflammatory responses after HRV infections in HNECs, we have obtained results similar to those of other studies using a submerged in vitro system for HNEC cultivation.1020373839 HRV16-induced cell viability and apoptosis, and the level of intracellular viral RNA in HRV16-infected HNECs could not be assessed due to the restriction in the number of cell passages permitted by this culture system and the limited amount of cellular RNA that can be obtained from this system. Pattern recognition receptor-mediated signaling involves a complex pattern of protein-protein interactions and modifications, including phosphorylation and ubiquitination.17 However, as we did not examine for changes in protein interactions and modifications, we cannot exclude the possibility that HRV16 infections in HNECs involve such changes.
We found that HNECs from CRS patients showed slightly impaired responses to HRV infection, such as a slightly delayed clearance of HRV, a mild impairment of IFN-β protein production, and a slight delay in MDA5 mRNA expression. Unlike BECs from asthmatics, it was difficult to conclude that anti-HRV16 responses in HNECs from CRS patients are markedly impaired compared to those in HNECs from non-CRS controls. The results indicate that HNECs from CRS patients may respond differently to HRV infection than the lower airway epithelial cells of asthmatic patients.
Figures and Tables
Fig. 1
HRV16 viral titer and LDH activity in HNECs from controls and patients with CRS. (A) HRV16 release into the culture supernatant was determined by calculating the logTCID50/mL using a titration assay. The reduction in the HRV16 titer was slightly slower in the CRS group than in the controls, although there were no significant differences in the HRV16 titers between control subjects and patients with CRS at 8, 24, and 48 hours after HRV16 infection. †P<0.05, ††P<0.01. (B) LDH release from HNECs into the culture supernatant was measured. The increase in LDH activity was also slightly slower in the CRS group than in the controls, although there were no significant differences in LDH activity between the control group and the CRS group at 8, 24, and 48 hours after A HRV16 infection. *P<0.05; **P<0.01.
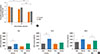
Fig. 2
Secretion of proinflammatory cytokines from HNECs into the culture supernatant. Levels of IL-6 (A) and IL-8 (B) at 8, 24, and 48 hours. IL-6 and IL-8 secretion were significantly increased to a similar extent in both CRS patients and controls after HRV16 infection, except for IL-6 levels at 48 hours and IL-8 levels at 8 hours. *P<0.05; **P<0.01; §P<0.05, control group compared with the CRS group.
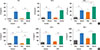
Fig. 3
HRV16-induced IFN-β protein in HNECs. IFN-β was induced at 24 hours after HRV16 infection in the non-CRS control group. However, IFN-β expression was not significantly increased by HRV16 infection in the CRS groups. *P<0.05.
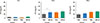
Fig. 4
RNA helicase mRNA expression in HNECs after HRV16 infection. (A) RIG-I mRNA was not significantly increased by HRV16 infection in either group. (B) MDA5 mRNA expression was significantly increased at 8 hours after HRV16 infection in the control group and at 24 hours in the CRS group. *P<0.05.
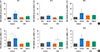
ACKNOWLEDGMENTS
This study was supported by grants from the Korean Healthy Technology R&D Project, Ministry of Health & Welfare, Republic of Korea (A110893). The funders had no role in study design, data collection and analysis, decision to publish, or preparation of the manuscript.
References
1. Vareille M, Kieninger E, Edwards MR, Regamey N. The airway epithelium: soldier in the fight against respiratory viruses. Clin Microbiol Rev. 2011; 24:210–229.
2. Winther B, Gwaltney JM Jr, Mygind N, Hendley JO. Viral-induced rhinitis. Am J Rhinol. 1998; 12:17–20.
3. Greenberg SB. Respiratory viral infections in adults. Curr Opin Pulm Med. 2002; 8:201–208.
4. Greenberg SB. Respiratory consequences of rhinovirus infection. Arch Intern Med. 2003; 163:278–284.
5. Triantafilou K, Vakakis E, Richer EA, Evans GL, Villiers JP, Triantafilou M. Human rhinovirus recognition in non-immune cells is mediated by Toll-like receptors and MDA-5, which trigger a synergetic pro-inflammatory immune response. Virulence. 2011; 2:22–29.
