Abstract
Purpose
Asthma is a chronic respiratory disorder that leads to inflammation and narrowing of the airways. Its global prevalence has attained epidemic levels and treatment options that reach beyond temporary relief of symptoms are urgently needed. Since the processes leading to clinically symptomatic asthma start early in life, we set out to systematically evaluate a neonatal immunotherapeutic based on Listeria monocytogenes (Lm) for the control of allergic sensitization.
Methods
We modified Lm to express the model allergen, ovalbumin (OVA), and tested the ability of neonatal immunization with this strain to control allergic sensitization in a mouse model of OVA-induced asthma. Mice were immunized as newborns with live or heat killed LmOVA or live Lm, followed 6 weeks later by allergic sensitization with OVA. In order to determine whether the TH1-polarizing effect of this vaccine vector inadvertently may exacerbate development of certain TH1-driven allergic diseases, mice immunized as newborns were assessed in a model of adult hypersensitivity pneumonitis (HP).
Results
Both LmOVA and Lm-control vaccines were highly effective in providing long-lasting protection from airway inflammation after only one immunization given perinatally. Serum antibody levels and lung cytokine production suggest that this prophylactic strategy is associated with an allergen specific TH1-dominated response. Specifically, LmOVA vaccinated mice displayed significantly elevated OVA-specific serum IgG2a, but no difference in anti-OVA IgE antibodies and only slightly decreased anti-OVA IgG1 antibodies. Importantly, Lm-based neonatal vaccination did not exacerbate Th1/Th17 driven HP, arguing against broad spectrum immune skewing.
The global prevalence, morbidity, mortality and economic cost associated with asthma have been on the rise, increasing nearly 50% every decade.1,2 Asthma is most often first diagnosed in childhood and acute asthmatic responses are one of the most common causes of emergency room visits in children.3 Currently, most common treatment options provide only temporary relief to alleviate symptoms while sustained relief requires long-term interventions that include medication and lifestyle modifications. Part of the underlying mechanisms leading to asthma relate to immune mediated, Th2-biased allergen-specific responses4 and immunomodulatory approaches such as allergen-specific immunotherapy have been successfully employed.5,6 However, neonatal prophylactic vaccine-based strategies offer an even more effective approach that could lead to protection from asthma.6
The processes leading to clinically symptomatic asthma have been shown to begin early in life7,8 and therefore, attempts to prevent asthma would likely be most effective if initiated perinatally. Studies in human and animal models have identified the time around birth and early infancy as a period during which allergen-specific immune memory responses of pro-allergic Th2 versus non-allergic immune responses to environmental stimuli are made.8,9 The increased risk for the development of asthma and allergy in early life has been attributed to an apparent Th2-bias in the newborn's immune system,10,11 and the immune-mediated physiological parameters that lead to clinical asthma are known to be driven by an increased production of allergen-specific CD4 Th2 cytokines, in particular IL-4, IL-5, and IL-13. These cytokines have been shown to initiate and sustain, via immune memory, allergic asthmatic inflammatory responses by enhancing the production of IgE antibodies as well as the growth, differentiation and recruitment of pro-inflammatory cells to the lung tissue (e.g. eosinophils).10 In summary, a successful therapy aimed at altering the underlying cause of asthma would need to focus on modifying or reducing pro-inflammatory allergen-specific Th2 immune responses early in life.6
It is well established that whole heat-killed bacteria like Mycobacterium bovis, Chlamydia muridarum, Lm11,12 or bacterially-derived Toll-like receptor ligands13 when administered as adjuvants with model allergens can effectively prevent allergic sensitization and/or allergic inflammation in animal models.14,15 One of the most potent inducers of IFN-γ and other non-Th2 effector molecules is live Lm.16,17 Previous publications harnessing the benefits of Lm to modify hypersensitivity reactions have focused on the efficacy of heat-killed Lm (HKLm) in adult mice. In these studies, HKLm successfully suppressed allergen-specific, Th2-dominated responses by inducing allergen-specific Th1-dominated responses in adult mice.12,18 However, these studies have not evaluated the ability of Lm-based vaccines to modulate atopic diseases originating in early life. Additionally, live attenuated Lm may have higher potential than HKLm for preventing or treating allergic diseases. Thus, the novelty of our study is determining the efficacy of neonatal Lm vaccine. Our findings show that this approach can work in early life, which is a time point that is clinically important.
We show here that a single neonatal dose of a live attenuated Lm vaccine provides sustained protection from allergic airway inflammation in a murine model of asthma. And while this early life Lm-vaccination was associated with reduced Th2 responses, it did not lead to increased susceptibility to Th1/Th17-mediated hypersensitivity pneumonitis (HP), suggesting that Lm-based immune modulation early in life supports a balanced immune status.
For all experiments we used murine F1 pups (H-2b×H-2d) derived from crosses between C57BL/6 (H-2b) and C57B10.D2 (H-2d) mice provided from the Jackson Laboratories (Bar Harbor, ME,USA) to match our previous work on the development of our neonatal vaccine platform based on Lm Δ(trpS actA)/pSP0-PShlyOVA (LmOVA).19 F1 mice were vaccinated either 6 days post-partum (Fig. 1 and 4). All animals were maintained under pathogen-free conditions at the Child and Family Research Institute of the University of British Columbia according to animal experiment protocols approved by the Institutional Animal Care and Use Committee. When analyzing the asthma model, we did not detect any difference between males and females for any of the parameters tested, and thus data presented were derived from both genders combined unless specified. We did, however, detect a significantly higher response in female vs. male mice to Saccharopolyspora rectivirgula (SR)-antigen in the HP model for all parameters tested and thus data presented were obtained using female mice only in this model.
