Abstract
Purpose
Allergic rhinitis (AR) is an inflammatory disorder of the upper airway. Exosomes or extracellular vesicles are nanosized vesicles of endosomal origin released from inflammatory and epithelial cells that have been implicated in allergic diseases. In this study, we characterized the microRNA (miRNA) content of exosomes in AR.
Methods
Extracellular vesicles were isolated from nasal mucus from healthy control subjects (n=10) and patients with severe AR (n=10). Vesicle RNA was analyzed by using a TaqMan microRNA assays Human Panel-Early Access kit (Applied Biosystems, Foster City, CA, USA) containing probes for 366 human miRNAs, and selected findings were validated with quantitative RT-PCR. Target prediction and pathway analysis for the differentially expressed miRNAs were performed using DIANA-mirPath.
Results
Twenty-one vesicle miRNAs were up-regulated and 14 miRNAs were under-regulated significantly (P<0.05) in nasal mucus from AR patients when compared to healthy controls. Bioinformatic analysis by DIANA-mirPath demonstrated that 32 KEGG biological processes were significantly enriched (P<0.05, FDR corrected) among differentially expressed vesicle miRNA signatures. Among them, the B-cell receptor signaling pathway (P=3.709E-09), the natural killer cell-mediated cytotoxicity (P=8.466E-05), the T-cell receptor signaling pathway (P=0.00075), the RIG-I-like receptor signaling pathway (P=0.00127), the Wnt signaling pathway (P=0.00130), endocytosis (P=0.00440), and salivary secretion (P=0.04660) were the most prominent pathways enriched in quantiles with differential vesicle miRNA patterns. Furthermore, miR-30-5p, miR-199b-3p, miR-874, miR-28-3p, miR-203, and miR-875-5p, involved in B-cell receptor and salivary secretion signaling pathways, were selected for validation using independent samples from 44 AR patients and 20 healthy controls. MiR-30-5p and miR-199b-3p were significantly increased in extracellular vesicles from nasal mucus when compared to healthy controls, while miR-874 and miR-28-3p were significantly down-regulated. In addition, miRNA-203 was significantly increased in AR patients, while miRNA-875-5p was found to be significantly decreased in AR patients.
Allergic rhinitis (AR) is an IgE-mediated disorder triggered by exposure of nasal mucosa to allergens.1 In a worldwide study of over 1 million adolescents aged 13 and 14 years, the prevalence of AR was 14.6%.2 AR results in rhinorrhea, itching, and sneezing as well as sleep disturbance, which are easily observed and reported by parents or guardians.3 However, nasal congestion, the most common symptom of AR, may be more difficult to elicit from young children.23 A main goal of AR research is to understand genetic and environmental triggers for AR and factors that lead to variations in its natural history. Identifying pathways that allow AR to be expressed is fundamental to improving preventative strategies, developing diagnostic tools, and designing therapies.
Exosomes are present in most body fluids, and their composition differs depending on their cellular origin.456 Vesicles in the extracellular space are derived from exosomes and also from ectosomes (shedding vesicles). The presence of RNA has previously been confirmed in exosomes from saliva, plasma, and breast milk.7 Exosomes can transfer genetic material to nearby cells, thereby affecting the function of the recipient cell.789 However, importance of exsomes in the regulation of AR remains to be established. MicroRNAs (miRNAs) are small (22-25 nt) endogenous noncoding RNAs that anneal to the 3'-UTR of target mRNAs to mediate inhibition of translation and lower protein levels. The presence of microRNA (miRNA) in exosomes from certain bodily fluids, including saliva, has also been confirmed.910 MicroRNAs have emerged as key negative regulators of diverse biological and pathological processes, including developmental timing, organogenesis, apoptosis, cell proliferation, differentiation,11 and tumorigenesis.1213 Previous reports implicated miRNAs in the development of AR.1415 However, alterations in vesicle miRNA content in AR have not yet been described. The primary goal of this study was to characterize differences in vesicle miRNA in nasal mucus from AR patients and to explore their biological functions.
The study was performed in accordance with relevant guidelines and regulations, following the approval of the licensing committee of First People Hospital of Zhangjiagang City. Written informed consent was obtained from patients with AR or their parents in the case of children. Written informed consent for participation in the study was obtained from all the healthy controls in the study. This work received approval from the institution ethics committee and conformed to the tenets of the Declaration of Helsinki.
