Abstract
The prevalence of asthma and allergic disease has increased worldwide over the last few decades. Many common environmental factors are associated with this increase. Several theories have been proposed to account for this trend, especially those concerning the impact of environmental toxicants. The development of the immune system, particularly in the prenatal period, has far-reaching consequences for health during early childhood, and throughout adult life. One underlying mechanism for the increased levels of allergic responses, secondary to exposure, appears to be an imbalance in the T-helper function caused by exposure to the toxicants. Exposure to environmental endocrine-disrupting chemicals can result in dramatic changes in cytokine production, the activity of the immune system, the overall Th1 and Th2 balance, and in mediators of type 1 hypersensitivity mediators, such as IgE. Passive exposure to tobacco smoke is a common risk factor for wheezing and asthma in children. People living in urban areas and close to roads with a high volume of traffic, and high levels of diesel exhaust fumes, have the highest exposure to environmental compounds, and these people are strongly linked with type 1 hypersensitivity disorders and enhanced Th2 responses. These data are consistent with epidemiological research that has consistently detected increased incidences of allergies and asthma in people living in these locations. During recent decades more than 100,000 new chemicals have been used in common consumer products and are released into the everyday environment. Therefore, in this review, we discuss the environmental effects on allergies of indoor and outside exposure.
Epidemiological research suggests that exposure to environmental toxins (such as pesticides, solvents, and air pollutants) is associated with an increased prevalence of asthma and allergies. Asthma and allergic diseases are the most common chronic inflammatory diseases in children, and represent a substantial morbidity and mortality burden in severe cases.1 The prevalence of allergic disorders has seen a worldwide increase over the last few decades. Most countries report population rates of allergic diseases (such as allergic rhinitis and asthma) between 15%-35%, with the worldwide average being 22%.2 Asthma prevalence has been increasing since the early 1980s and by 2004, 1 in 15 Americans suffered from asthma.3 Exposure to environmental toxicants not only contributes to the increasing prevalence of asthma and allergies, it also affects disease outcomes, many of which are due to an underlying immune and inflammatory dysfunction.4 Mounting epidemiological evidence suggests that a proper functioning of the immune system is a major determinant of health across the course of a lifetime; for example, adults who suffered with childhood asthma may have an elevated risk of developing lung cancer.4 Therefore, exposure to environmental toxicants may cause life-long changes in responses to infectious agents and in the homeostasis of the immune system, as well as in our overall physical health. Epidemiological studies have suggested that following exposure to air pollution, variant genes controlling the innate immune response and the subsequent inflammatory reactions, are associated with an adverse respiratory outcome.5 Some environmental toxins, such as endocrine disrupting chemicals (EDCs), imitate the action of steroid hormones and have promoted endocrine and reproductive disorders, both in animal and human studies. These environmental toxins interfere with the synthesis of cytokines, immunoglobulins, and inflammatory mediators, and they also affect the activation and survival of immune cells. The dysfunction of the immune system caused by some EDCs may lead to attenuation of immunity immunodeficiency against infection, or to hyperreactivity of immune responses (allergy and autoimmune disease). In vitro and in vivo studies have demonstrated that many of the environmental chemicals and pollutants that have been associated with increased allergic tendency promote type 2 helper T cell (Th2) reactions, consistent with Th2 predominant responses found in asthma and allergic rhinitis. In this review, we have summarized epidemiologic, animal, and cell studies to demonstrate the impact of indoor and outdoor environmental toxins on allergy diseases and the associated immune mechanisms, such as the regulatory effects of epigenetics.
EDCs have increased since World War II6, and this coincides with an increased prevalence of autoimmune and allergic diseases.7 EDCs are ubiquitous in the environment, and are found in the air, water, and the soil, and many have undesirable effects on human health. EDCs are regarded as inducers of the inflammatory response, and this can be via the estrogen receptor, or other receptors, such as the aryl hydrocarbon receptor.8 EDCs have also been reported as potential modulators of the immune system and allergic responses in allergic disease.9 For example, a high concentration of diethyl hexyl phthalate (DEHP) in indoor dust is associated with wheezing in preschool children. Another alkyphenol, p-octylphenol, has been shown to enhance strong Th2 polarization via suppression of type 1 helper T cell (Th1) and augmentation of Th2 immune responses, respectively.6,10 Recently, new evidence has shown that exposure to alkylphenols plays a key role in the allergic response that may be associated with the development of asthma. Due to the low solubility, high hydrophobicity, and low estrogenic activity, alkylphenols tend to accumulate in the human body and lead to promote the development as well as progression of allergic diseases. Alkylphenols exert their effects on several key cell types in the context of allergic inflammation.
