Abstract
Purpose
Asthma is a chronic inflammatory disease of the airways associated with structural changes and airway remodeling. Interleukin (IL)-9 has pleiotropic effects on both inflammatory cells and airway structural cells, which are involved in asthma pathogenesis. We evaluated the effects of IL-9 blockade on chronic airway inflammation.
Methods
Acute airway inflammation was induced in Balb/c mice using aerosolized ovalbumin (OVA), whereas chronic asthma was induced by OVA exposure for 5 weeks with anti-IL-9 or isotype-matched antibody (Ab) treatment during the OVA challenge. Inflammatory cells in bronchoalveolar lavage fluid (BALF) were counted and lung tissues were stained to detect cellular infiltration, mucus deposition, and collagen accumulation. The levels of interferon (IFN)-γ, IL-4, IL-5, IL-9, IL-17, and immunoglobulin E (IgE) in BALF were measured using enzyme linked immunosorbent assays, and profiles of inflammatory cells and subsets of T helper (Th) cells were analyzed using flow cytometry.
Results
IL-9, IL-17, and IFN-γ levels were significantly increased in the chronic group compared to the acute asthma group. However, the number of IL-9-positive cells was not affected, with a decrease in Th17 cells in OVA-challenged caspase-1 knockout mice. Numbers of eosinophils, neutrophils, B cells, mast cells, and Th17 cells decreased after administration of anti-IL-9 Ab. Total IgE, IL-5, IL-9, and IL-17 levels were also lower in the anti-IL-9 group.
Asthma is a chronic inflammatory disease of the airways characterized by airway hyperresponsiveness (AHR) and varying degrees of airflow limitation accompanied by cough, dyspnea, and wheezing. These symptoms are often reversible either spontaneously or with treatment.1 Although most patients' asthma can be controlled with inhaled corticosteroids, some patients are refractory to treatments due to airway remodeling. The histological characteristics of airway remodeling include epithelial denudation, hyperplasia of goblet cells and mucus glands, subepithelial fibrosis, and hypertrophy and hyperplasia of airway smooth muscle. Airway remodeling is thought to be a result of chronic inflammation and is induced by various inflammatory and airway structural cells, in addition to chemical mediators.1-4
Although many studies have suggested that inhaled corticosteroids suppress chronic airway inflammation and airway remodeling, this remains unclear.4 Previous studies have shown that airway remodeling occurs in parallel with inflammation in pediatric patients with asthma and suggests limitations of inhaled corticosteroids.5 For these reasons, therapeutic agents other than corticosteroids, such as cytokine inhibitors, have been developed to suppress airway remodeling.
Asthma pathogenesis is primarily related to T helper type 2 (Th2) cells and their associated cytokines, namely, interleukin (IL)-4, IL-5, and IL-13. Th17 cells and regulatory T (Treg) cells, as well as their cytokines, are correlated with the development of asthma. We previously reported that IL-33 induces Th17-mediated airway inflammation via mast cells in ovalbumin (OVA)-challenged mice.6 Asthma is thought to develop due to an imbalance between Th17 and Treg cells.7,8 Many studies have explored therapeutic agents for asthma such as antibodies (Ab) to IL-5 and IL-4/IL-13 and tumor necrosis factor (TNF)-α inhibitors.8-12 However, anti-IL-5 Abs are only effective in patients with steroid-dependent or steroid-resistant eosinophilic asthma, and TNF-α inhibitors sometimes produce adverse drug effects. Because of this variation in efficacy and the potential for deleterious effects, further studies are required to develop novel therapeutic agents.
IL-9, which was first described in 1980, triggers mast cells and lymphocytes to release chemical mediators and accelerate cell growth and survival. IL-9 is thought to be a Th2 cytokine because of its ability to induce the Th2-biased immune response.13 When Th2 cells or naïve T cells are simultaneously exposed to IL-4 and transforming growth factor (TGF)-β, they undergo phenotype switching driven by transcription factor PU.1 and interferon regulatory factor 4 (IRF4) into IL-9-producing cells (Th9 cells).14 Populations of Th cells other than Th9 cells are also known to produce IL-9 in addition to their own cytokines. Th2 cells produce IL-4, IL-5, and IL-13 in addition to IL-9, and Th17 cells produce interferon (IFN)-γ, IL-17, and IL-9. Although mouse Th9 cells produce both IL-9 and IL-10, human Th9 cells only produce IL-9.15,16 Th9 cells also accelerate chemokine production in airway smooth muscles and epithelial cells, both of which are closely associated with airway remodeling. Previous studies have revealed a close correlation between IL-9 and AHR,17-19 but the relationship between IL-9 and the induction of Th1 or Th17 subsets in allergic asthma remains unclear. In the present study, we examined the relationships between IL-9 and chronic asthma inflammation and investigated whether IL-9 blockade has significant effects on the induction of IL-17-producing cells and airway remodeling in a mouse model of asthma.
Eight-week-old female Balb/c mice and C57BL/6 mice were purchased from Daehan Biolink (Eumseong, Korea). Caspase-1 knockout (KO) mice were generously provided by Dr. Sutterwala from the University of Iowa (Iowa City, IA, USA). Mice were housed in the animal care facility with food and water available ad libitum. Mice were exposed to a 12:12 hours light:dark cycle in room air at room temperature (RT). All procedures were approved by the Animal Care and Use Committee of the Ewha Womans University School of Medicine (ESM 11-0164).