Patients presenting with AR visited Department of Otolaryngology, First People Hospital of Zhangjiagang City, Suzhou, China from 2010 to 2014. The study population comprised 54 patients with clinical data on persistent AR and 30 controls. A detailed evaluation was conducted, including clinical history and physical examination, in patients and healthy controls. The diagnosis of AR was based on the patients' medical history, symptoms, and the presence of a positive skin prick test (SPT, Allergopharma, Hamburg, Germany) in response to a panel of common allergens defined by the ARIA 2008 guidelines.1 The SPT results were utilized to diagnose AR in accordance with the recommendations of the Subcommittee on Allergen Standardization and Skin Tests of the European Academy of Allergy and Clinical Immunology.16 A positive SPT result was defined as the formation of a wheal larger than or equal to a half the diameter of the histamine control wheal, and at least 3 mm larger than the diameter of the negative control wheal. A total of 18 inhaled allergens were tested, including house dust, grass, tree, mold, food, and cat/dog dander. Exclusion criteria were: bronchial asthma, chronic rhinosinusitis, nasal polyposis, excessive septal deviation, and current smoking. The patients were not under pharmacological treatment (anti-histamines) at least 10 days before testing. Exclusion criteria for the healthy subjects were clinical history of rhinitis and positive reaction to any of the allergens from the test panel. All the patients and controls underwent a bronchial provocation test. Airway responsiveness to methacholine was evaluated, with FEV1 higher than 70% of predicted.
Nasal mucus samples were collected according to the method of Ruocco et al.,17 which is well tolerated by subjects and induces minimal stimulation of the mucosa. The nasal mucus was collected in patients with ongoing nasal symptoms and in the control group. In AR patients, the pieces of gauzes imbibed with nasal mucus were then placed in a plastic tip and put into a test tube in which 1.000 µL of PBS at pH 7.4 were added. The mucus was eluted by centrifugation at 2,000 rpm for 20 minutes at 4℃. The mucus samples were then collected in plastic tubes and stored at -20℃ until tested for extracellular vesicle isolation.
Extracellular vesicles were isolated as described before.18 In brief, nasal mucus was subjected to successive centrifugations of 3,000×g (15 minutes) and 10,000×g (30 minutes). Extracellular vesicles were then pelleted at 50,000×g for 1 hour, using an SW28 rotor (Beckman Coulter Instruments, Brea, CA, USA). Extracellular vesicles pellets were resuspended in 0.32 M sucrose and centrifuged at 100,000×g for 1 hour (SW60Ti rotor; Beckman Coulter Instruments). The extracellular vesicles pellet was then resuspended in PBS.
Nasal mucus was added directly to Dynabeads (2 mL/mL beads) coated with anti-MHC class II (MHCII) antibodies (clone HKB1; Invitrogen/Dynal, Paisley, United Kingdom) as previously described.19 Beads were labeled with fluorescein isothiocyanate-labeled anti-MHC class II, CD63, or isotype-matched controls (BioLegend, San Diego, CA, USA). Samples were analyzed in a FACS Calibur (BD Biosciences, San Jose, CA, USA) by using forward scatter/side scatter bead gating, and mean fluorescence intensity (MFI) ratios were calculated as the geometric mean of the marker divided by the geometric mean of the isotype control.
RNA was extracted and separated into small RNA (including miRNAs, 18-200 nt) and large RNA (>200 nt) fractions by using a mirVana miRNA isolation kit (Applied Biosystems, Foster City, CA, USA). RNA quality was assessed by using UV 260/280 and 230/260 absorbance ratios obtained by using Nanodrop (Thermo Scientific, Wilmington, NC, USA), resulting in a mean 260/280 ratio of 1.95. RNA size distribution was examined on RNA Pico LabChips (Agilent Technologies, Palo Alto, CA, USA) processed on the Agilent 2100 Bioanalyzer small RNA electrophoresis program.