Nonylphenol (NP), one of the alkylphenols, is the most important metabolite of a group of nonionic surfactants, designated as NP polyethoxylates11 and is one of the common EDCs.12 NP decomposes in the environment, and it can influence human health via bioaccumulation in the diet or through other contact means.13 NP is structurally similar to 17β-estradiol, which feminizes male animals and has possible links to infertility.14 Some in vitro or ex vivo studies suggest that NP skews T cells towards Th2 responses through its influence on dendritic cells (DCs). For example, splenic conventional DCs from NP-exposed mice have shown a potent Th2-skewing ability and express increased levels of IL-6 and TNF-α, but not IL-10 and IL-12, in response to LPS stimulation.15 Further, bone marrow-derived DCs in the presence of NP can influence antigen-specific T cells to secrete significantly less IFN-γ.15 Importantly, NP-exposed mice developed relatively more severe OVA-induced allergic lung inflammation.15 Plasmacytoid DCs (pDCs) are the predominant cells that secrete type I IFN during infection. Type I IFN increases the survival of T cells, the differentiation of Th1 and cytotoxic T lymphocytes, and also increases the activity of natural killer cells, leading to an overall boost in the antiviral T-cell activity.16 Concurrently, type 1 IFNs suppress the Th2 immune response.17 We previously reported that NP and 4-octylpnehol (4-OP) influenced the function of pDCs in vitro and in vivo by inducing the pro-inflammatory cytokine TNF-α, and by suppressing regulatory cytokines such as IL-10, IFN-α and IFN-β, suggesting the potential impact of endocrine disrupting chemicals on immune regulation.18 Accordingly, exposure to NP may be linked to an impairment in anti-viral immunity.
The tendency to develop asthma and other chronic allergic inflammatory disorders such as allergic rhinitis, allergic conjunctivitis and atopic dermatitis, is inherited, but it can be influenced by the environment and modified by in utero exposure and aging. These features are all linked to epigenetic regulation. Environmental exposure, especially prenatal maternal smoking, has been associated with asthma and can be partly explained by epigenetic regulation. There are several forms of epigenetic regulation, including DNA methylation or histone modification. NP increased the expression of TNF-α, but suppressed IL-10 production in a range of physiological doses, concomitant with activation of the MKK3/6-p38 signaling pathway and enhanced levels of acetylated histone 3, and histone 4 at the TNFA gene locus. NP or 4-OP-induced increased TNF-α levels in myeloid DCs (mDCs), which was concomitant with increasing levels of methyltranferase complex mixed-lineage leukemia and tryptophan-aspartic acid repeat domain 5 (WDR5) in the nucleus, that subsequently contributed to the increasing levels of trimethylated H3K4 at the TNFA gene locus. The up-regulated TNF-α expression by NP could be suppressed by a histone acetyltransferase inhibitor. In the presence of NP-treated mDCs, T cells showed increased levels of IL-13 but decreased expression of IFN-γ.19 In a cell study, NP suppressed type I IFN production in response to CpG stimulation, along with down-regulation of IRF-7 and involvement of the MKK1/2-ERK-Elk-1 pathways, leading to the impairment of anti-enterovirus 71 activities. Furthermore, splenic pDCs from NP-exposed mice showed similar cytokine changes upon CpG stimulation under conditions relevant to routing levels of exposure in humans. The exposure to NP promoted allergic lung inflammation in a murine model of asthma.15,18
Phthalates are compounds added to plastics to make them more flexible. Phthalates can easily be released into the surrounding environment because they are not strongly bound to the plastics. At least 2 types of phthalates are reported to be linked with higher rates of allergic respiratory incidences. In a cohort study of 10,852 children, exposure to butyl benzyl phthalate (BBzP) and DEHP was associated with rhinitis, eczema and asthma.20 DEHP is found in all PVC products, and exposure to PVC flooring during pregnancy could be a critical factor in the development of asthma in children.21 Phthalates are reported to enhance Th2 differentiation, the production of Th2 cytokines, and increase levels of Th2-promoted immunoglobulins (mainly IgG1 but also IgE) in mice.22 Similar to NP, phthalates may also interfere with immunity against viral infection and promote a decrease in the Th2 response, thus increasing the allergic reaction by acting on human pDCs via suppression of IFN-α/IFN-β expression and modulation of the ability to stimulate T-cell responses. DEHP and BBP can suppress CpG-induced IFN-α/IFN-β expression in pDCs by suppressing CpG-induced interferon regulatory factor (IRF)-7 expression, via suppression of the histone H3K4 trimethylation at the IRF7 gene promoter region and inhibiting translocation of H3K4-specific trimethyltransferase WDR5 from the cytoplasm into the nucleus. BBP- or DEHP-treated pDCs also suppress IFN-γ while enhancing IL-13 production by CD4+ T cells.23