Mice (n=15) were immunized via intraperitoneal injection on days 0 and 5 with 10 µg OVA Grade II (Sigma, St. Louis, MO, USA) complexes with 1 mg aluminum hydroxide (Sigma) at a volume of 0.2 mL per mouse. Using a nebulizer (Medel, Vlaeberg, South Africa), mice were exposed for 30 minutes per day to an aerosol of either 1% OVA in phosphate-buffered saline (PBS) or PBS only on days 12-15 post-immunization. Mice were sacrificed 24 hours after the final aerosol challenge to collect bronchoalveolar lavage fluid (BALF) and lung tissues.
Mice (n=10) were immunized via intraperitoneal injection on days 0 and 12 with 10 µg OVA complex with 1 mg aluminum hydroxide at a volume of 0.2 mL per mouse. Using a nebulizer, mice were exposed to 1% OVA in PBS on days 18-23 for 30 minutes per day. After day 26, mice were exposed to aerosolized 1% OVA three times per week for 5 weeks. Anti-IL-9 Ab (n=5) or control isotype immunoglobulin G (Iso IgG) (n=5) were administered intraperitoneally (100 µg/mouse) once a week during the OVA challenge. A schematic of this protocol is shown in Fig. 1.
After the mice were anesthetized with a mixture of Zoletil® (30 mg/kg, Virbac Laboratories, France) and Rompun® (10 mg/kg, Bayer AG, Germany), the tracheas were cannulated. BAL was subsequently conducted with the instillation of 4×0.5 mL PBS into the lungs, along with gentle massaging to maximize cell recovery. BAL cells were pelleted via centrifugation and counted with a hemocytometer. Differential leukocyte counts were obtained on cytospin slide preparations after Giemsa staining.
Lung tissues were fixed in 4% paraformaldehyde and embedded in paraffin. Fixed tissues were cut into 5 µm sections, placed on glass slides, and de-paraffinized. Sections were stained with hematoxylin and eosin (H&E) to detect cellular infiltration, with periodic acid-Schiff (PAS) to detect mucus deposition, and with Sirius Red stain to observe collagen accumulation. The degree of peripheral inflammatory cell infiltration was rated on a 4-point scale: 0, normal; 1, fewer cells, 2, a ring of inflammatory cells up to four cells deep; and 3, a ring of inflammatory cells more than four cells deep.20 Tissue sections from each mouse were measured using image analysis software (analySIS FIVE, Olympus Corporation, Japan) to analyze the deposition of mucus and collagen.
Enzyme-linked immunosorbent assays (ELISAs) were performed to measure IFN-γ, IL-4, and IL-5 with BD OptEIA™ (BD Biosciences, San Diego, CA, USA). IL-9, IL-17, and IgE concentrations in BALF were measured using a commercially available kit from BioLegend (San Diego, CA, USA). Wells were coated with 100 µL capture Ab in a coating buffer (0.1 M sodium carbonate, pH 9.5) and plates were incubated overnight at 4℃. Wells were rinsed with a washing buffer (PBS with 0.05% Tween-20) and reactions were stopped with assay diluent (10% fetal bovine serum in PBS) for 1 hour at RT. After the supernatant was obtained by BALF centrifugation, 100 µL supernatant was put in each well and incubated for 2 hours at RT. The supernatant was removed, the wells were washed, and 100 µL detection Ab and streptavidin-conjugated horse radish peroxidase (HRP) reagent was added and reacted for 1 hour at RT. After the reaction, the wells were washed, 100 µL substance solution (tetramethylbenzidine and hydrogen peroxide, BD Biosciences) was added to each well, and the plates were incubated in the dark for 30 minutes at RT. The absorbance was measured at 450 nm within 30 minutes of adding the stop solution (2N H2SO4).
To compare cellular profiles after the induction of airway inflammation, BAL cells from mice challenged with OVA with or without anti-IL-9 Ab were stained and analyzed on a FACSCalibur system (Becton Dickinson, Franklin Lakes, NJ, USA). The Abs used for flow cytometry were as follows: FITC-anti-mouse CD49d (9C10, BD Biosciences), FITC-anti-mouse Ly-6G/Ly-6C (Gr-1; RB6-8C5, BioLegend), PE-anti-mouse FcεRIα (MAR-1, BioLegend), APC-anti-mouse CD117 (c-kit; ACK2, BioLegend), FITC-anti-mouse CD49b (HMα2, BioLegend), FITC-anti-mouse CD19 (6D5, BioLegend), anti-mouse CD4 (GK1.5, BioLegend), APC-anti-mouse IFN-γ (XGM1.2, BioLegend), anti-mouse IL-4 (11B11, BD Bioscience), anti-mouse IL-9 (RM9A4, BioLegend), anti-mouse IL-17A (TC11-18H10.1, BioLegend), and Alexa Fluor®488-anti-mouse FoxP3 (206D, BioLegend).