We employed our recently reported live and highly-attenuated Lm-based vaccine platform Lm Δ (trpS actA)/pSP0 that permits specific antigen expression and is particularly safe and well-tolerated in newborn mice.19 We have shown previously that it provides a strong and broad immune response against ovalbumin (OVA), including CD4 and CD8 T cell responses as well as humoral responses.19 Lm platform strains were grown to late logarithmic phase (optical density at 600 nm [OD600], 1.0) at 37℃ in Brain Heart Infusion (BHI) Medium (BD, MD, USA), washed and resuspended in endotoxin-free isotonic saline solution (0.9% NaCl) and stored in 20% glycerol at -80℃ prior to injection as described below.
Mice were immunized intraperitoneally (i.p.) with Lm, resuspended in 0.1 mL endotoxin-free 0.9% NaCl. HKLm were prepared by boiling 1×107 bacteria at 110℃ for 30 minutes. Bacterial viability and colony forming unit counts were determined by plating serial dilutions on BHI agar plates.
Based on the 2 models (allergic asthma, HP), we used 2 different antigens, (A) OVA to induce asthma and (B) SR to induce HP. To induce asthma, 6 weeks after immunization with Lm strains, mice were sensitized twice i.p. with 100 µg OVA (Worthington, Lakewood NJ, USA) absorbed onto alum hydroxide gel (Brenntag Biosector, Frederikssund, Denmark) - this marked day 1 and 8 in the experimental schedule (Fig. 1A). Non-immunized naive mice received alum hydroxide gel alone on the same schedule. Subsequently, anesthetized mice were challenged intranasally (i.n.) on days 22, 23, 24, 26, and 28 with 200 µg OVA in 100 µL PBS prior to sacrifice on day 29. Non-immunized naive mice were i.n. with saline only on the same schedule. The following abbreviations are used for the different experimental groups (Fig. 1): "Naïve" - immunization with NaCl without subsequent allergen exposure; "Ctrl" - immunization with NaCl followed by allergen exposure; "Lm" - immunization with Lm Δ(trpS actA)/pSP0, followed by allergen exposure; "LmOVA" - immunization with Lm Δ(trpS actA)/pSP0-PShlyOVA, followed by allergen exposure; "HKLmOVA" - immunization with HKLm Δ(trpS actA)/pSP0-PShly-OVA, followed by allergen exposure.
To induce HP, mice were immunized at day 6 after birth with Lm strains and 6 weeks later were primed i.n. and challenged with 40 µL of 4 mg/mL endotoxin-free SR antigen for 3 consecutive days per week for 3 weeks. Non-immunized naive mice were challenged i.n. with saline only. Mice were sacrificed four days after the last challenge. On the day of analysis for both experiments, bronchoalveolar lavages (BAL) were obtained by 3 sequential introductions and aspiration of 1 mL sterile PBS each. Total viable BAL cells were counted using trypan blue staining and differential counts were obtained from cytospin preparations stained with hematoxylin and eosin (H&E stain) according to the manufacturer's instructions (Hema 3® Stain Set, Fisher Scientific). For HP experiments, H&E results were confirmed using flow-cytometry based quantitation of neutrophils (7.4 antigen +), eosinophils (SiglecF+/CD11c-), marcophages (SiglecF+/CD11c+), T cells (CD3+) and B cells (B220+) using antibodies purchased from BD-Pharmingen (San Diego, CA, USA). In addition, blood was collected by cardiac puncture and lung tissue was harvested. Serum was stored at -20℃ until further analysis. The following abbreviations are used for the different experimental groups (Fig. 4): "Naive" - immunization with NaCl without SR exposure; "Ctrl" - immunization with NaCl followed by SR exposure; "Lm" - immunization with Lm Δ(trpS actA)/pSP0, followed by SR exposure.
The right lung lobes were harvested and prepared according to previously described protocols20: a total of 2.5×106 lung cells were cultured for 48 hours in 200 µL of complete medium R10 (RPMI 1640 supplemented with 10% FCS, streptomycin, penicillin) in the presence or absence of either OVA (500 µg/mL) (asthma experiments), or endotoxin-free SR (10 µg/mL and 100 µg/mL) (HP experiment). Supernatants were collected and stored at -80℃ until batch analysis for cytokine production was evaluated using a 6-Milliplex Mouse Cytokine/Chemokine Immunoassay according to manufacturer's instructions (Millipore Corporation, MA, USA).
Left lungs were inflated with 10% formalin and fixed for 24 hours. Following fixation, lungs were isolated and bisected as described previously.21
Histological evaluations were performed by 2 blinded investigators who scored lung sections using a 0 to 5 scale21,22 to rank the degree of cell infiltration and goblet cell metaplasia. A score of "0" indicated that there was no cell inflammation/goblet cell metaplasia while a score of "5" referred to the highest amount of cell infiltration/goblet cell metaplasia observed.