Cigarette smoke contains harmful chemicals with hazardous effects on almost every organ in the body of smokers, and non-smokers exposed to environmental tobacco smoke (ETS). At least 40,000,000 children are regularly exposed to ETS every year, increasing their risk for acute respiratory infections. It has been recognized that asthma and wheezing are more common in children exposed to cigarette smoke. Tobacco smoke is thought to be the main indoor air pollutant and can lead to higher rates of allergy and asthma, with early exposure to ETS being associated with wheezing in children.24 Diseases of the airways in later life can often be influenced by environmental exposure that occurred during critical developmental periods of the immune systems.25 Maternal smoking is of greater significance than paternal smoking and prenatal exposure is also more significant than postnatal exposure for childhood allergic sensitization.26 Exposure to maternal smoking prenatally and after birth also increased the risk of wheezing and asthma in children.26 Thus, avoiding tobacco smoke exposure during pregnancy and in early childhood might prevent or delay the development of asthma.27 Passive smoking also increases the incidences of wheezing and asthma in children and young people by at least 20%. Therefore, stopping parents smoking is crucially important to the prevention of childhood asthma.28 Second-hand smoke exposure has a dose-dependent synergy with a family history of asthma. Avoiding second-hand smoke may be an important preventative method for reducing the risk of adult-onset asthma among those with asthmatic heredity.29 Detectable levels of serum and salivary cotinine, a marker for tobacco smoke exposure, are common in children admitted for asthma.30 Detectable serum and salivary cotinine levels are also associated with re-admission in children hospitalized for asthma.30 There is evidence to support an association between the development of asthma and tobacco smoke exposure, and evidence is mounting to suggest that exposure to tobacco smoke may also increase the risk of IgE sensitization.31 Tobacco smoke reduces the Th1 response by suppressing IFN-γ production and natural killer cell activity, but it enhances the Th2 response by increasing production of IL-4, IL-5 and other pro-inflammatory cytokines that result in increased allergic responses.32,33,34
There is an association between heavy metals and allergic reactions. Prenatal and early postnatal exposure to heavy metals, such as lead and mercury, has been reported to skew immune responses toward a Th2 bias and elevate production of IgE or Th2-related cytokines. Lead exposure in mice promotes a Th2 reaction and suppresses IFN-γ expression.35 Mercuric chloride induces a higher IgE production and increases IL-4 levels.36 In a study of children, increased ambient nickel and vanadium concentrations were significantly associated with an increased probability of wheezing at 24 months old.37
Epidemiologic studies have suggested that prenatal and early childhood exposure to diesel exhaust plays a role in the development of allergies. The offspring of mice exposed to diesel exhaust particles (DEP) were hypersensitive to ovalbumin (OVA), indicated by inflammation of the airways and hyperresponsiveness, increased serum OVA-specific IgE levels, and increased pulmonary and systemic Th2 and type 17 helper T cell (Th17) cytokine levels.38 However, exposure to DEPs alone did not induce asthma in mice. The co-exposure of DEPs and house dust mites (HDM) markedly enhanced airway hyperresponsiveness compared with HDM exposure alone and induced Th2 and Th17 responses, including IL-13+, IL-17A+ double-producing T cells.39 In a recent study of children with allergic asthma, those exposed to high levels of DEPs had more frequent symptoms over a 12-month period (32.2%) compared with only 14.2% in a low DEP-exposure group. Children with allergic asthma exposed to high DEP levels had ~6 fold higher serum IL-17A levels compared with low DEP-exposed children.39