BAL cells were lysed in a lysis buffer (1% Triton X-100, 150 mM NaCl, 20 mM Tris, pH 7.5) with protease inhibitors, and 10 µg protein was separated using 12% sodium dodecyl sulfate polyacrylamide gel electrophoresis (SDS-PAGE). The resolved proteins were transferred to polyvinylidene fluoride membranes (Millipore, Bedford, MA, USA). Membranes were blocked with 5% skim milk in Tris-buffered saline and incubated with the following primary Abs: anti-IL-1β (#2022, Cell Signaling Technology, Danvers, MA, USA), anti-β-actin (AC-15, mouse monoclonal, Abcam, Cambridge, UK), and anti-PU.1 (#2266, Cell Signaling Technology). After washing, the membranes were incubated with HRP-conjugated anti-mouse or anti-rabbit IgG secondary Ab, and the signals were visualized using an enhanced chemiluminescence kit (Thermo Fisher Scientific Inc., Waltham, MA, USA), in accordance with the manufacturer's instructions.
We used OVA in a standard sensitization protocol in Balb/c mice to induce acute (Fig. 1A) or chronic airway inflammation (Fig. 1B). BAL was performed on the mice 24 hours after the last challenge via the inhalation of 1% OVA aerosol solution to determine the airway inflammation state. We compared total BAL cells and pathological changes after OVA inhalation because inflammatory pulmonary disease is characterized by the infiltration of inflammatory cells into the airway and tissue. BALF from OVA-challenged Balb/c mice exhibited higher total cell numbers, regardless of the duration of OVA challenge (Fig. 2A). In acute asthma inflammation, the total numbers of inflammatory cells were 0.71±0.39×106 cells/mL in the control group and 5.13±1.35×106 cells/mL in the acute asthma group. In chronic asthma inflammation, there were 1.95±0.86×106 cells/mL in the control group and 4.58±1.21×106 cells/mL in the chronic asthma group (Fig. 2A). H&E-stained lung tissue specimens showed more inflammatory cellular infiltrates in both the acute and chronic asthma groups than in the control groups (Fig. 2B).
Inflammation associated with asthma is regulated by multiple pro-inflammatory mediators. We measured the concentrations of IFN-γ, IL-4, IL-5, IL-9, IL-17, and IgE to compare the type of immune response elicited by different durations of OVA challenge. IL-4, IL-5, and IgE concentrations were significantly higher in the acute and chronic asthma groups than in either of the control groups. In the acute asthma group, IL-4 increased from 18.17±2.36 pg/mL to 112.67±21.65 pg/mL, IL-5 increased from 9.33±1.89 pg/mL to 143.57±27.67 pg/mL, and IgE increased from 0.04±0.02 ng/mL to 6.69±0.76 ng/mL. In the chronic asthma group, IL-4 increased from 19.00±1.00 pg/mL to 44.33±5.01 pg/mL, IL-5 increased from 10.20±2.80 pg/ml to 86.00±8.72 pg/mL, and IgE increased from 0.04±0.02 ng/mL to 4.17±0.51 ng/mL (Fig. 3).
IFN-γ, IL-9, and IL-17 concentrations only increased in the chronic asthma group. There were some differences in the concentration of IFN-γ (25.33±9.61 pg/mL in acute asthma vs. 41.00±13.08 pg/mL in controls), IL-9 (65.33±5.03 pg/mL in acute asthma vs. 107.33±11.02 pg/mL in controls), and IL-17 (32.43±2.10 pg/mL in acute asthma vs. 36.97±3.94 pg/mL in controls), but the differences were not statistically significant. However, in the chronic asthma group, IFN-γ increased from 21.67±2.89 pg/mL to 95.67±14.64 pg/mL, IL-9 increased from 60.67±9.24 pg/mL to 588.00±73.73 pg/mL, and IL-17 markedly increased from 35.87±4.94 pg/mL to 101.33±17.04 pg/mL (Fig. 3). These results indicate that airway inflammation induced by OVA aerosol challenge results in prominent Th2 responses in acute asthma mice, whereas chronic asthma mice display heterogeneous responses with a significant increase in IL-17 and IL-9 secretion in the lung.
During the development of allergic airway inflammation, caspase-1 activation via the inflammasome pathway leads to the production of the active form of IL-1β and contributes to the enhancement of Th17 cell differentiation.21 We induced chronic airway inflammation in caspase-1 KO mice to investigate the role of Th17 cells in asthma because caspase-1-mediated production of IL-17 is correlated with disease severity in asthmatic patients.22 We found that total BAL cells counts were increased in OVA-challenged caspase-1 KO mice (Fig. 4A), and IL-1β expression was primarily increased in wild-type OVA-challenged (WT-OVA) mice (Fig. 4B). PU.1 expression was increased in both WT-OVA and caspase-1 KO mice (Fig. 4B). Although WT-OVA mice and caspase-1 KO mice both showed increased recruitment of inflammatory cells and mucus secretion (Fig. 4C), the only difference in cell numbers between the WT and caspase-1 KO mice was observed in IL-17-producing CD4+ cells. We confirmed that a decrease in IL-1β affected Th17 subsets but not IL-9-producing CD4+ cells. Moreover, we observed that an increase in IL-9-producing CD4+ cells did not affect the induction of Th17 cells in OVA-challenged caspase-1 KO mice (Fig. 4D and E).