The following ELISA kits and sample dilutions were used to quantify antibody (Ab) levels in serum. The mouse anti-OVA IgG1 ELISA kit from BioVendor R&D was used at Ab dilutions of 1:100. The mouse anti-OVA IgG2a ELISA was performed using HRP Rat Anti-Mouse IgG2a antibodies from BD Pharmingen (San Diego, CA, USA) at Ab dilution of 1:1,000. The mouse anti-OVA IgE ELISA kit from Shibayagi was used at Ab dilutions of 1:20. Total IgE levels were determined with the ELISA MAX™ Deluxe Set provided by BioLegend (San Diego, CA, USA) at serum dilutions of 1:600, total IgG1 levels were checked on serum dilutions of 1:50,000 using anti-IgG1 ELISA kits provided by Bethyl Laboratories (Montgomery, TX, USA).
Airway responsiveness to methacholine (MCh) (Sigma, Oakville, ON, CANADA) challenges was determined using a constant phase model flow employed by the FlexiVent (SCIREQ Scientific Respiratory Equipment Inc., Montreal, QC, Canada). Mice were anesthetized with Avertin (Tribromoethanol, Sigma, Canada) (150 mg/kg, i.p.) and tracheotomized with a cannula ensuring a constant flow of air. Mice were given pancuronium bromide (Sigma, Oakville, ON, CANADA) (0.8 mg/kg, i.m.) to block spontaneous breathing. After baseline airway resistance stabilized (Rbase), increasing doses of MCh (62.5 µg/kg, 125 µg/kg, 250 µg/kg, 500 µg/kg, 1,000 µg/kg, 2,000 µg/kg) were infused via the jugular vein. Total respiratory system resistance was determined immediately before MCh infusion (Rmin), and then every 2 seconds following infusion until the maximum response was reached (Rmax). MCh-induced increases in resistance were calculated as follows: % increase in airway resistance=([Rmax-Rmin]/Rbase)×100. Significant differences in airway hyperresponsiveness (AHR) were determined using a one-way ANOVA with a Bonferroni's post-hoc test23 and visualized by establishing the area under the curve of the AHR response.24
The displayed results represent pooled data from four separate neonate asthma experiments (3-5 mice per group per experiment), and data from a single HP experiment (6-14 mice per group). The results are expressed as mean±standard error mean (SEM). Statistically significant differences across the asthma or HP groups were determined using ANOVA.
To test whether Lm-based vaccines could offer protection from allergic airway disease, neonatal mice were immunized on postnatal day 6 with either saline, the parental Lm platform strain, the Lm strain expressing ovalbumin (LmOVA) or heat killed LmOVA (HKLmOVA) as described in the Materials and Methods and previously.19 These neonatal vaccinated mice were then subjected to a well-described model of OVA-induced asthma25,26,27 as outlined in Fig. 1A. One day after the final instillation, the bronchoalveolar lavage fluid (BALF) from the murine airways was collected and evaluated for total cell numbers (Fig. 1B) as well as differential cell counts of infiltrates (Fig. 1C). Mice immunized with HKLmOVA as neonates, exhibited a similar degree of airway cell infiltration as the allergen exposed control group (Ctrl). Strikingly, however, mice immunized with live-attenuated OVA-expressing strain (LmOVA) exhibited significantly reduced total numbers of cells infiltrating the airways. Intriguingly, mice immunized with control Lm (lacking OVA) also exhibited a trend towards protection from allergen-induced infiltrates into the BALF, but this did not reach statistical significance (Fig. 1B).
Next, we investigated the type of inflammatory cells recruited to the BALF with particular attention to the types of cells that produce the wide array of pro-inflammatory mediators involved in asthma pathogenesis.28 Most strikingly, eosinophil levels exhibited significant differences between the control and vaccinated groups (Fig. 1C). While eosinophils were found in greatest abundance in the control and HKLmOVA group, mice from both the LmOVA and Lm groups exhibited significantly fewer eosinophils in BALF. We found no significant difference in the number of macrophages, lymphocytes or neutrophils between mice from any of the vaccine groups compared to mice from the negative or positive controls.
To further investigate alterations in the adaptive immune response resulting from Lm immunization, we investigated the levels of allergen-specific serum antibodies in the various test groups. Compared to naïve mice, all experimental groups subjected to OVA-based asthma induction exhibited elevated circulating anti-OVA IgE and anti-OVA IgG1 antibodies (Supplemental Fig. 1). However, only the LmOVA mice showed significantly elevated circulating levels of anti-OVA IgG2a antibody (Fig. 1D). Somewhat surprisingly, this was not at the expense of Th2-associated isotype production since all experimental groups exhibited similar levels of OVA-specific IgE and IgG1.