Particulate matter (PM) is a heterogeneous mixture of small particles and liquid droplets suspended in the air. PM can be classified into three categories based on the particle size. Coarse PM (diameter, 2.5-10 µm) is derived from abraded soil, road dust, construction debris and an aggregation of smaller combustion particles. Fine (≤2.5 µm) and ultrafine (≤0.1 µm) PM is formed during the combustion of fossil fuel products. Diesel exhaust is a major source of particulates in urban areas, and accounts for most airborne PM. Particulates can stimulate our immune system to induce inflammatory reactions. Several nanometer-to micrometer-sized particulates, such as particle matter 2.5 (PM2.5), diesel particles, and sand dust, induce pulmonary inflammation, and especially allergic asthma. Exposure to PM air pollution induces an exacerbation of asthma in children, and their exposure to PM is likely to include combustion-related ambient PM and PM related to the child's activity in their indoor and outdoor microenvironments. PM can pass through the throat and nose and enter the lungs, and has an adverse effect on many organs in the body. PM size may be directly linked to its potential to induce immune responses and cause allergy problems. PM2.5 may be more respirable and hence more toxic. There is an association between ambient PM2.5 and the development of allergic asthma and exacerbation of acute asthma. There is also increasing evidence to link ambient levels of PM2.5 to declining lung function, and increased reliance on the need for medical services to treat asthma.40,41,42 PM components from fossil fuel combustion can induce oxidative stress initiated by reactive oxygen species (ROS). Reported associations between worsening asthma and PM2.5 mass could be related to the oxidative potential of PM to induce airway inflammation as a result of oxidative stress. DEPs contribute significantly to urban PM2.5 concentrations.43 The decrease in the FEV1 of asthma patients was significantly associated with increased hourly peaks and the daily average levels of PM2.5.40,44 Nanometer- to micrometer-sized crystals, spheres, and hydrogel forms of aluminum salts have been used as vaccine adjuvants to enhance antibody responses, and most of these particulates induce Th2 immune responses.45
Several particulates exhibit adjuvant effects in immune responses For example, aluminum can selectively stimulate humoral immune responses, especially Th2 immune responses, which are characterized by the production of IL-4 and IL-5 and the induction of IgE and IgG1.46,47 Crystalline silica can also induce Th2 responses and antigen-specific IgE and IgG1.48 Synthesized particles, such as polystyrene particles, poly lactic-co-glycolic acid, nickel oxide nanoparticles and carbon nanotubes, induce humoral immunity, especially antigen-specific production of IgG1 and IgE.49,50,51 Epidemiologic studies have consistently shown that PM can cause asthma and allergies in children.52,53,54
Pesticides are widely used to control pest and pest-related diseases in agriculture, fishery, forestry, and also in the food industry. There are a number of respiratory diseases and symptoms that have been associated with occupational exposure to pesticides. Impaired lung function has also been found in people suffering from allergies who are occupationally exposed to pesticides. Several respiratory symptoms, such as coughing, wheezing and airway inflammation, are commonly observed in people exposed to pesticides.55,56 Exposure to pesticides in utero has been reported as a risk for childhood asthma.57 There is strong evidence of an association between occupational exposure to pesticides and asthma, especially in agriculture. If the fetus is exposed to certain pesticides during pregnancy, allergies and hay fever appear to be more common in the offspring, and especially in the male offspring.57 The potential effects of early exposure to pesticides may be confounded by the generally protective effects of in utero exposure to the farmyard environment and exposure to livestock. Livestock farmers on farms had significantly lower incidences of allergic rhinitis than crop farmers.58 There is little antigenicity in chemical pesticides, pesticide-induced or -promoted allergic/atopic asthma. Therefore, pesticide-induced allergy may be due to the indirect effects of pesticides on the immune system, such as interfering with the Th1/Th2 balance, or pesticide-induced oxidative stress.59 There is an urgent need for training and education on basic safety precautions and the proper use of personal protection equipment. This type of intervention could control the incidences of respiratory disease associated with pesticide exposure in an occupational setting. We summary the potential immunological mechanisms induced by environmental toxicant on allergic inflammation in Table and Figure.