6. Slater L, Bartlett NW, Haas JJ, Zhu J, Message SD, Walton RP, et al. Coordinated role of TLR3, RIG-I and MDA5 in the innate response to rhinovirus in bronchial epithelium. PLoS Pathog. 2010; 6:e1001178.
7. Kato H, Takeuchi O, Sato S, Yoneyama M, Yamamoto M, Matsui K, et al. Differential roles of MDA5 and RIG-I helicases in the recognition of RNA viruses. Nature. 2006; 441:101–105.
8. Takaoka A, Hayakawa S, Yanai H, Stoiber D, Negishi H, Kikuchi H, et al. Integration of interferon-alpha/beta signalling to p53 responses in tumour suppression and antiviral defence. Nature. 2003; 424:516–523.
9. Message SD, Johnston SL. Host defense function of the airway epithelium in health and disease: clinical background. J Leukoc Biol. 2004; 75:5–17.
10. Yeo NK, Hwang YJ, Kim ST, Kwon HJ, Jang YJ. Asian sand dust enhances rhinovirus-induced cytokine secretion and viral replication in human nasal epithelial cells. Inhal Toxicol. 2010; 22:1038–1045.
11. Rosenfeld RM, Andes D, Bhattacharyya N, Cheung D, Eisenberg S, Ganiats TG, et al. Clinical practice guideline: adult sinusitis. Otolaryngol Head Neck Surg. 2007; 137:S1–S31.
12. Jang YJ, Kwon HJ, Park HW, Lee BJ. Detection of rhinovirus in turbinate epithelial cells of chronic sinusitis. Am J Rhinol. 2006; 20:634–636.
13. Cho GS, Moon BJ, Lee BJ, Gong CH, Kim NH, Kim YS, et al. High rates of detection of respiratory viruses in the nasal washes and mucosae of patients with chronic rhinosinusitis. J Clin Microbiol. 2013; 51:979–984.
14. Hellings PW, Hens G. Rhinosinusitis and the lower airways. Immunol Allergy Clin North Am. 2009; 29:733–740.
15. Heymann PW, Carper HT, Murphy DD, Platts-Mills TA, Patrie J, McLaughlin AP, et al. Viral infections in relation to age, atopy, and season of admission among children hospitalized for wheezing. J Allergy Clin Immunol. 2004; 114:239–247.
16. Soto-Quiros M, Avila L, Platts-Mills TA, Hunt JF, Erdman DD, Carper H, et al. High titers of IgE antibody to dust mite allergen and risk for wheezing among asthmatic children infected with rhinovirus. J Allergy Clin Immunol. 2012; 129:1499–1505.e5.
17. Wark PA, Johnston SL, Bucchieri F, Powell R, Puddicombe S, Laza-Stanca V, et al. Asthmatic bronchial epithelial cells have a deficient innate immune response to infection with rhinovirus. J Exp Med. 2005; 201:937–947.
18. Sykes A, Edwards MR, Macintyre J, del Rosario A, Bakhsoliani E, Trujillo-Torralbo MB, et al. Rhinovirus 16-induced IFN-α and IFN-β are deficient in bronchoalveolar lavage cells in asthmatic patients. J Allergy Clin Immunol. 2012; 129:1506–1514.e6.
19. Jang YJ, Wang JH, Kim JS, Kwon HJ, Yeo NK, Lee BJ. Levocetirizine inhibits rhinovirus-induced ICAM-1 and cytokine expression and viral replication in airway epithelial cells. Antiviral Res. 2009; 81:226–233.
20. Wang JH, Kwon HJ, Chung YS, Lee BJ, Jang YJ. Infection rate and virus-induced cytokine secretion in experimental rhinovirus infection in mucosal organ culture: comparison between specimens from patients with chronic rhinosinusitis with nasal polyps and those from normal subjects. Arch Otolaryngol Head Neck Surg. 2008; 134:424–427.
21. Kim JH, Kwon HJ, Jang YJ. Effects of rhinovirus infection on the expression and function of cystic fibrosis transmembrane conductance regulator and epithelial sodium channel in human nasal mucosa. Ann Allergy Asthma Immunol. 2012; 108:182–187.
22. Meltzer EO, Hamilos DL, Hadley JA, Lanza DC, Marple BF, Nicklas RA, et al. Rhinosinusitis: establishing definitions for clinical research and patient care. J Allergy Clin Immunol. 2004; 114:155–212.