IL-9 expression is elevated in lungs of asthmatic patients, and IL-9 receptor (IL-9R) expression can be observed in the lungs of asthmatic individuals but not healthy controls.23-25 IL-9 has biological effects on a number of distinct cell types, and may have other effects on immune cells and resident tissue cells that contribute to the development of inflammation beyond its first description as a T-cell or mast cell growth factor. Our ELISA (Fig. 3) results suggest that IL-9 may be a crucial inflammatory mediator in chronic airway inflammation, and is not affected by IL-17 in caspase-1 KO mice (Fig. 4E). We utilized a model of chronic asthma inflammation in which OVA-sensitized Balb/c mice were subjected to a 5-week exposure to OVA to investigate the effect of anti-IL-9 Ab treatment on chronic inflammation and airway remodeling (Fig. 5A).
Mice were sacrificed after the last aerosol challenge, BALF was collected, and different cell types were counted to assess the effect of anti-IL-9 Ab on pulmonary inflammation. The total numbers of inflammatory cells in BALF were (2.54±0.73)×106 cells/mL in the untreated (control) group, (4.94±0.48)×106 cells/mL in the Iso Ab group, and (3.20±0.32)×106 cells/mL in the anti-IL-9 Ab group. The difference in cell numbers observed between the Iso Ab group and the anti-IL-9 Ab group was statistically significant (Fig. 5B). Specifically, there were significant changes in the number of eosinophils. The numbers of eosinophils were (0.25±0.07)×106 cells/mL in the control group, (3.75±0.35)×106 cells/mL in the Iso Ab group, and (1.95±0.35)×106 cells/mL in the anti-IL-9 Ab group. Monocytes and basophil cell counts decreased without statistical significance (Fig. 5C).
Histological examination of the lung tissue revealed that chronic OVA challenge induced extensive infiltration of inflammatory cells in peri-vascular and peri-bronchial tissues. This infiltrate was severe in the Iso Ab group, but markedly depressed in the anti-IL-9 Ab group. The inflammation indices, described in the Materials and Methods, were 0.25±0.5 in the control group, 2.75±0.5 in the Iso Ab group, and 1.25±0.5 in the anti-IL-9 Ab group. The mucus indices, measured by PAS staining, were 1.33%±0.15% in the control group, 13.63%±1.37% in the Iso Ab group, and 5.60%±0.82% in the anti-IL-9 Ab group (*P<0.05). The collagen indices, as calculated from the results of Sirius Red staining, were 0.46%±0.12% in the control group, 3.40%±0.82% in the Iso Ab group, and 1.77%±0.35% in the anti-IL-9 Ab group (*P<0.05; Fig. 5D and E). Together, these results suggest that neutralizing IL-9 is effective for preventing or halting tissue fibrosis during chronic asthmatic responses.
To determine the profile of airway-infiltrating cells in OVA-challenged mice with or without anti-IL-9 Ab, we analyzed changes in inflammatory cells such as eosinophils, neutrophils, mast cells, basophils, and B cells in BALF by flow cytometry (Fig. 6A). The proportion of eosinophils decreased from 34.33%±3.51% to 21.67%±3.51% in the anti-IL-9 Ab group and correlated well with the results shown in Fig. 5C. Neutrophils decreased from 37.33%±2.51% to 16.33%±1.53%, mast cells decreased from 22.67%±2.52% to 10.00%±2.00%, basophils decreased from 13.33%±3.06% to 8.33%±1.53%, and B cells decreased from 32.33%±3.21% to 22.33%±2.52%. These differences were statistically significant (Fig. 6B). Next, we examined the proportion of subsets of CD4+ helper T cells to compare the lymphocytic immune response after administration of anti-IL-9 Ab (Fig. 6C). In the anti-IL-9 Ab group, the proportion of IL-9-producing T cells significantly decreased from 23.00%±3.00% to 9.00%±2.00%, and the proportion of IL-17-producing cells significantly decreased from 12.67%±2.52% to 6.00%±1.00%. The proportions of IL-4-producing T cells and Foxp3-positive T cells did not change significantly. The proportions of IFN-γ-producing CD4+ T cells were 0.72%±0.37% in the control group, 3.33%±1.53% in the Iso Ab group, and 0.36%±0.21% in the anti-IL-9 Ab group (Fig. 6D).
Next, we evaluated the effect of anti-IL-9 Ab treatment on IgE and cytokines in the lung (Fig. 7). IL-4 and IFN-γ were markedly increased in the Iso Ab group compared to the control group, but the decrease after IL-9-neutralizing Ab injection was not statistically significant. IFN-γ levels were 29.69±7.37 pg/mL in the control, 62.5±12.02 pg/mL in the Iso Ab group, and 49.67±13.65 pg/mL in the anti-IL-4 Ab group. IL-4 levels were 20.67±2.89 pg/mL in the control group, 37.67±3.79 pg/mL in the Iso Ab group, and 31.33±3.21 pg/mL in the anti-IL-9 Ab group. In contrast, IL-5, IL-9, IL-17, and IgE levels were significantly lower in the anti-IL-9 Ab group than in the Iso Ab group. IL-5 levels were 31.00±3.61 pg/mL in the control group, 75.00±6.24 pg/mL in the Iso Ab group, and 47.67±1.53 pg/mL in the anti-IL-9 Ab group. After IL-9 Ab administration, IL-9 levels were 67.67±34.27 pg/mL in the control group, 759.33±179.05 pg/mL in the Iso Ab group, and 347.33±24.79 pg/mL in the anti-IL-9 Ab group. IL-17 levels were 13.33±1.15 pg/mL in the control group, 58.33±16.17 pg/mL in the Iso Ab group, and 28.33±9.29 pg/mL in the anti-IL-9 Ab group. IgE levels were 0.02±0.00 ng/mL in the control group, 4.20±0.28 ng/mL in the Iso Ab group, and 2.20±0.57 ng/mL in the anti-IL-9 Ab group (Fig. 7). Based on these results, IL-9 blockade significantly attenuated the levels of IL-5, IL-9, IL-17, and IgE. These data indicate that the suppression of pulmonary inflammation by anti-IL-9 Ab is mediated through a decrease in multiple inflammatory mediators, including IL-17.