Allergic diseases affect a significant percentage of children, and represent the largest group of pediatric chronic diseases in most countries. A wide range of environmental factors and toxins are associated with childhood allergic diseases. Air pollution and toxins should be targeted for intervention as they are a major cause of allergic diseases, particularly in children. Because most of the risk factors are controllable, disease prevention by avoidance of prenatal and neonatal environmental risk factors may offer significant promise to reduce the prevalence of pediatric allergies. However, these established risk factors cannot account for all the recent increases in immune-dependent pediatric asthma. Therefore, new chemical and drug safety testing strategies are needed to identify the main environmental factors and to ensure adequate protection of the most vulnerable i.e., pregnant women and children, from allergy-related developmental immunotoxicity. Early-life immune dysfunction/imbalance has been recognized as a biomarker for the risk of pediatric allergy and asthma. Oxidative stress and epigenetic mechanisms are also considered as possible factors in the development of allergic disease and asthma. Further studies to identify the major environmental risks and to understand how these mechanisms regulate gene-environment interactions will lead us to develop new methods of controlling or preventing allergic disease in children.
Figures and Tables
Figure
Possible mechanisms of the effects of environmental toxins on allergic inflammation. Alkyphenols and phthalates alter the function of human plasmacytoid dendritic cells (pDC) and myeloid DCs (mDC) by changing the expression cytokines, including tumor necrosis factor-α (TNF-α), interleukin (IL)-10, interferon (IFN)-α and IFN-β via the epigenetic regulation by histone acetylation as well as trimethylation. Alkyphenols and phthalates change the T cell stimulation function of DCs that promote Th2 development but suppress Th1 development. Heavy metals suppress Th1 development by inhibiting IFN-γ expression and promote Th2 development by enhancing IL-4 expression, and increase the production of IgE. Heavy metals also increase IgE-dependent basophil-mediated inflammation. Tobacco smoke suppresses the Th1 immune response by inhibiting IFN-γ expression and the survival of natural killer T cells, and promotes the Th2 immune response by increasing IL-4, IL-5, and IL-13 expression. Diesel exhaust particles increase IgE levels, and pulmonary and systemic Th2 and Th17 cytokine levels.
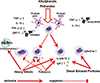
Table
Possible mechanisms of the effects of environmental toxins on allergic inflammation
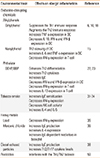
Environmental toxin | Effects on allergic inflammation | Reference |
---|---|---|
Endocrine-disrupting chemicals | ||
Alkylphenols | ||
Octylphenol | Suppresses the Th1 immune response | 6, 10, 18 |
Augments the Th2 immune response | ||
Increases TNF-α expression in DC | ||
Decreases IL-10, IFN-α and IFN-β expression in DC | ||
Nonylphenol | Th2-skewing of DC | 15 |
Increases IL-6 and TNF-α expression in DC | ||
Decreases IFN-γ expression in T cell | ||
Phthalate | ||
DEHP/BBP | Enhances Th2 differentiation | 22, 23 |
Increases Th2 cytokines | ||
Increases IgE | ||
Decreases IFN-α and IFN-β expression in DC | ||
Decreases IFN-γ expression in T cell | ||
Increases IL-13 expression in T cell | ||
Tobacco smoke | Increases IgE sensitization | 31, 32, 33, 34 |
Decreases IFN-γ expression | ||
Decreases NK cell activity | ||
Increases IL-4 and IL-5 | ||
Heavy metals | ||
Lead | Decreases IFN-γ expression | 35 |
Mercuric chloride | Increases IgE production | 36 |
Increases IL-4 expression | ||
Increases IgE-dependent mediators in basophil | ||
Diesel exhaust particles | Increases IgE production | 38 |
Increases Th2/Th17 cytokine levels | ||
Pesticides | Interferes with the Th1/Th2 balance | 59 |
References
1. Wenzel SE, Barnes PJ, Bleecker ER, Bousquet J, Busse W, Dahlén SE, et al. A randomized, double-blind, placebo-controlled study of tumor necrosis factor-alpha blockade in severe persistent asthma. Am J Respir Crit Care Med. 2009; 179:549–558.
2. Warner JO, Kaliner MA, Crisci CD, Del Giacco S, Frew AJ, Liu GH, et al. Allergy practice worldwide: a report by the World Allergy Organization Specialty and Training Council. Int Arch Allergy Immunol. 2006; 139:166–174.
3. Centers for Disease Control and Prevention (US). National surveillance for asthma ---United States, 1980--2004 [Internet]. Atlanta (GA): Centers for Disease Control and Prevention;2007. cited 2012 Feb 1. Available from: http://www.cdc.gov/mmwr/preview/mmwrhtml/ss5608a1.htm.
4. Dietert RR, DeWitt JC, Germolec DR, Zelikoff JT. Breaking patterns of environmentally influenced disease for health risk reduction: immune perspectives. Environ Health Perspect. 2010; 118:1091–1099.