TaqMan microRNA assays Human Panel-Early Access kit (Applied Biosystems) containing probes for 366 human miRNAs was utilized for vesicle miRNA profiling. RNA extracted from nasal mucus among AR patients and healthy controls were separately pooled and used for miRNA profiling. Equal quantity of RNA (30 ng) from nasal mucus (n=10) from both groups were pooled and reverse transcribed for cDNA synthesis using the TaqMan Multiplex RT set (Applied Biosystems) for TaqMan Array Human MicroRNA Panels. Each RT reaction was diluted 62.5-fold with water, and 55 µL of each diluted product was combined with 55 µL of TaqMan 2X Universal PCR Master Mix, No AmpErase UNG (Applied Biosystems). One hundred microliters of the sample/master mix for each Multiplex pool were loaded into fill reservoirs on the microfluidic card. The array was then centrifuged and mechanically sealed with the Applied Biosystems sealer device. Quantitative PCR was performed on an Applied BioSystems 7900HT thermocycler (Applied Biosystems) using the manufacturer's recommended cycling conditions. The relative expression levels between samples were calculated using the comparative delta Ct (threshold cycle number) method.20
DIANA-mirPath,21 a web-based application, was introduced to perform the enrichment analysis of predicted target genes by 1 or more miRNAs in biological pathways. Two algorithms were used to predict miRNA targets, namely, microT-CDS2223 and miRTarBase.24 The software performs enrichment analysis of multiple miRNA target genes to all known KEGG pathways. The combinatorial effect of co-expressed miRNAs in the modulation of a given pathway is taken into account by the simultaneous analysis of multiple miRNAs. The graphical output of the program provides an overview of parts of the pathway modulated by selected miRNAs, facilitating the interpretation and presentation of the analysis results. The statistical significance value associated with the identified biological pathways was calculated by the mirPath. The software is available at http://microrna.gr/mirpath.
The independent sets of extracellular vesicles samples from nasal mucus were used for qPCR confirmation. Total RNA was isolated as described earlier. Six differential miRNAs (miR-30-5p, miR-199b-3p, miR-874, miR-28-3p, miR-203, and miR-875-5p) from miRNA array were further quantitated by TaqMan miRNA assays (Applied Biosystems). The delta Ct values were calculated by using RNU44 as the endogenous control.
Data analysis of miRNA expression levels from TaqMan Low-Density Array was performed by using the SDS software version 2.2.2 (Applied Biosystems) and the baseline and threshold were automatically set. Data were normalized and then analyzed to define vesicle miRNAs that were differentially expressed between AR patients' pools and healthy controls. Assays that had Ct values >35 were removed from the analysis. Student's t test was performed to detect differentially expressed miRNAs between AR patients and healthy controls. The Mann-Whitney test was performed to determine the significance of vesicle miRNA levels from nasal mucus for independent validation. All statistical calculations of independent TaqMan real-time PCR were performed using GraphPad Prism 5. All data were expressed as mean±SE unless otherwise specified. P values of less than 0.05 were considered statistically significant.
The demographics of the cases and controls enrolled in this study are shown in Table 1. There were no significant differences between the cases and controls in terms of the mean age and gender distribution. Twenty patients (37%) were sensitive to house dust mite, 10 (18.5%) were sensitive to tree pollen, and 18 (33.3%) were sensitive to multiple allergens. Pollens were seasonal allergens. House dust mite and multiple allergens, such as grass, tree, mold, food, cat/dog dander, were perennial allergens. All the subjects included in the miRNA array were perennial-type patients.
To confirm that the structures studied indeed are exosomal phenotyped extracellular vesicles, they were examined by flow cytometric analysis. Nasal mucus was treated with Dynabeads to detect surface proteins. Pilot experiments showed that the studied vesicles were confirmed as exosomes by using FACS analysis by specifically binding to latex beads coated with anti-CD63 (Fig. 1), which demonstrated the presence of the surface protein CD63-a commonly used marker of exosomes. Further analysis indicated that exosomes from all samples showed the presence of MHCII (Fig. 1). No significant differences were seen between the groups.
In an initial effort to identify differentially expressed vesicle miRNA in AR patients, we profiled the expression of 366 miRNA by using TaqMan miRNA arrays. The high sensitivity and specificity of this method have been well established.25 To investigate relative abundances of the vesicle miRNAs detected, they were normalized in each sample to Mamm RNU44. The data indicated that 79 miRNAs (21.3%) could be detected (assays giving Ct values <35 in at least 1 pool were classed as detectable, results not shown). The study revealed differential expression of 35 vesicle miRNAs in nasal mucus extracellular vesicles. Among them, we found that 21 vesicle miRNAs were up-regulated and 14 miRNAs were under-regulated significantly (P<0.05) in nasal mucus from AR patients when compared to healthy controls (Table 2). To investigate relative abundances of the miRNAs detected, we found strong correlation between our Taqman miRNA arrays profiling and Taqman Real-Time RT-PCR data (Pearson correlation coefficient: r=0.95, P<0.001; Fig. 2). Taken together, these data indicated that our preliminary screening assay of aberrantly expressed miRNAs for AR in extracellular vesicle was a reliable and feasible method.