23. Fokkens WJ, Lund VJ, Mullol J, Bachert C, Alobid I, Baroody F, et al. EPOS 2012: European position paper on rhinosinusitis and nasal polyps 2012. A summary for otorhinolaryngologists. Rhinology. 2012; 50:1–12.
24. Lopez-Souza N, Favoreto S, Wong H, Ward T, Yagi S, Schnurr D, et al. In vitro susceptibility to rhinovirus infection is greater for bronchial than for nasal airway epithelial cells in human subjects. J Allergy Clin Immunol. 2009; 123:1384–1390.e2.
25. Wang Q, Nagarkar DR, Bowman ER, Schneider D, Gosangi B, Lei J, et al. Role of double-stranded RNA pattern recognition receptors in rhinovirus-induced airway epithelial cell responses. J Immunol. 2009; 183:6989–6997.
26. Proud D, Hudy MH, Wiehler S, Zaheer RS, Amin MA, Pelikan JB, et al. Cigarette smoke modulates expression of human rhinovirus-induced airway epithelial host defense genes. PLoS One. 2012; 7:e40762.
27. Bogefors J, Kvarnhammar AM, Latif L, Petterson T, Uddman R, Cardell LO. Retinoic acid-inducible gene 1-like receptors in the upper respiratory tract. Am J Rhinol Allergy. 2011; 25:e262–e267.
28. Takeuchi O, Akira S. MDA5/RIG-I and virus recognition. Curr Opin Immunol. 2008; 20:17–22.
29. Edwards MR, Regamey N, Vareille M, Kieninger E, Gupta A, Shoemark A, et al. Impaired innate interferon induction in severe therapy resistant atopic asthmatic children. Mucosal Immunol. 2013; 6:797–806.
30. Mallia P, Message SD, Gielen V, Contoli M, Gray K, Kebadze T, et al. Experimental rhinovirus infection as a human model of chronic obstructive pulmonary disease exacerbation. Am J Respir Crit Care Med. 2011; 183:734–742.
31. Vareille M, Kieninger E, Alves MP, Kopf BS, Möller A, Geiser T, et al. Impaired type I and type III interferon induction and rhinovirus control in human cystic fibrosis airway epithelial cells. Thorax. 2012; 67:517–525.
32. Gaajetaan GR, Geelen TH, Vernooy JH, Dentener MA, Reynaert NL, Rohde GG, et al. Interferon-β induces a long-lasting antiviral state in human respiratory epithelial cells. J Infect. 2013; 66:163–169.
33. Okabayashi T, Kojima T, Masaki T, Yokota S, Imaizumi T, Tsutsumi H, et al. Type-III interferon, not type-I, is the predominant interferon induced by respiratory viruses in nasal epithelial cells. Virus Res. 2011; 160:360–366.
34. Scagnolari C, Midulla F, Pierangeli A, Moretti C, Bonci E, Berardi R, et al. Gene expression of nucleic acid-sensing pattern recognition receptors in children hospitalized for respiratory syncytial virus-associated acute bronchiolitis. Clin Vaccine Immunol. 2009; 16:816–823.
35. Luo B, Feng L, Jintao D, Yafeng L, Shixi L, Nan Z, et al. Immunopathology features of chronic rhinosinusitis in high-altitude dwelling Tibetans. Allergy Rhinol (Providence). 2013; 4:e69–e76.
36. Shi LL, Xiong P, Zhang L, Cao PP, Liao B, Lu X, et al. Features of airway remodeling in different types of Chinese chronic rhinosinusitis are associated with inflammation patterns. Allergy. 2013; 68:101–109.
37. Yeo NK, Jang YJ. Rhinovirus infection-induced alteration of tight junction and adherens junction components in human nasal epithelial cells. Laryngoscope. 2010; 120:346–352.
38. Jang YJ, Lee YH, Shin SH. Rhinovirus-infected nasal polyp epithelial cells: effect on the activation and migration of eosinophils by airborne fungi. Ann Allergy Asthma Immunol. 2010; 104:434–439.
39. Min JY, Shin SH, Kwon HJ, Jang YJ. Levocetirizine inhibits rhinovirus-induced bacterial adhesion to nasal epithelial cells through down-regulation of cell adhesion molecules. Ann Allergy Asthma Immunol. 2012; 108:44–48.