We examined the relationship between IL-9 and chronic airway inflammation to determine if IL-9 blockade would affect IL-17-driven airway inflammation. After anti-IL-9 Ab administration, the number of inflammatory cells and Th17 cells in BALF, as well as the inflammation index, mucus index, and the collagen index, were significantly lower in the chronic asthma group.
IL-9 has direct and indirect effects on lymphocytes, mast cells, epithelial cells, smooth muscle cells, and hematopoietic progenitors that affect the development of immunity and inflammation. In asthma patients, IL-9R is found on mast cells and polymorphonuclear cells in the lung.26 Lung-selective expression of IL-9 is known to cause airway inflammation characterized by infiltrating eosinophils and lymphocytes and pathologic changes including epithelial cell hypertrophy, mucus production, and increased subepithelial deposition of collagen.27 Transgenic mice systemically over-expressing IL-9 display an elevated AHR to bronchoconstrictors, enhanced eosinophilic inflammation of the airways, and elevated serum IgE following sensitization and challenge with the mold Aspergillus fumigates.17 However, goblet cell hyperplasia and mastocytosis are severely impaired in IL-9-deficient mice in response to pulmonary challenge.28
IL-9 induces differentiation and proliferation of Th17 cells, enhances immunologic tolerance of Treg cells without proliferation, and increases IgE production in B cells.13 However, our results showed that even though IL-9+ CD4+ T cells were increased in OVA-challenged caspase-1 KO mice, they did not affect the number of IL-17-producing CD4+ T cells (Fig. 4E). However, after treatment with an IL-9 neutralizing Ab, the proportion of Th17 cells and secretion of IL-17 was decreased (Figs. 6D and 7). Our data suggest that IL-9 can promote the development of Th17 cells at sufficient IL-1β concentrations.
There have been several studies on changes in inflammatory reactions induced by anti-IL-9 Ab administration. Cheng et al.29 demonstrated that anti-IL-9 Ab treatment suppresses airway inflammation and hyperreactivity to methacholine challenge. They found that the Th2 cytokines IL-4, IL-5, and IL-13 were decreased in BALF after exposure to anti-IL-9 Ab, which does not agree with our results. This discrepancy may due to the fact that anti-IL-9 Ab was administered during the acute stage of inflammation in the previous study, whereas we administered the Ab during the chronic stage of inflammation. Kearley et al.30 indicated that, in a chronic asthma model, IL-9 blockade reduces the number of mast cells and production of vascular endothelial growth factor, fibroblast growth factor 2, and TGF-β, and thus suppresses AHR and remodeling. Th2 cells play a crucial role in acute allergic reactions, but not during late-phase allergic reactions and airway remodeling.31 It is interesting to note that IL-9 expression increases when Th2 cells are exposed to IL-4 and TGF-β (a representative cytokine for airway remodeling). Soroosh and Doherty32 suggested that some effector Th2 cells may switch to Th9 cells and play a role in distinct aspects of chronic lung inflammation mediated by IL-9 in the presence of IL-4 and TGF-β.
Two randomized phase-IIa clinical trials of a humanized anti-IL-9 monoclonal Ab have been performed. These studies showed that anti-IL-9 Ab has linear pharmacokinetics, induces no Ab to anti-IL-9 Ab, and has a similar frequency of adverse drug effects as the placebo.33 They also described that the maximal therapeutic effect was achieved 56 days after administration of the monoclonal Ab, which suggests that IL-9 blockade may be more effective for treating chronic allergic inflammation rather than acute allergic inflammation. Further studies with a larger sample size and a longer duration of drug administration are required before applying these results to clinical practice. In the same clinical trials, they administered the Ab twice weekly for 8 weeks, which is not feasible in a clinical setting. Safe application of anti-IL-9 Ab in patients with asthma requires longer intervals between individual drug administrations using an effective drug-delivery system.
In conclusion, anti-IL-9 Ab can reduce cellular infiltration related to chronic airway inflammation and remodeling. Treatment with an anti-IL-9 Ab can reduce the number of Th9 cells, Th17 cells, mast cells, eosinophils, and neutrophils in the airway, inhibit the synthesis and secretion of cytokines, and reduce IgE synthesis in B cells. Further studies should be performed to utilize an anti-IL-9 Ab to treat asthma patients because this Ab can improve various pathophysiological changes.