Asthma pathophysiology involves a variety of cells and a complex interaction of their pro-inflammatory mediators.29,30 We investigated the production of IL-4, IL-5, IL-13, IL-10, IL-12, TGF-β, IL-2, and IFN-γ following ex vivo OVA restimulation of cells isolated from lungs.31,32 IL-4, IL-13, IL-12, TGF-β, and IL-2 production in response to OVA-stimulation in any of the groups remained at the level of control mice (data not shown). IFN-γ production was, overall, very low (Fig. 2A) and while there was no significant difference between any of the vaccinated groups, it tended to be higher in the LmOVA group. IL-5 (Fig. 2B) on the other hand was produced at significantly lower levels in the Lm, LmOVA, and HKLmOVA groups in comparison to the control group and showed the highest statistical significance in the LmOVA groups. Lastly, following ex vivo OVA restimulation of lung resident cells, IL-10 was found to be produced at significantly lower levels selectively in the LmOVA vaccinated group, while production of IL-10 in other groups was unaltered (Fig. 2C). In summary, these data suggest that neonatal Lm immunization induces a significant dampening of Th2 inflammatory mediator production in the lungs of allergen challenged animals and that this response is further enhanced by immunization with Lm carrying OVA.
To evaluate additional pathologic hallmarks of asthma, we used the FlexiVent small animal ventilator with live, intubated, and anesthetized mice to directly measure airway resistance in response to a non-specific stimulus methacholine (MCh), in an attempt to determine overall AHR.21,33 We omitted the HKLmOVA group since we failed to observe reduce inflammatory infiltrates in the BALF of this group (see above), and thus were unlikely to observe protection from AHR with MCh challenges. Dose-response curves of increasing MCh challenges revealed that at the highest dose of 2 mg/kg, the control group responded with a far greater AHR than the "Naïve" group (Fig. 3A). Indeed, the allergic control group was the only cohort that exhibited statistically significant hyper-responsiveness above the naïve controls. To highlight the dose-response relationships between the experimental vaccine groups, we calculated the area under the curve of these responses (Fig. 3B). This revealed that both, LmOVA as well as the non-allergen-specific Lm groups exhibited reductions in AHR following MCh challenge. Thus, our data suggest that neonatal immunization with Lm ameliorates this second major hallmark of allergic inflammation.
Given that neonatal Lm-vaccination non-specifically (i.e. non-OVA allergen-specific) reduced allergen-induced eosinophil numbers in the BALF, lowered IL-5 production from lung-resident cells and dampened AHR following the MCh challenge. We considered the possibility that neonatal Lm vaccination may lead to broad spectrum immune modulation away from a Th2 response and enhance susceptibility to Th1/17 driven disease. To this end, we investigated the effect of neonatal Lm-immunization on susceptibility to SR-induced HP, a classic model for Th1/17 dependent pulmonary disease34 (Fig. 4A). Thus, neonatally vaccinated mice were primed and challenged with SR antigen intranasally 3 times per week for 3 consecutive weeks. They were then evaluated for BALF infiltrates, and histological parameters associated with HP. The results are shown in Fig. 4. We were unable to detect a significant alteration in cellular - and in particular lymphocytic - infiltration into the BALF, airways, pulmonary vessels or parenchyma following neonatal Lm-immunization and subsequent SR-induced HP (Fig. 4B-F and Supplemental Fig. 3). Correspondingly, anti-SR serum IgG1 and IgG2a antibody levels were unaltered by neonatal Lm-immunization (Supplemental Fig. 2). From these experiments, we conclude that although neonatal immunization with Lm protects against subsequent Th2 mediated allergic disease, it does not broadly increase the sensitivity to Th1/Th17-driven lung inflammatory disease.
We show here that a single perinatal immunization with attenuated Lm is effective at prophylactically reducing the impact of asthma sensitization in a murine model. Importantly, while neonatal Lm-based vaccination appears to have allergen-specific as well as non-specific components contributing to its protective effect, it does not increase the risk for Th1/Th17 mediated HP. The efficacy and safety of neonatal administration of Lm observed here support further exploration of early life immune modulation using Lm as a preventive strategy for allergic asthma and potentially other Th2-driven allergic disease.
Both genetic and environmental factors contribute to the development of allergic asthma35; however, the precise molecular underpinnings at the intersection of host and environment that predispose to the development of clinically apparent asthma are still largely obscure. In the absence of detailed mechanistic insight, empirical interventions continue to represent the main approach to slowing this growing epidemic. We therefore set out to investigate live, virulence-attenuated Lm as an immune-modulatory vehicle to redirect the inflammatory immune trajectory of atopic asthma.