5. Vawda S, Mansour R, Takeda A, Funnell P, Kerry S, Mudway I, et al. Associations between inflammatory and immune response genes and adverse respiratory outcomes following exposure to outdoor air pollution: a HuGE systematic review. Am J Epidemiol. 2014; 179:432–442.
6. Kolarik B, Naydenov K, Larsson M, Bornehag CG, Sundell J. The association between phthalates in dust and allergic diseases among Bulgarian children. Environ Health Perspect. 2008; 116:98–103.
7. Weiss ST. Eat dirt--the hygiene hypothesis and allergic diseases. N Engl J Med. 2002; 347:930–931.
8. Brokken LJ, Giwercman YL. Gene-environment interactions in male reproductive health: special reference to the aryl hydrocarbon receptor signaling pathway. Asian J Androl. 2014; 16:89–96.
9. Chalubinski M, Kowalski ML. Endocrine disrupters--potential modulators of the immune system and allergic response. Allergy. 2006; 61:1326–1335.
10. Kato T, Tada-Oikawa S, Takahashi K, Saito K, Wang L, Nishio A, et al. Endocrine disruptors that deplete glutathione levels in APC promote Th2 polarization in mice leading to the exacerbation of airway inflammation. Eur J Immunol. 2006; 36:1199–1209.
11. Giger W, Brunner PH, Schaffner C. 4-Nonylphenol in sewage sludge: accumulation of toxic metabolites from nonionic surfactants. Science. 1984; 225:623–625.
12. White R, Jobling S, Hoare SA, Sumpter JP, Parker MG. Environmentally persistent alkylphenolic compounds are estrogenic. Endocrinology. 1994; 135:175–182.
13. Correa-Reyes G, Viana MT, Marquez-Rocha FJ, Licea AF, Ponce E, Vazquez-Duhalt R. Nonylphenol algal bioaccumulation and its effect through the trophic chain. Chemosphere. 2007; 68:662–670.
14. Fraser LR, Beyret E, Milligan SR, Adeoya-Osiguwa SA. Effects of estrogenic xenobiotics on human and mouse spermatozoa. Hum Reprod. 2006; 21:1184–1193.
15. Suen JL, Hsu SH, Hung CH, Chao YS, Lee CL, Lin CY, et al. A common environmental pollutant, 4-nonylphenol, promotes allergic lung inflammation in a murine model of asthma. Allergy. 2013; 68:780–787.
16. Asselin-Paturel C, Trinchieri G. Production of type I interferons: plasmacytoid dendritic cells and beyond. J Exp Med. 2005; 202:461–465.
17. So EY, Park HH, Lee CE. IFN-gamma and IFN-alpha posttranscriptionally down-regulate the IL-4-induced IL-4 receptor gene expression. J Immunol. 2000; 165:5472–5479.
18. Hung CH, Yang SN, Wang YF, Liao WT, Kuo PL, Tsai EM, et al. Environmental alkylphenols modulate cytokine expression in plasmacytoid dendritic cells. PLoS One. 2013; 8:e73534.
19. Hung CH, Yang SN, Kuo PL, Chu YT, Chang HW, Wei WJ, et al. Modulation of cytokine expression in human myeloid dendritic cells by environmental endocrine-disrupting chemicals involves epigenetic regulation. Environ Health Perspect. 2010; 118:67–72.
20. Bornehag CG, Sundell J, Weschler CJ, Sigsgaard T, Lundgren B, Hasselgren M, et al. The association between asthma and allergic symptoms in children and phthalates in house dust: a nested case-control study. Environ Health Perspect. 2004; 112:1393–1397.
21. Shu H, Jönsson BA, Larsson M, Nånberg E, Bornehag CG. PVC flooring at home and development of asthma among young children in Sweden, a 10-year follow-up. Indoor Air. 2014; 24:227–235.
22. Guo J, Han B, Qin L, Li B, You H, Yang J, et al. Pulmonary toxicity and adjuvant effect of di-(2-exylhexyl) phthalate in ovalbumin-immunized BALB/c mice. PLoS One. 2012; 7:e39008.
23. Kuo CH, Hsieh CC, Kuo HF, Huang MY, Yang SN, Chen LC, et al. Phthalates suppress type I interferon in human plasmacytoid dendritic cells via epigenetic regulation. Allergy. 2013; 68:870–879.