Figures and Tables
Fig. 1
Protocol for inducing experimental acute and chronic airway inflammation models. (A) In the acute asthma model, mice were sensitized by two intraperitoneal injections of OVA on days 0 and 5, and then received aerosolized OVA challenge on days 12-15 using a nebulizer. (B) Mice were sensitized via intraperitoneal injection of OVA on days 0 and 12 to induce chronic asthma. Mice were exposed to an aerosol of 1% OVA in PBS on days 18-23 using a nebulizer. After day 26, mice were exposed to aerosolized 1% OVA three times per week for 5 weeks. OVA, ovalbumin; PBS, phosphate-buffered saline.
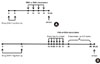
Fig. 2
OVA-induced airway inflammation in acute and chronic asthma groups. (A) BALF from acute (n=15) or chronic asthma (n=10) mice was collected 24 hours after the final OVA challenge, and the number of cells in the BALF was determined as described in the Materials and Methods. The data are expressed as the means±SEM (*P<0.05, groups were compared as indicated by Mann-Whitney U tests). (B) Mice were sacrificed 1 day after the last OVA challenge. Mice lungs were fixed with 4% paraformaldehyde, and tissue sections were stained with H&E. Images are presented at the original magnification of ×400. OVA, ovalbumin; BALF, bronchoalveolar lavage fluid; SEM, standard error of the mean; H&E, hematoxylin and eosin.
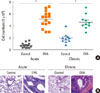
Fig. 3
Comparison of cytokine profiles after OVA challenge. Concentrations of IFN-γ, IL-4, IL-5, IL-9, IL-17, and IgE in BALF from acute asthma or chronic asthma mice were measured by ELISA. Data are expressed as the means±SEM (n=5).
*P<0.05 for control versus OVA-inhalation and acute-OVA versus chronic-OVA by Mann-Whitney U tests.
OVA, ovalbumin; IFN, interferon; IL, interleukin; BALF, bronchoalveolar lavage fluid; ELISA, enzyme-linked immunosorbent assay; SEM, standard error of the mean.
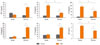
Fig. 4
Caspase-1 KO mice induced airway inflammation. (A) BALF from WT C57BL/6 (n=5) or caspase-1 KO (n=5) mice was collected 24 hours after the final OVA challenge, and the number of cells in the BALF was determined. The data are expressed as the means±SEM (*P<0.05;0.05, groups were compared as indicated by Mann-Whitney U tests). (B) BAL cells were lysed, and whole-cell extracts were resolved using 12% SDS-PAGE, transferred to polyvinylidene fluoride membranes, and probed with anti-IL-1β, anti-β-actin, and anti-PU.1 Ab. Representative blots of three independent experiments are shown. (C) Mice lungs were fixed with 4% paraformaldehyde, tissue sections were stained with H&E, and mucus was visualized by PAS staining. Images are presented at the original magnification of ×400. (D) After OVA challenge, proportions of basophils (CD49d+, FcεRI+), mast cells (c-Kit+, SSClow), eosinophils (CD49b+, SSCint), and neutrophils (Gr1+) were collected for flow cytometric analysis (n=5 for each groups). Cell numbers were calculated from the percentage of cells×BAL cell numbers. (E) The proportions of CD4+ T cells were determined according to intracellular levels of cytokines: IFN-γ+ for IFN-γ-secreting T cells, IL-4+ for IL-4-secreting T cells, IL-9+ for IL-9-secreting T cells, and IL-17+ for Th17 cells. Cell numbers were calculated from the percentage of cells subsets×BAL cell numbers. Data are expressed as the means±SEM (n=5).
*P<0.05; groups were compared as indicated by unpaired t-tests.
KO, knockout; BALF, bronchoalveolar lavage fluid; OVA, ovalbumin; SEM, standard error of the mean; SDS-PAGE, sodium dodecyl sulfate polyacrylamide gel electrophoresis; IL, interleukin; Ab, antibody; H&E, hematoxylin and eosin; PAS, periodic acid-Schiff.
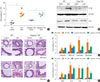
Fig. 5
Effects of IL-9 blockade on chronic pulmonary inflammation. (A) Anti-IL-9 or control Iso IgG Ab were administered (100 µg/mouse, intraperitoneally) once a week throughout the OVA induced development of chronic asthma. Five weeks of OVA challenge induced massive infiltration of eosinophils, and anti-IL-9 treatment significantly suppressed their infiltration. (B) BALF from each experimental mouse was collected 24 hours after the final OVA challenge, and the number of cells in the BALF was determined. (C) Differential cell counts were calculated as (total cell number of BAL cells)×(percentage of mononuclear cells, eosinophils, or neutrophils counted in the Giemsa-stained cytospin cell preparation). MNC=mononuclear cell. (D) IL-9 blockade attenuated tissue inflammation. The randomly selected histologic images were scored as the mean inflammatory index, which represents the severity of peri-bronchial inflammation. Mucus producing goblet cells were visualized by PAS staining. Sections were stained with Sirius Red solution to detect collagen deposition. Images are presented at the original magnification of ×400. PAS-positive goblet cells and Sirius Red-positive sub-epithelial areas for each bronchiole were calculated using image analysis software for quantitative analysis. Data are expressed as the mean±SEM (n=5).
*P<0.05; groups were compared as indicated using the Mann-Whitney U tests.
OVA, ovalbumin; BALF, bronchoalveolar lavage fluid; IL, interleukin; PAS, periodic acid-Schiff; SEM, standard error of the mean.