In order to examine which biologic pathways were affected during development of AR, we utilized DIANA-mirPath on AR-related dysregulated exosmal miRNA signatures for further investigation. Twenty-two KEGG biological processes were significantly enriched (P<0.05, FDR corrected) among differentially expressed vesicle miRNAs in nasal mucus when compared to those of healthy controls. Among them, the B-cell receptor signaling pathway (P=3.709E-09), Natural killer cell-mediated cytotoxicity (P=8.466E-05), the T-cell receptor signaling pathway (P=0.00075), the RIG-I-like receptor signaling pathway (P=0.00127), the Wnt signaling pathway (P=0.00130), endocytosis (P=0.00440), salivary secretion (P=0.04660) were the most prominent pathways enriched in quantiles with differential vesicle miRNA patterns (Table 3), suggesting that these biologic pathways were involved in the development of AR.
We employed TaqMan Real-Time RT-PCR to validate expression levels of the dysregulated miRNAs from microRNA assay. Four miRNAs (miR-30-5p, miR-199b-3p, miR-874, and miR-28-3p) in the B-cell receptor signaling pathway were selected for further validation using an independent cohort of 44 AR patients and 20 healthy controls. In agreement with the preliminary data from microRNA assay, miR-30-5p and miR-199b-3p were significantly increased in individual vesicle samples from nasal mucus when compared to healthy controls. While miR-874 and miR-28-3p were significantly under expressed in individual vesicle samples (Fig. 3). In addition, we chose another 2 miRNAs (miR-203 and miR-875-5p) in the salivary secretion pathway using individual vesicle samples from 44 AR patients and 20 healthy controls. MiRNA-203 was significantly increased in AR patients, while miRNA-875-5p was found to be significantly decreased in AR patients (Fig. 4). Taken together, independent TaqMan real-time qPCR expression results confirmed the validity of differentially expressed vesicle miRNAs identified by human microRNA assay and our results defined a profile of dysregulated vesicle miRNA signatures related to AR, which revealed that these miRNAs may have a functional role in the pathogenesis of AR.
AR is a common disease characterized by chronic inflammation of the nasal mucosa,2627 but we have not fully understood the mechanism for the development of AR. In this study, we report that a substantial group of vesicle miRNA expressions are altered in AR and that differentially expressed miRNAs appear to be involved in the development of AR. Our finding may lead to a better understanding about the roles of identified vesicle miRNAs in the pathogenesis of AR; this would be considered in future therapeutic strategies.
In this study, for the first time, we have, verified the presence of miRNA in extracellular vesicles isolated from the supernatant of nasal mucus from AR patients. Furthermore, we revealed alterations in the vesicle miRNA profiles from AR patients, suggesting an intrinsic dysregulation of vesicle miRNA content during AR. Increasing evidence supports the importance of miRNA regulation in development of AR, but the regulatory mechanism has so far been poorly defined. Prediction and identification of miRNA-targeting genes offers an experimental basis for further research on miRNA regulatory mechanisms. Bioinformatic methods based on sequence similarities between targets and miRNAs were used to predict the potential target genes. In this study, we utilized DIANA-mirPath to demonstrate that 32 KEGG biological processes were significantly enriched among differentially expressed vesicle miRNA signatures, including the B-cell receptor signaling pathway, the natural killer cell-mediated cytotoxicity, the T-cell receptor signaling pathway, the RIG-I-like receptor signaling pathway, the Wnt signaling pathway, endocytosis, and salivary secretion. It further expanded to some other important pathways, such as RNA degradation, the mRNA surveillance pathway, the Wnt signaling pathway, the adipocytokine signaling pathway, and leukocyte transendothelial migration. Taken together, these results might indicate that vesicle miRNAs exert a moderating regulatory function in AR. Exosomes are either released from the cell when multivesicular bodies fuse with the plasma