24. Gilmour MI, Jaakkola MS, London SJ, Nel AE, Rogers CA. How exposure to environmental tobacco smoke, outdoor air pollutants, and increased pollen burdens influences the incidence of asthma. Environ Health Perspect. 2006; 114:627–633.
25. Dietert RR, Etzel RA, Chen D, Halonen M, Holladay SD, Jarabek AM, et al. Workshop to identify critical windows of exposure for children's health: immune and respiratory systems work group summary. Environ Health Perspect. 2000; 108:Suppl 3. 483–490.
26. Raherison C, Pénard-Morand C, Moreau D, Caillaud D, Charpin D, Kopfersmitt C, et al. In utero and childhood exposure to parental tobacco smoke, and allergies in schoolchildren. Respir Med. 2007; 101:107–117.
27. Grabenhenrich LB, Gough H, Reich A, Eckers N, Zepp F, Nitsche O, et al. Early-life determinants of asthma from birth to age 20 years: a German birth cohort study. J Allergy Clin Immunol. 2014; 133:979–988.
28. Burke H, Leonardi-Bee J, Hashim A, Pine-Abata H, Chen Y, Cook DG, et al. Prenatal and passive smoke exposure and incidence of asthma and wheeze: systematic review and meta-analysis. Pediatrics. 2012; 129:735–744.
29. Lajunen TK, Jaakkola JJ, Jaakkola MS. The synergistic effect of heredity and exposure to second-hand smoke on adult-onset asthma. Am J Respir Crit Care Med. 2013; 188:776–782.
30. Howrylak JA, Spanier AJ, Huang B, Peake RW, Kellogg MD, Sauers H, et al. Cotinine in children admitted for asthma and readmission. Pediatrics. 2014; 133:e355–e362.
31. Moerloose KB, Robays LJ, Maes T, Brusselle GG, Tournoy KG, Joos GF. Cigarette smoke exposure facilitates allergic sensitization in mice. Respir Res. 2006; 7:49.
32. Singh SP, Mishra NC, Rir-Sima-Ah J, Campen M, Kurup V, Razani-Boroujerdi S, et al. Maternal exposure to secondhand cigarette smoke primes the lung for induction of phosphodiesterase-4D5 isozyme and exacerbated Th2 responses: rolipram attenuates the airway hyperreactivity and muscarinic receptor expression but not lung inflammation and atopy. J Immunol. 2009; 183:2115–2121.
33. Feng Y, Kong Y, Barnes PF, Huang FF, Klucar P, Wang X, et al. Exposure to cigarette smoke inhibits the pulmonary T-cell response to influenza virus and Mycobacterium tuberculosis. Infect Immun. 2011; 79:229–237.
34. Hogan AE, Corrigan MA, O'Reilly V, Gaoatswe G, O'Connell J, Doherty DG, et al. Cigarette smoke alters the invariant natural killer T cell function and may inhibit anti-tumor responses. Clin Immunol. 2011; 140:229–235.
35. Strenzke N, Grabbe J, Plath KE, Rohwer J, Wolff HH, Gibbs BF. Mercuric chloride enhances immunoglobulin E-dependent mediator release from human basophils. Toxicol Appl Pharmacol. 2001; 174:257–263.
36. Nyland JF, Fillion M, Barbosa F Jr, Shirley DL, Chine C, Lemire M, et al. Biomarkers of methylmercury exposure immunotoxicity among fish consumers in Amazonian Brazil. Environ Health Perspect. 2011; 119:1733–1738.
37. Patel MM, Hoepner L, Garfinkel R, Chillrud S, Reyes A, Quinn JW, et al. Ambient metals, elemental carbon, and wheeze and cough in New York City children through 24 months of age. Am J Respir Crit Care Med. 2009; 180:1107–1113.
38. Manners S, Alam R, Schwartz DA, Gorska MM. A mouse model links asthma susceptibility to prenatal exposure to diesel exhaust. J Allergy Clin Immunol. 2013; pii: S0091-6749(13)01709-0.
39. Brandt EB, Kovacic MB, Lee GB, Gibson AM, Acciani TH, Le Cras TD, et al. Diesel exhaust particle induction of IL-17A contributes to severe asthma. J Allergy Clin Immunol. 2013; 132:1194–1204.e2.