Atopic hypersensitivity reactions such as asthma often originate early in life,7,8 therefore, attempts to prevent asthma would likely be most effective if initiated perinatally. We found that newborn mice vaccinated with live LmOVA and then challenged with OVA as adults exhibited a significant reduction in the total number of cells infiltrating the bronchial airways (Fig. 1). In particular, we observed a significant reduction in BALF infiltrating eosinophils, which are known to be major contributors to asthma-induced pathological changes in lung structure and function.36,37 However, neonatal mice that were immunized with HKLmOVA, exhibited a similar degree of airway cell infiltration as the Ctrl group (the group that was sensitized and challenged with OVA in the absence of Listerial infection), arguing that HKLm does not alter the total number or type of inflammatory cells recruited to the BALF in response to OVA-induced asthma. IL-5 is known to be the principal cytokine involved in eosinophilopoiesis. LmOVA vaccinated mice were also observed to produce less of this cytokine upon ex vivo restimulation of resident lung inflammatory cells (Fig. 2). This, however, is not sufficient to fully explain the protection conferred by LmOVA vaccination since similar reductions in IL-5 production were observed in Lm and HKLmOVA vaccinated mice, which exhibited a more modest or no reduction in eosinophil recruitment, respectively (Fig. 1 and 2). An additional paradox is the diminished production of IL-10 selectively from the lungs of mice receiving LmOVA vaccinations (Fig. 2). IL-10 is typically thought to act as a suppressor of inflammation and therefore one might expect a decrease would result in enhanced, rather than reduced inflammation. Furthermore, the trend towards increased IFN-γ production and reduced IL-10 production in the LmOVA group further support the notion that these mice are skewed towards a more mixed immune response to what would typically be a potent Th2-eliciting immune regimen. More detailed analysis of the exact mechanisms of immune modulation by Listeria-based vaccination is warranted, particularly the assessment of allergen-specific cytokine production by cells from mediastinal lymph nodes. In addition, it has been shown that alveolar macrophages produce a significantly higher level of IL-17 in OVA-sensitized mice compared to naïve mice. Thus, it will be interesting to investigate how Listeria-based vaccination affects IL-17 production from the alveolar macrophages in vaccinated OVA-sensitized mice.
In summary, these data suggest that LmOVA vaccination, and to a lesser extent Lm vaccination, dampen eosinophilic inflammation in a manner that does not correlate with a strict shift from a Th2 to a Th1 immune response or with a general dampening of all inflammatory responses. These observations are born out further by an analysis of antibody production in vaccinated mice. While there is normally a strong correlation between allergen-specific serum IgE levels and asthma in humans and mouse models of Th2 airway disease, mice in our LmOVA group displayed no difference in the level of anti-OVA IgE or IgG1 antibodies, but instead harboured significantly elevated anti-OVA IgG2a38 (Supplementary Fig. 1 and 2). Although the full significance of the increased OVA-specific IgG2a in the face of unchanged IgE and IgG1 levels remains to be clarified, these data suggest that perinatal LmOVA vaccination leads to a more mixed (or perhaps, more balanced) immune response to what is, normally, a highly Th2-polarizing inflammatory insult. Thus, the mechanism by which Lm inhibits allergic inflammation remains to be clarified.
To further illuminate the immunological impact of early-life Lm vaccination on future disease, we tested a similar cohort of mice for their susceptibility to the Th1/Th17-driven lung inflammatory disease, HP (Fig. 4). Here we saw no significant exacerbation or protection from disease in the vaccinated groups using a variety of parameters. The fact that neither systemic parameters (serological response to the SR antigen), nor pulmonary histological assessment in our study indicated a worsening of HP in neonatally vaccinated mice suggests, again, that early life Lm immunization appears to induce a balanced immune response, rather than a simple Th2/Th1 shift.
It is important to note that previous human association studies examining the prevalence of asthma as a function of specific microbial exposures in early life found a reduction in subjects exposed to microbes, including Lm.39,40 Likewise, additional studies in mice have suggested that perinatal, and in some instances prenatal, exposure to bacteria or antibiotic-induced shifts in host flora can have protective or exacerbating effects on future susceptibility to allergic airways disease, respectively.41,42,43
Previous studies in adult mice have shown that HKLm immunization is a strong nonspecific activator of IL-12 and IFN-γ production which can bias the immune system toward a Th1 immune response.18 HKLm immunization also reduces antigen-specific IL-4 production in lymph-node cells, reduces antigen-specific IgE production and enhances protective immune responses in the respiratory tract.12 However, the ability of Lm-based vaccines to modulate atopic diseases originating in early life has not been evaluated in these studies. Asthma has been shown to begin early in life7,8; therefore, asthma prevention would likely be most effective if initiated perinatally.
Since exposure to HKLm did not prevent airway inflammation in our model as it did in previous studies, the data would argue that mere exposure to Listerial components is insufficient to change the trajectory of atopic diseases, and that asthma prevention requires the more robust response generated in response to live Lm. It is unclear why HKLmOVA did not confer protection in our experimental model despite its positive outcomes observed by other research groups.12,18,44 Different mouse strains or protocols used to prepare HKLm could potentially explain these opposing findings.
In previous studies, the hygiene hypothesis has been postulated as a mechanistic explanation for asthma susceptibility. According to this view, allergy and asthma have become an "epidemic in the absence of infection" or perhaps more correctly, in the absence of perinatal colonization with a broad spectrum of bacteria.45 Although the mechanisms are still unknown regarding which microbial stimuli - such as Lm - could possibly protect children from developing asthma and atopy, changes of the innate immune system likely play a role in mediating immune responses away from allergic responses.14,46,47,48 Our study shows that early life exposure to a single live-attenuated bacterial strain can reduce susceptibility to asthma. This sets the framework to test multiple hypotheses about the mechanism of immune skewing towards or away from the development of asthma and other atopic diseases in early life. The many available tools in the Lm-based experimental system will permit detailed dissection of molecular mechanisms underlying Lm's ability to prevent allergic airway inflammation. These efforts will open the door to development of rationally targeted specific therapeutic interventions mimicking the effect of our live Lm vector.