membrane or they are released directly from the plasma membrane.28 It would be helpful to know the origin of exosomes to evaluate the importance and function of altered miRNAs in AR. Numerous investigators have actively researched the role that exosomes may play in cell-to-cell signaling, hypothesizing that because exosomes can merge with and release their contents into cells that are distant from their cell of origin, they may influence processes in the recipient cell. For example, RNA that is shuttled from one cell to another, known as "vesicle shuttle RNA," could potentially affect protein production in the recipient cell.829 By transferring molecules from one cell to another, exosomes from certain cells of the immune system, such as dendritic cells and B cells, may play a functional role in mediating adaptive immunity.30
MiRNAs are critical post-transcriptional regulators of gene expression that control the development of AR. Deregulation of miRNA-mediated mechanisms is emerging as an important pathological factor for allergy-related disease.1415 Yu et al.31 compared the miRNA expression profiles in nasal mucosa from AR patients and nonallergic controls by means of microarray. They identified 9 differentially expressed miRNAs with a more than 2-fold change, including 2 up-regulated miRNAs and 7 down-regulated miRNAs. Further quantitative RT-PCR assay confirmed the down-regulation of hsa-miR-224, hsa-miR-187, and hsa-miR-143 in AR.31 Employing quantitative RT-PCR, Soujalehto et al.32 measured the expression levels of selected 35 miRNAs that were formerly identified as differentially expressed miRNAs in AR in Yu's study or considered to relate to inflammation or immunological responses by earlier studies. They found that subjects with current AR symptoms have increased levels of miR-155, miR-205, and miR-498, but reduced levels of let-7e in nasal mucosa. They also found that patients with positive skin prick test results show increased miR-155/miR-205 but decreased let-7a expressions as compared to subjects with negative skin prick test results. Therefore, dysregulated microRNAs may play an important role in the development of AR.
Although studying miRNA expression profiles in tissue can provide us with an overview of gene regulation in diseased mucosa, there may be a significant degree of cellular heterogeneity across individual biopsies. It is confusing that we can see more discrepancies than consistency in miRNA profiles in AR between Yu's and Soujalehto's studies. The underlying reasons are unclear but may be related to the heterogeneity of the tissue. The other limitation of their studies is that functions of aberrantly expressed miRNAs in AR have not been investigated. Chen et al.33 screened for the expression of a panel of 157 miRNAs in mononuclear leucocytes from human umbilical CB samples and analyzed the association of miRNA expression with the development of AR. They found that miR-21 and miR-126 levels are significantly lower in CB with elevated IgE levels and in monocytes from AR children. Transfection of miR-21 precursor into monocytes suppressed transforming growth factor beta receptor 2 (TGFBR2) expression, suggesting a role of miR-21 to regulate TGFBR2.33 In our study, we have predicted that some miRNAs target genes, such as c-Fos, Lyn, MUC7, and GNAC, are associated with development of AR. Previous evidence demonstrated that c-fos is a regulator of degranulation and cytokine production in FcεRI-activated mast cells,34 which play a significant role in the initiation of many inflammatory responses, such as allergy and allergy-associated diseases. A role for Lyn kinase as a positive regulator of immunoglobulin (Ig) E-dependent allergy has long been accepted. Contrary to this belief, Lyn kinase has been found to have an important role as a negative regulator of the allergic response.35 MUC7 is differentially expressed in mucous and serous cells of submucosal glands in human bronchial airways of asthmatic patients.36 Based on these studies, we believe that miRNAs regulating c-fos, Lyn, and MUC7 possibly have a significant function during disease progression of AR. In the future, we will study the mechanism of dysregulated miRNAs in extracellular vesicles during the development of AR.