40. Delfino RJ, Staimer N, Tjoa T, Gillen D, Kleinman MT, Sioutas C, et al. Personal and ambient air pollution exposures and lung function decrements in children with asthma. Environ Health Perspect. 2008; 116:550–558.
41. Mar TF, Larson TV, Stier RA, Claiborn C, Koenig JQ. An analysis of the association between respiratory symptoms in subjects with asthma and daily air pollution in Spokane, Washington. Inhal Toxicol. 2004; 16:809–815.
42. Halonen JI, Lanki T, Yli-Tuomi T, Kulmala M, Tiittanen P, Pekkanen J. Urban air pollution, and asthma and COPD hospital emergency room visits. Thorax. 2008; 63:635–641.
43. Schauer JJ. Evaluation of elemental carbon as a marker for diesel particulate matter. J Expo Anal Environ Epidemiol. 2003; 13:443–453.
44. Delfino RJ, Quintana PJ, Floro J, Gastañaga VM, Samimi BS, Kleinman MT, et al. Association of FEV1 in asthmatic children with personal and microenvironmental exposure to airborne particulate matter. Environ Health Perspect. 2004; 112:932–941.
45. Kuroda E, Coban C, Ishii KJ. Particulate adjuvant and innate immunity: past achievements, present findings, and future prospects. Int Rev Immunol. 2013; 32:209–220.
46. Aimanianda V, Haensler J, Lacroix-Desmazes S, Kaveri SV, Bayry J. Novel cellular and molecular mechanisms of induction of immune responses by aluminum adjuvants. Trends Pharmacol Sci. 2009; 30:287–295.
47. Marrack P, McKee AS, Munks MW. Towards an understanding of the adjuvant action of aluminium. Nat Rev Immunol. 2009; 9:287–293.
48. Mancino D, Buono G, Cusano M, Minucci M. Adjuvant effects of a crystalline silica on IgE and IgG1 antibody production in mice and their prevention by the macrophage stabilizer poly-2-vinylpyridine N-oxide. Int Arch Allergy Appl Immunol. 1983; 71:279–281.
49. Kuroda E, Ishii KJ, Uematsu S, Ohata K, Coban C, Akira S, et al. Silica crystals and aluminum salts regulate the production of prostaglandin in macrophages via NALP3 inflammasome-independent mechanisms. Immunity. 2011; 34:514–526.
50. Nygaard UC, Hansen JS, Samuelsen M, Alberg T, Marioara CD, Løvik M. Single-walled and multi-walled carbon nanotubes promote allergic immune responses in mice. Toxicol Sci. 2009; 109:113–123.
51. Inoue K, Koike E, Yanagisawa R, Hirano S, Nishikawa M, Takano H. Effects of multi-walled carbon nanotubes on a murine allergic airway inflammation model. Toxicol Appl Pharmacol. 2009; 237:306–316.
52. Gehring U, Wijga AH, Brauer M, Fischer P, de Jongste JC, Kerkhof M, et al. Traffic-related air pollution and the development of asthma and allergies during the first 8 years of life. Am J Respir Crit Care Med. 2010; 181:596–603.
53. Morgenstern V, Zutavern A, Cyrys J, Brockow I, Koletzko S, Krämer U, et al. Atopic diseases, allergic sensitization, and exposure to traffic-related air pollution in children. Am J Respir Crit Care Med. 2008; 177:1331–1337.
54. Brauer M, Hoek G, Smit HA, de Jongste JC, Gerritsen J, Postma DS, et al. Air pollution and development of asthma, allergy and infections in a birth cohort. Eur Respir J. 2007; 29:879–888.
55. Sanborn MD, Cole D, Abelsohn A, Weir E. Identifying and managing adverse environmental health effects: 4. Pesticides. CMAJ. 2002; 166:1431–1436.
56. O'Malley M. Clinical evaluation of pesticide exposure and poisonings. Lancet. 1997; 349:1161–1166.
57. Weselak M, Arbuckle TE, Wigle DT, Krewski D. In utero pesticide exposure and childhood morbidity. Environ Res. 2007; 103:79–86.
58. Smit LA, Zuurbier M, Doekes G, Wouters IM, Heederik D, Douwes J. Hay fever and asthma symptoms in conventional and organic farmers in The Netherlands. Occup Environ Med. 2007; 64:101–107.
59. Maestrelli P, Boschetto P, Fabbri LM, Mapp CE. Mechanisms of occupational asthma. J Allergy Clin Immunol. 2009; 123:531–542.