Figures and Tables
Fig. 1
Vaccination with Lm and LmOVA significantly decreases Th2 driven allergic airway inflammation. (A) Schematic of Lm vaccination and experimental allergic airway inflammation protocol. (B) Total cell counts in BALF from naïve and OVA challenged mice. Naïve=saline vaccination and no subsequent OVA challenge, Ctrl=saline vaccination and OVA challenge, Lm=Listeria vaccination and OVA challenge, LmOVA=Listeria-OVA vaccination and OVA challenge, HKLmOVA=heat-killed LmOVA vaccination followed by OVA challenge. (C) Differential cell counts in BALF. (D) Relative concentration of OVA-specific IgG2a. Serum was diluted 1:1,000 and then serial dilutions were evaluated for OVA-specific antibody reactivity by ELISA as described in Materials and Methods. Values are expressed as mean±SEM. (**P value≤0.01, ***P value≤0.001). The number of mice per group per experiment was 3-5.
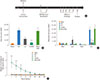
Fig. 2
Inflammatory lung cell cytokine responses are shifted by neonatal prophylactic LmOVA vaccination. Single cell suspensions were prepared from lungs, placed in culture and cytokine production was examined 48 hours after restimulation using 500 µg/mL of OVA. Cytokine production was determined by the 6-Milliplex Mouse Cytokine/Chemokine Immunoassay. (A) IFN-γ production. (B) IL-5 production. (C) IL-10 production. Values are expressed as mean±SEM. (*P value ≤0.05, ***P value ≤0.001). The number of mice per group per experiment was 3-5.
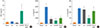
Fig. 3
Neonatal prophylactic immunization with Lm and LmOVA prevents significant increase in airway hyperresponsiveness (AHR) to methacholine challenge. (A) Mice were immunized and challenged as described in Fig. 1 and administered increasing doses of MCh intravenously. Airway resistance (R % increase) was measured for each dose of MCh. (B) Data from (A) re-evaluated as area under the curve. Values are expressed as mean±SEM. The number of mice per group was 8.
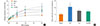
Fig. 4
Neonatal Lm vaccination does not significantly alter sensitivity to Th1/17 driven Hypersensitivity Pneumonitis (HP). (A) Schematic of vaccination and Saccharopolyspora rectivirgula (SR)-induced HP induction protocol. (B) Total cell counts in BALF from naïve and SR-challenged mice were determined as shown in Fig. 1. (C) Differential cell counts in BALF in response to HP induction. (D), (E), (F) Clinical scores for inflammatory infiltrates attributed to airways, vessels, and lung parenchyma respectively. Lungs were fixed and stained with H&E prior to blind scoring as described in Materials and Methods. Values are expressed as mean±SEM, The number of mice per group was 6-14.
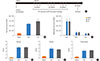
ACKNOWLEDGMENTS
This work was supported by a Canadian Institutes of Health Research (CIHR) Catalyst Grant and an AllerGen Network of Centres of Excellence operating grant (both to T.R.K. and K.M.M.). T.R.K. is also supported in part by a Career Award in the Biomedical Sciences from the Burroughs Wellcome Fund and a Michael Smith Foundation for Health Research Career Investigator Award. K.M.M. is a Michael Smith Foundation for Health Research Senior Scholar.
We thank the CFRI Animal Facility for expert technical assistance and animal care and Lisa Xu for outstanding flow cytometry assistance. We thank the Saudi Arabian Cultural Bureau for support of Sheka Y. Aloyouni.
References
1. Asher MI, Montefort S, Björkstén B, Lai CK, Strachan DP, Weiland SK, Williams H. ISAAC Phase Three Study Group. Worldwide time trends in the prevalence of symptoms of asthma, allergic rhinoconjunctivitis, and eczema in childhood: ISAAC Phases One and Three repeat multicountry cross-sectional surveys. Lancet. 2006; 368:733–743.
2. Valenta R. The future of antigen-specific immunotherapy of allergy. Nat Rev Immunol. 2002; 2:446–453.
3. [Asthma: facts and statistics]. J Pharm Belg. 1997; 52:127–128.
4. Wenzel SE. Asthma phenotypes: the evolution from clinical to molecular approaches. Nat Med. 2012; 18:716–725.
5. Pipet A, Botturi K, Pinot D, Vervloet D, Magnan A. Allergen-specific immunotherapy in allergic rhinitis and asthma. Mechanisms and proof of efficacy. Respir Med. 2009; 103:800–812.
6. Valenta R, Campana R, Marth K, van Hage M. Allergen-specific immunotherapy: from therapeutic vaccines to prophylactic approaches. J Intern Med. 2012; 272:144–157.
7. Hammad H, Lambrecht BN. Dendritic cells and epithelial cells: linking innate and adaptive immunity in asthma. Nat Rev Immunol. 2008; 8:193–204.
8. Tantisira KG, Weiss ST. Childhood infections and asthma: at the crossroads of the hygiene and Barker hypotheses. Respir Res. 2001; 2:324–327.
9. Garn H, Renz H. Epidemiological and immunological evidence for the hygiene hypothesis. Immunobiology. 2007; 212:441–452.