Compared to mRNAs and even proteins, miRNAs are in a relatively stable form, which makes miRNAs a potential candidate as noninvasive biomarkers. Some preclinical studies on cancer offer an exciting possibility of miRNAs acting as biomarkers for disease diagnosis, stratification, and monitoring.3738 The finding that miR-21 has the potential to facilitate the diagnosis of AR in newborn also highlights the possibility of miRNAs as biomarkers for diagnosis and prognosis of inflammatory upper airway diseases.33 Suppression of overexpressed miRNAs or restoration of down-regulated miRNAs in different disease conditions may be of therapeutic profit. Intravenous or local delivery of synthetic miRNAs and their inhibitors in mice has shown little evidence of toxicity.39 Currently, there is a lack of in vivo studies regarding the therapeutic effect of miRNAs in inflammatory upper airway disease. There is no doubt that the translational studies on the clinical usage of miRNAs in inflammatory upper airway disease will finally change our approach to the diagnosis, prognosis, and treatment of AR. Nevertheless, despite the promising efficacy and safety of miRNAs, the efficacy and specificity of the chosen miRNAs/anti-miRNAs, their ability to be effectively delivered to the disease site, and their chronicity once they reach their intended location are all aspects for intense development in future. To conclude, our study reveals that there could be a substantial dysregulated vesicle miRNAs in patients with AR and will aid in future clinical interventions.
Figures and Tables
Fig. 1
Flow cytometric analysis of extracellular vesicles displayed expression of CD63 and MHCII surface markers. Results are shown as the MFI for the detected molecule divided by the MFI for the isotype control. Data shown are representative of at least 3 independent experiments and are shown as mean±SEM.
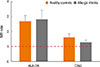
Fig. 2
Correlation between TaqMan microRNA and TaqMan real-time RT-PCR assays. Eight detectable miRNAs (miR-146a, miR-155, let-7a, miR-181a, miR-454, let-7b, miR-28-3p, and miR-885-5p) were selected for the evaluation of the 384-well microfluidic card, by independent TaqMan real-time RT-PCR on the same samples. Data are represented representative of 3 repetitive experiments.
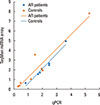
Fig. 3
Differentially expressed vesicle miRNAs in the B-cell receptor signaling pathway. (A) Diagram of the B-cell receptor signal pathway. Four dysregulated miRNAs (miR-30-5p, miR-199b-3p, miR-874, and miR-28-3p) were associated with the B-cell receptor signaling pathway by possibly regulating their potential gene targets. (B) Quantitative expression of miR-30-5p, miR-199b-3p, miR-874, and miR-28-3p as assessed by TaqMan real-time RT-PCR in individual nasal mucus vesicle samples from 44 AR patients and 20 healthy controls. Relative expression of these miRNAs was statistically differentially expressed compared to healthy controls (P<0.05, Mann Whitney U test). All qRT-PCR reactions were performed in triplicate, and the Ct values greater than 35 from real-time PCR assays were treated as 35. The delta Ct values were calculated by using RNU44 as the endogenous control.
*P<0.05; **P<0.01.
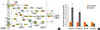
Fig. 4
Differentially expressed vesicle miRNAs in the salivary secretion signal pathway. (A) Two dysregulated miRNAs (miR-203 and miR-875-5p) as examples for examining miRNA-mRNA relationships in salivary secretion. (B) Quantitative expression of miR-203 and miR-875-5p as assessed by TaqMan real-time RT-PCR in individual nasal mucus vesicle samples from 44 AR patients and 20 healthy controls. Relative expression of both miRNAs was statistically differentially expressed compared to healthy controls (P<0.05, Mann Whitney U test). All qRT-PCR reactions were performed in triplicate, and the Ct values greater than 35 from the real-time PCR assays were treated as 35. The delta Ct values were calculated by using RNU44 as the endogenous control.
*P<0.05; **P<0.01.
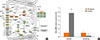
Table 1
Selected clinical features and demographic characteristics of the study subjects
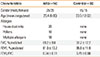
Table 2
Differential miRNA expression in AR patients
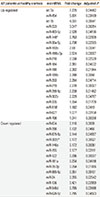
Table 3
Biologic pathways enriched by differentially expressed miRNAs in AR patients
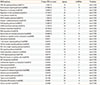
References
1. Bousquet J, Khaltaev N, Cruz AA, Denburg J, Fokkens WJ, Togias A, et al. Allergic Rhinitis and its Impact on Asthma (ARIA) 2008 update (in collaboration with the World Health Organization, GA(2)LEN and AllerGen). Allergy. 2008; 63:Suppl 86. 8–160.
2. Aït-Khaled N, Pearce N, Anderson HR, Ellwood P, Montefort S, Shah J, et al. Global map of the prevalence of symptoms of rhinoconjunctivitis in children: the International Study of Asthma and Allergies in Childhood (ISAAC) Phase Three. Allergy. 2009; 64:123–148.