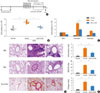
Fig. 6
IL-9 blockade altered BAL cell profiles. Flow cytometric analysis shows profiles of cells from BALF. Eosinophils (CD49b+, SSCint), neutrophils (Gr1+), mast cells (c-Kit+, SSClow), basophils (CD49d+, FcεRI+), and B cells (CD19+) were assessed. Data are presented as representative results of three independent experiments (A) and a statistical graph (B). The proportions of CD4+ T cells were determined according to intracellular levels of cytokines: IFN-γ+ for IFN-γ-secreting T cells, IL-4+ for IL-4-secreting T cells, IL-9+ for IL-9-secreting T cells, IL-17+ for Th17 cells, and Foxp3+ for Treg cells. Data are presented as representative results of three independent experiments (C) and a statistical graph (D). Data are expressed as the means±SEM (n=5) *P<0.05; groups were compared as indicated by unpaired t-tests.
BALF, bronchoalveolar lavage fluid; SEM, standard error of the mean.
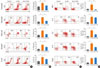
Fig. 7
IL-9 blockade alters cytokine levels in BALF. Mice were sacrificed and the levels of IgE and cytokines in BALF from OVA-challenged mice with anti-IL-9 Ab or Iso IgG Ab were measured using ELISA. Data are expressed as the means±SEM (n=5). *P<0.05; groups were compared as indicated by Mann-Whitney U tests.
IL, interleukin; BALF, bronchoalveolar lavage fluid; OVA, ovalbumin; ELISA, enzyme-linked immunosorbent assay; SEM, standard error of the mean.
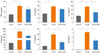
References
1. Global Initiative for Asthma (GINA). Global Strategy for Asthma Management and Prevention [Internet]. Vancouver (WA): GINA;2011. Available from: http://www.ginasthma.org/.
2. Yamauchi K, Inoue H. Airway remodeling in asthma and irreversible airflow limitation-ECM deposition in airway and possible therapy for remodeling. Allergol Int. 2007; 56:321–329.
3. Yamauchi K. Airway remodeling in asthma and its influence on clinical pathophysiology. Tohoku J Exp Med. 2006; 209:75–87.
4. Durrani SR, Viswanathan RK, Busse WW. What effect does asthma treatment have on airway remodeling? Current perspectives. J Allergy Clin Immunol. 2011; 128:439–448.
5. Payne DN, Rogers AV, Adelroth E, Bandi V, Guntupalli KK, Bush A, Jeffery PK. Early thickening of the reticular basement membrane in children with difficult asthma. Am J Respir Crit Care Med. 2003; 167:78–82.
6. Cho KA, Suh JW, Sohn JH, Park JW, Lee H, Kang JL, Woo SY, Cho YJ. IL-33 induces Th17-mediated airway inflammation via mast cells in ovalbumin-challenged mice. Am J Physiol Lung Cell Mol Physiol. 2012; 302:L429–L440.
7. Buc M, Dzurilla M, Vrlik M, Bucova M. Immunopathogenesis of bronchial asthma. Arch Immunol Ther Exp (Warsz). 2009; 57:331–344.
8. Kaiko GE, Foster PS. New insights into the generation of Th2 immunity and potential therapeutic targets for the treatment of asthma. Curr Opin Allergy Clin Immunol. 2011; 11:39–45.
9. Nair P, Pizzichini MM, Kjarsgaard M, Inman MD, Efthimiadis A, Pizzichini E, Hargreave FE, O'Byrne PM. Mepolizumab for prednisone-dependent asthma with sputum eosinophilia. N Engl J Med. 2009; 360:985–993.
10. Haldar P, Brightling CE, Hargadon B, Gupta S, Monteiro W, Sousa A, Marshall RP, Bradding P, Green RH, Wardlaw AJ, Pavord ID. Mepolizumab and exacerbations of refractory eosinophilic asthma. N Engl J Med. 2009; 360:973–984.
11. Corren J. Cytokine inhibition in severe asthma: current knowledge and future directions. Curr Opin Pulm Med. 2011; 17:29–33.
12. Berry MA, Hargadon B, Shelley M, Parker D, Shaw DE, Green RH, Bradding P, Brightling CE, Wardlaw AJ, Pavord ID. Evidence of a role of tumor necrosis factor alpha in refractory asthma. N Engl J Med. 2006; 354:697–708.
13. Goswami R, Kaplan MH. A brief history of IL-9. J Immunol. 2011; 186:3283–3288.
14. Chang HC, Sehra S, Goswami R, Yao W, Yu Q, Stritesky GL, Jabeen R, McKinley C, Ahyi AN, Han L, Nguyen ET, Robertson MJ, Perumal NB, Tepper RS, Nutt SL, Kaplan MH. The transcription factor PU.1 is required for the development of IL-9-producing T cells and allergic inflammation. Nat Immunol. 2010; 11:527–534.
15. Putheti P, Awasthi A, Popoola J, Gao W, Strom TB. Human CD4 memory T cells can become CD4+IL-9+ T cells. PLoS One. 2010; 5:e8706.
16. Staudt V, Bothur E, Klein M, Lingnau K, Reuter S, Grebe N, Gerlitzki B, Hoffmann M, Ulges A, Taube C, Dehzad N, Becker M, Stassen M, Steinborn A, Lohoff M, Schild H, Schmitt E, Bopp T. Interferon-regulatory factor 4 is essential for the developmental program of T helper 9 cells. Immunity. 2010; 33:192–202.