10. Barnes PJ. Th2 cytokines and asthma: an introduction. Respir Res. 2001; 2:64–65.
11. Tükenmez F, Bahçeciler NN, Barlan IB, Başaran MM. Effect of pre-immunization by killed Mycobacterium bovis and vaccae on immunoglobulin E response in ovalbumin-sensitized newborn mice. Pediatr Allergy Immunol. 1999; 10:107–111.
12. Hansen G, Yeung VP, Berry G, Umetsu DT, DeKruyff RH. Vaccination with heat-killed Listeria as adjuvant reverses established allergen-induced airway hyperreactivity and inflammation: role of CD8+ T cells and IL-18. J Immunol. 2000; 164:223–230.
13. Trujillo C, Erb KJ. Inhibition of allergic disorders by infection with bacteria or the exposure to bacterial products. Int J Med Microbiol. 2003; 293:123–131.
14. Jiao L, Han X, Wang S, Fan Y, Yang M, Qiu H, Yang X. Imprinted DC mediate the immune-educating effect of early-life microbial exposure. Eur J Immunol. 2009; 39:469–480.
15. Smits HH, Hammad H, van Nimwegen M, Soullie T, Willart MA, Lievers E, Kadouch J, Kool M, Kos-van Oosterhoud J, Deelder AM, Lambrecht BN, Yazdanbakhsh M. Protective effect of Schistosoma mansoni infection on allergic airway inflammation depends on the intensity and chronicity of infection. J Allergy Clin Immunol. 2007; 120:932–940.
16. Mizuki D, Miura T, Sasaki S, Mizuki M, Madarame H, Nakane A. Interference between host resistance to Listeria monocytogenes infection and ovalbumin-induced allergic responses in mice. Infect Immun. 2001; 69:1883–1888.
17. Bielecki J, Youngman P, Connelly P, Portnoy DA. Bacillus subtilis expressing a haemolysin gene from Listeria monocytogenes can grow in mammalian cells. Nature. 1990; 345:175–176.
18. Yeung VP, Gieni RS, Umetsu DT, DeKruyff RH. Heat-killed Listeria monocytogenes as an adjuvant converts established murine Th2-dominated immune responses into Th1-dominated responses. J Immunol. 1998; 161:4146–4152.
19. Loeffler DI, Smolen K, Aplin L, Cai B, Kollmann TR. Fine-tuning the safety and immunogenicity of Listeria monocytogenes-based neonatal vaccine platforms. Vaccine. 2009; 27:919–927.
20. Sauer KA, Scholtes P, Karwot R, Finotto S. Isolation of CD4+ T cells from murine lungs: a method to analyze ongoing immune responses in the lung. Nat Protoc. 2006; 1:2870–2875.
21. Hirota JA, Ellis R, Inman MD. Regional differences in the pattern of airway remodeling following chronic allergen exposure in mice. Respir Res. 2006; 7:120.
22. Shalaby KH, Gold LG, Schuessler TF, Martin JG, Robichaud A. Combined forced oscillation and forced expiration measurements in mice for the assessment of airway hyperresponsiveness. Respir Res. 2010; 11:82.
23. Vanoirbeek JA, Rinaldi M, De Vooght V, Haenen S, Bobic S, Gayan-Ramirez G, Hoet PH, Verbeken E, Decramer M, Nemery B, Janssens W. Noninvasive and invasive pulmonary function in mouse models of obstructive and restrictive respiratory diseases. Am J Respir Cell Mol Biol. 2010; 42:96–104.
24. Kollmann TR, Reikie B, Blimkie D, Way SS, Hajjar AM, Arispe K, Shaulov A, Wilson CB. Induction of protective immunity to Listeria monocytogenes in neonates. J Immunol. 2007; 178:3695–3701.
25. Blanchet MR, Bennett JL, Gold MJ, Levantini E, Tenen DG, Girard M, Cormier Y, McNagny KM. CD34 is required for dendritic cell trafficking and pathology in murine hypersensitivity pneumonitis. Am J Respir Crit Care Med. 2011; 184:687–698.
26. Blanchet MR, Maltby S, Haddon DJ, Merkens H, Zbytnuik L, McNagny KM. CD34 facilitates the development of allergic asthma. Blood. 2007; 110:2005–2012.
27. Lazarus SC. Inflammation, inflammatory mediators, and mediator antagonists in asthma. J Clin Pharmacol. 1998; 38:577–582.
28. Barnes PJ. The cytokine network in asthma and chronic obstructive pulmonary disease. J Clin Invest. 2008; 118:3546–3556.
29. Chung KF, Barnes PJ. Cytokines in asthma. Thorax. 1999; 54:825–857.
30. Conlan JW. Early pathogenesis of Listeria monocytogenes infection in the mouse spleen. J Med Microbiol. 1996; 44:295–302.
31. Flesch IE, Wandersee A, Kaufmann SH. Effects of IL-13 on murine listeriosis. Int Immunol. 1997; 9:467–474.
32. Tomioka S, Bates JH, Irvin CG. Airway and tissue mechanics in a murine model of asthma: alveolar capsule vs forced oscillations. J Appl Physiol (1985). 2002; 93:263–270.
33. Girard M, Israël-Assayag E, Cormier Y. Pathogenesis of hypersensitivity pneumonitis. Curr Opin Allergy Clin Immunol. 2004; 4:93–98.