3. International Rhinitis Management Working Group. International Consensus Report on the diagnosis and management of rhinitis. Allergy. 1994; 49:1–34.
4. van Niel G, Raposo G, Candalh C, Boussac M, Hershberg R, Cerf-Bensussan N, et al. Intestinal epithelial cells secrete exosome-like vesicles. Gastroenterology. 2001; 121:337–349.
5. Admyre C, Johansson SM, Qazi KR, Filén JJ, Lahesmaa R, Norman M, et al. Exosomes with immune modulatory features are present in human breast milk. J Immunol. 2007; 179:1969–1978.
6. Michael A, Bajracharya SD, Yuen PS, Zhou H, Star RA, Illei GG, et al. Exosomes from human saliva as a source of microRNA biomarkers. Oral Dis. 2010; 16:34–38.
7. Lässer C, Alikhani VS, Ekström K, Eldh M, Paredes PT, Bossios A, et al. Human saliva, plasma and breast milk exosomes contain RNA: uptake by macrophages. J Transl Med. 2011; 9:9.
8. Valadi H, Ekström K, Bossios A, Sjöstrand M, Lee JJ, Lötvall JO. Exosome-mediated transfer of mRNAs and microRNAs is a novel mechanism of genetic exchange between cells. Nat Cell Biol. 2007; 9:654–659.
9. Yuan A, Farber EL, Rapoport AL, Tejada D, Deniskin R, Akhmedov NB, et al. Transfer of microRNAs by embryonic stem cell microvesicles. PLoS One. 2009; 4:e4722.
10. Zomer A, Vendrig T, Hopmans ES, van Eijndhoven M, Middeldorp JM, Pegtel DM. Exosomes: fit to deliver small RNA. Commun Integr Biol. 2010; 3:447–450.
11. Ratajczak J, Miekus K, Kucia M, Zhang J, Reca R, Dvorak P, et al. Embryonic stem cell-derived microvesicles reprogram hematopoietic progenitors: evidence for horizontal transfer of mRNA and protein delivery. Leukemia. 2006; 20:847–856.
12. Ratajczak J, Wysoczynski M, Hayek F, Janowska-Wieczorek A, Ratajczak MZ. Membrane-derived microvesicles: important and underappreciated mediators of cell-to-cell communication. Leukemia. 2006; 20:1487–1495.
13. Mack M, Kleinschmidt A, Brühl H, Klier C, Nelson PJ, Cihak J, et al. Transfer of the chemokine receptor CCR5 between cells by membrane-derived microparticles: a mechanism for cellular human immunodeficiency virus 1 infection. Nat Med. 2000; 6:769–775.
14. Suojalehto H, Lindström I, Majuri ML, Mitts C, Karjalainen J, Wolff H, et al. Altered microRNA expression of nasal mucosa in long-term asthma and allergic rhinitis. Int Arch Allergy Immunol. 2014; 163:168–178.
15. Zhang XH, Zhang YN, Liu Z. MicroRNA in chronic rhinosinusitis and allergic rhinitis. Curr Allergy Asthma Rep. 2014; 14:415.
16. The European Academy of Allergology and Clinical Immunology. Position paper: allergen standardization and skin tests. Allergy. 1993; 48:48–82.
17. Ruocco L, Fattori B, Romanelli A, Martelloni M, Casani A, Samolewska M, et al. A new collection method for the evaluation of nasal mucus proteins. Clin Exp Allergy. 1998; 28:881–888.
18. Jansen FH, Krijgsveld J, van Rijswijk A, van den Bemd GJ, van den Berg MS, van Weerden WM, et al. Exosomal secretion of cytoplasmic prostate cancer xenograft-derived proteins. Mol Cell Proteomics. 2009; 8:1192–1205.
19. Johansson SM, Admyre C, Scheynius A, Gabrielsson S. Different types of in vitro generated human monocyte-derived dendritic cells release exosomes with distinct phenotypes. Immunology. 2008; 123:491–499.
20. Anglicheau D, Sharma VK, Ding R, Hummel A, Snopkowski C, Dadhania D, et al. MicroRNA expression profiles predictive of human renal allograft status. Proc Natl Acad Sci U S A. 2009; 106:5330–5335.
21. Papadopoulos GL, Alexiou P, Maragkakis M, Reczko M, Hatzigeorgiou AG. DIANA-mirPath: integrating human and mouse microRNAs in pathways. Bioinformatics. 2009; 25:1991–1993.