17. McLane MP, Haczku A, van de Rijn M, Weiss C, Ferrante V, MacDonald D, Renauld JC, Nicolaides NC, Holroyd KJ, Levitt RC. Interleukin-9 promotes allergen-induced eosinophilic inflammation and airway hyperresponsiveness in transgenic mice. Am J Respir Cell Mol Biol. 1998; 19:713–720.
18. Postma DS, Bleecker ER, Amelung PJ, Holroyd KJ, Xu J, Panhuysen CI, Meyers DA, Levitt RC. Genetic susceptibility to asthma--bronchial hyperresponsiveness coinherited with a major gene for atopy. N Engl J Med. 1995; 333:894–900.
19. Nicolaides NC, Holroyd KJ, Ewart SL, Eleff SM, Kiser MB, Dragwa CR, Sullivan CD, Grasso L, Zhang LY, Messler CJ, Zhou T, Kleeberger SR, Buetow KH, Levitt RC. Interleukin 9: a candidate gene for asthma. Proc Natl Acad Sci U S A. 1997; 94:13175–13180.
20. Choi JM, Ahn MH, Chae WJ, Jung YG, Park JC, Song HM, Kim YE, Shin JA, Park CS, Park JW, Park TK, Lee JH, Seo BF, Kim KD, Kim ES, Lee DH, Lee SK, Lee SK. Intranasal delivery of the cytoplasmic domain of CTLA-4 using a novel protein transduction domain prevents allergic inflammation. Nat Med. 2006; 12:574–579.
21. Sutton C, Brereton C, Keogh B, Mills KH, Lavelle EC. A crucial role for interleukin (IL)-1 in the induction of IL-17-producing T cells that mediate autoimmune encephalomyelitis. J Exp Med. 2006; 203:1685–1691.
22. Bullens DM, Truyen E, Coteur L, Dilissen E, Hellings PW, Dupont LJ, Ceuppens JL. IL-17 mRNA in sputum of asthmatic patients: linking T cell driven inflammation and granulocytic influx? Respir Res. 2006; 7:135.
23. Erpenbeck VJ, Hohlfeld JM, Discher M, Krentel H, Hagenberg A, Braun A, Krug N. Increased expression of interleukin-9 messenger RNA after segmental allergen challenge in allergic asthmatics. Chest. 2003; 123:370S.
24. Shimbara A, Christodoulopoulos P, Soussi-Gounni A, Olivenstein R, Nakamura Y, Levitt RC, Nicolaides NC, Holroyd KJ, Tsicopoulos A, Lafitte JJ, Wallaert B, Hamid QA. IL-9 and its receptor in allergic and nonallergic lung disease: increased expression in asthma. J Allergy Clin Immunol. 2000; 105:108–115.
25. Bhathena PR, Comhair SA, Holroyd KJ, Erzurum SC. Interleukin-9 receptor expression in asthmatic airways In vivo. Lung. 2000; 178:149–160.
26. Abdelilah S, Latifa K, Esra N, Cameron L, Bouchaib L, Nicolaides N, Levitt R, Hamid Q. Functional expression of IL-9 receptor by human neutrophils from asthmatic donors: role in IL-8 release. J Immunol. 2001; 166:2768–2774.
27. Temann UA, Ray P, Flavell RA. Pulmonary overexpression of IL-9 induces Th2 cytokine expression, leading to immune pathology. J Clin Invest. 2002; 109:29–39.
28. Townsend JM, Fallon GP, Matthews JD, Smith P, Jolin EH, McKenzie NA. IL-9-deficient mice establish fundamental roles for IL-9 in pulmonary mastocytosis and goblet cell hyperplasia but not T cell development. Immunity. 2000; 13:573–583.
29. Cheng G, Arima M, Honda K, Hirata H, Eda F, Yoshida N, Fukushima F, Ishii Y, Fukuda T. Anti-interleukin-9 antibody treatment inhibits airway inflammation and hyperreactivity in mouse asthma model. Am J Respir Crit Care Med. 2002; 166:409–416.
30. Kearley J, Erjefalt JS, Andersson C, Benjamin E, Jones CP, Robichaud A, Pegorier S, Brewah Y, Burwell TJ, Bjermer L, Kiener PA, Kolbeck R, Lloyd CM, Coyle AJ, Humbles AA. IL-9 governs allergen-induced mast cell numbers in the lung and chronic remodeling of the airways. Am J Respir Crit Care Med. 2011; 183:865–875.
31. Doherty TA, Soroosh P, Broide DH, Croft M. CD4+ cells are required for chronic eosinophilic lung inflammation but not airway remodeling. Am J Physiol Lung Cell Mol Physiol. 2009; 296:L229–L235.
32. Soroosh P, Doherty TA. Th9 and allergic disease. Immunology. 2009; 127:450–458.
33. Parker JM, Oh CK, La Force C, Miller SD, Pearlman DS, Le C, Robbie GJ, White WI, White B, Molfino NA. MEDI-528 Clinical Trials Group. Safety profile and clinical activity of multiple subcutaneous doses of MEDI-528, a humanized anti-interleukin-9 monoclonal antibody, in two randomized phase 2a studies in subjects with asthma. BMC Pulm Med. 2011; 11:14.