34. Mukherjee AB, Zhang Z. Allergic asthma: influence of genetic and environmental factors. J Biol Chem. 2011; 286:32883–32889.
35. Lee JJ, Dimina D, Macias MP, Ochkur SI, McGarry MP, O'Neill KR, Protheroe C, Pero R, Nguyen T, Cormier SA, Lenkiewicz E, Colbert D, Rinaldi L, Ackerman SJ, Irvin CG, Lee NA. Defining a link with asthma in mice congenitally deficient in eosinophils. Science. 2004; 305:1773–1776.
36. Humbles AA, Lloyd CM, McMillan SJ, Friend DS, Xanthou G, McKenna EE, Ghiran S, Gerard NP, Yu C, Orkin SH, Gerard C. A critical role for eosinophils in allergic airways remodeling. Science. 2004; 305:1776–1779.
37. Song C, Luo L, Lei Z, Li B, Liang Z, Liu G, Li D, Zhang G, Huang B, Feng ZH. IL-17-producing alveolar macrophages mediate allergic lung inflammation related to asthma. J Immunol. 2008; 181:6117–6124.
38. Sung S, Rose CE, Fu SM. Intratracheal priming with ovalbumin- and ovalbumin 323-339 peptide-pulsed dendritic cells induces airway hyperresponsiveness, lung eosinophilia, goblet cell hyperplasia, and inflammation. J Immunol. 2001; 166:1261–1271.
39. Ege MJ, Mayer M, Normand AC, Genuneit J, Cookson WO, Braun-Fahrländer C, Heederik D, Piarroux R, von Mutius E. GABRIELA Transregio 22 Study Group. Exposure to environmental microorganisms and childhood asthma. N Engl J Med. 2011; 364:701–709.
40. Riedler J, Braun-Fahrländer C, Eder W, Schreuer M, Waser M, Maisch S, Carr D, Schierl R, Nowak D, von Mutius E. ALEX Study Team. Exposure to farming in early life and development of asthma and allergy: a cross-sectional survey. Lancet. 2001; 358:1129–1133.
41. Conrad ML, Ferstl R, Teich R, Brand S, Blümer N, Yildirim AO, Patrascan CC, Hanuszkiewicz A, Akira S, Wagner H, Holst O, von Mutius E, Pfefferle PI, Kirschning CJ, Garn H, Renz H. Maternal TLR signaling is required for prenatal asthma protection by the nonpathogenic microbe Acinetobacter lwoffii F78. J Exp Med. 2009; 206:2869–2877.
42. Russell SL, Gold MJ, Hartmann M, Willing BP, Thorson L, Wlodarska M, Gill N, Blanchet MR, Mohn WW, McNagny KM, Finlay BB. Early life antibiotic-driven changes in microbiota enhance susceptibility to allergic asthma. EMBO Rep. 2012; 13:440–447.
43. Hill DA, Siracusa MC, Abt MC, Kim BS, Kobuley D, Kubo M, Kambayashi T, Larosa DF, Renner ED, Orange JS, Bushman FD, Artis D. Commensal bacteria-derived signals regulate basophil hematopoiesis and allergic inflammation. Nat Med. 2012; 18:538–546.
44. Frick OL, Teuber SS, Buchanan BB, Morigasaki S, Umetsu DT. Allergen immunotherapy with heat-killed Listeria monocytogenes alleviates peanut and food-induced anaphylaxis in dogs. Allergy. 2005; 60:243–250.
45. Cookson WO, Moffatt MF. Asthma: an epidemic in the absence of infection? Science. 1997; 275:41–42.
46. Han X, Fan Y, Wang S, Yang J, Bilenki L, Qiu H, Jiao L, Yang X. Dendritic cells from Chlamydia-infected mice show altered Toll-like receptor expression and play a crucial role in inhibition of allergic responses to ovalbumin. Eur J Immunol. 2004; 34:981–989.
47. Dietert RR, Piepenbrink MS. The managed immune system: protecting the womb to delay the tomb. Hum Exp Toxicol. 2008; 27:129–134.
48. Holtzman MJ. Asthma as a chronic disease of the innate and adaptive immune systems responding to viruses and allergens. J Clin Invest. 2012; 122:2741–2748.
Supplementary Material
Supplemental Fig. 1
Neonatal prophylactic LmOVA immunization does not change OVA-specific IgE or IgG1 titers. Antibody (Ab) titers to OVA were evaluated by ELISA of diluted serum from the indicated groups as described in the Materials and Methods. Values are expressed as mean±SEM. The number of mice per group per experiment was 3-5.
Supplemental Fig. 2
Neonatal Lm vaccination did not change the IgG2a or IgG1 SR-specific serum Ab levels. Anti-SR antigen titers in diluted serum from the indicated groups were determined by ELISA as described in the Materials and Methods. Values are expressed as mean±SEM. The number of mice per group was 6-14.
Supplemental Fig. 3
Neonatal Lm vaccination did not alter HP-induced cellular infiltration into airways, vessels, or parenchyma. HP was induced in experimental groups as described. Left lobes of the lungs were formalin fixed, bisected, and sectioned. Histological sections were evaluated for the presence of mononuclear cell infiltration around the airways, vessels and parenchyma and scored according to the scheme described in the Materials and Methods. The number of mice per group was 6-14.