22. Reczko M, Maragkakis M, Alexiou P, Grosse I, Hatzigeorgiou AG. Functional microRNA targets in protein coding sequences. Bioinformatics. 2012; 28:771–776.
23. Paraskevopoulou MD, Georgakilas G, Kostoulas N, Vlachos IS, Vergoulis T, Reczko M, et al. DIANA-microT web server v5.0: service integration into miRNA functional analysis workflows. Nucleic Acids Res. 2013; 41:W169–W173.
24. Hsu SD, Lin FM, Wu WY, Liang C, Huang WC, Chan WL, et al. miR-TarBase: a database curates experimentally validated microRNA-target interactions. Nucleic Acids Res. 2011; 39:D163–D169.
25. Scian MJ, Maluf DG, David KG, Archer KJ, Suh JL, Wolen AR, et al. MicroRNA profiles in allograft tissues and paired urines associate with chronic allograft dysfunction with IF/TA. Am J Transplant. 2011; 11:2110–2122.
26. Zhang Y, Zhang L. Prevalence of allergic rhinitis in china. Allergy Asthma Immunol Res. 2014; 6:105–113.
27. Dhong HJ. Classification of allergic rhinitis: what is most suitable in Korea? Allergy Asthma Immunol Res. 2013; 5:65–67.
28. Booth AM, Fang Y, Fallon JK, Yang JM, Hildreth JE, Gould SJ. Exosomes and HIV Gag bud from endosome-like domains of the T cell plasma membrane. J Cell Biol. 2006; 172:923–935.
29. Balaj L, Lessard R, Dai L, Cho YJ, Pomeroy SL, Breakefield XO, et al. Tumour microvesicles contain retrotransposon elements and amplified oncogene sequences. Nat Commun. 2011; 2:180.
30. Li XB, Zhang ZR, Schluesener HJ, Xu SQ. Role of exosomes in immune regulation. J Cell Mol Med. 2006; 10:364–375.
31. Shaoqing Y, Ruxin Z, Guojun L, Zhiqiang Y, Hua H, Shudong Y, et al. Microarray analysis of differentially expressed microRNAs in allergic rhinitis. Am J Rhinol Allergy. 2011; 25:e242–e246.
32. Suojalehto H, Toskala E, Kilpeläinen M, Majuri ML, Mitts C, Lindström I, et al. MicroRNA profiles in nasal mucosa of patients with allergic and nonallergic rhinitis and asthma. Int Forum Allergy Rhinol. 2013; 3:612–620.
33. Chen RF, Huang HC, Ou CY, Hsu TY, Chuang H, Chang JC, et al. MicroRNA-21 expression in neonatal blood associated with antenatal immunoglobulin E production and development of allergic rhinitis. Clin Exp Allergy. 2010; 40:1482–1490.
34. Lee YN, Tuckerman J, Nechushtan H, Schutz G, Razin E, Angel P. c-Fos as a regulator of degranulation and cytokine production in FcepsilonRI-activated mast cells. J Immunol. 2004; 173:2571–2577.
35. Odom S, Gomez G, Kovarova M, Furumoto Y, Ryan JJ, Wright HV, et al. Negative regulation of immunoglobulin E-dependent allergic responses by Lyn kinase. J Exp Med. 2004; 199:1491–1502.
36. Sharma P, Dudus L, Nielsen PA, Clausen H, Yankaskas JR, Hollingsworth MA, et al. MUC5B and MUC7 are differentially expressed in mucous and serous cells of submucosal glands in human bronchial airways. Am J Respir Cell Mol Biol. 1998; 19:30–37.
37. Li T, Leong MH, Harms B, Kennedy G, Chen L. MicroRNA-21 as a potential colon and rectal cancer biomarker. World J Gastroenterol. 2013; 19:5615–5621.
38. Siasos G, Kollia C, Tsigkou V, Basdra EK, Lymperi M, Oikonomou E, et al. MicroRNAs: novel diagnostic and prognostic biomarkers in atherosclerosis. Curr Top Med Chem. 2013; 13:1503–1517.
39. Baxter D, McInnes IB, Kurowska-Stolarska M. Novel regulatory mechanisms in inflammatory arthritis: a role for microRNA. Immunol Cell Biol. 2012; 90:288–292.