Abstract
BACKGROUND/OBJECTIVES
Several medicinal properties of Smilax china L. have been studied including antioxidant, anti-inflammatory, and anti-cancer effects. However, the antiobesity activity and mechanism by which the water-soluble fraction of this plant mediates its effects are not clear. In the present study, we investigated the lipolytic actions of the water-soluble fraction of Smilax china L. leaf ethanol extract (wsSCLE) in 3T3-L1 adipocytes.
MATERIALS/METHODS
The wsSCLE was identified by measuring the total polyphenol and flavonoid content. The wsSCLE was evaluated for its effects on cell viability, lipid accumulation, glycerol, and cyclic adenosine monophosphate (cAMP) contents. In addition, western blot analysis was used to evaluate the effects on protein kinase A (PKA), PKA substrates (PKAs), and hormone-sensitive lipase (HSL). For the lipid accumulation assay, 3T3-L1 adipocytes were treated with different doses of wsSCLE for 9 days starting 2 days post-confluence. In other cell experiments, mature 3T3-L1 adipocytes were treated for 24 h with wsSCLE.
RESULTS
Results showed that treatment with wsSCLE at 0.05, 0.1, and 0.25 mg/mL had no effect on cell morphology and viability. Without evidence of toxicity, wsSCLE treatment decreased lipid accumulation compared with the untreated adipocyte controls as shown by the lower absorbance of Oil Red O stain. The wsSCLE significantly induced glycerol release and cAMP production in mature 3T3-L1 cells. Furthermore, protein levels of phosphorylated PKA, PKAs, and HSL significantly increased following wsSCLE treatment.
Obesity is a metabolic disease caused by the accumulation of surplus energy source in adipocytes as a result of imbalanced nutrient absorption and energy consumption [1]. Excessive fat accumulation in adipocytes is associated with the development of chronic conditions such as diabetes, hypertension, and cardiovascular disease [2]. Therefore, many studies have been performed to investigate how to prevent fat accumulation in cells and how to regulate the breakdown of accumulated fat. Lipid droplets in adipocytes are known to play an important role in the regulation of lipid metabolism. Furthermore, the breakdown of triglycerides, which are the main components of lipid droplets, and the resultant secretion of glycerol, is thought to be an important mechanism and biomarker of the inhibition of fat accumulation within cells [34]. Lipase, in particular, plays an important role in the breakdown of triglycerides [4].
Hormone-sensitive lipase (HSL) plays a major role in the lipolytic process in lipid droplets and its activity is regulated by hormones such as insulin and epinephrine [5]. Phosphorylation by protein kinase A (PKA) or by extracellular signal-regulated kinase (ERK) plays an important role in HSL regulation. In other words, PKA phosphorylates HSL at residues serine 563 (Ser563), 659 (Ser659), and 660 (Ser660) and ERK phosphorylates HSL at serine 600 (Ser600) (numbering for rat HSL) [56]. Phosphorylation of Ser660 on HSL induces its translocation to lipid droplets, and phosphorylation of Ser563 and Ser600 has an effect on lipase activation [57]. Unlike insulin, which is the most potent antilipolytic hormone, catecholamines such as epinephrine mediate lipolytic action by associating with β-adrenergic receptors (β-AR) on adipocytes [8]. Stimulation of catecholamine-induced β-AR signaling increases cyclic AMP (cAMP) levels within cells, which in turn induces PKA activity. Furthermore, as noted, HSL activated by PKA-mediated phosphorylation breaks down triglyceride into glycerol and fatty acids [58].
The U.S. Food and Drug Administration (FDA) have approved the antiobesity drugs sibutramine and orlistat for clinical use. Sibutramine induces weight loss by suppressing appetite and increasing satiety, whereas orlistat affects weight loss by controlling fat absorption due to the inhibition of lipase activation in the pancreas and gastrointestinal tract [9]. However, sibutramine has side effects that include a dry mouth, insomnia, and constipation, whereas orlistat has side effects that include gastroenteric trouble, hypersensitivity, dyscholia, and the suppression of fat-soluble vitamin uptake. Furthermore, the efficacy of orlistat drugs has been reported to decrease with long-term use [1011]. Therefore, research has focused on materials and food that can help reduce the side effects when used in combination with sibutramine or orlistat or have antiobesity effects when used on their own [1213].
Smilax china L. leaves are known to have antioxidant effects and flavonoids such as kaempferol-7-O-α-l-rhamnopyranoside and kaempferol-3,7-O-α-l-dirhamnopyranoside have been reported as the active components of these leaves [14]. In addition, phenol compounds such as resveratrol and kaempferol, which are found in Smilax china L. leaves, have been reported to have antiobesity effects that are mediated by the inhibition of adipocyte differentiation and fat accumulation [151617]. Furthermore, Wang et al. [18] reported that polyphenol intake could be beneficial against obesity. Accordingly, this study aimed to investigate the antiobesity effects and the mechanisms involved in extracts of Smilax china L. leaves.
The Smilax china L. leaves that were used in this study were collected in Uiryeong in Kyeongsangnamdo, Korea and examined by Professor Heung-Mook Shin (College of Oriental Medicine, Dongguk University, Gyeongju, Korea). Smilax china L. leaves were dried in the shade and pulverized into the fine powder. The extract of the dried leaves was extracted with the addition of 80% ethanol (10-fold of their weight) and by heating at 80℃ for 5 h in a reflux extractor. The mixture was cooled to room temperature and centrifuged at 3,000×g for 30 min. The supernatant was collected and filtered through Whatman filter paper (Whatman International, Maidstone, UK) and evaporated in a rotary evaporator (Eyela SB-1000, Tokyo, Japan) followed by lyophilizing in a lyophilizer (FD 8508, Ilshin, Korea). The yield was 8.8% of the dry weight of Smilax china L. leaves. According to the method described by Sakagami Y [19], 3 g of dried extracts were dissolved in 10 mL of sterile water and the supernatant was moved to fresh tubes and lyophilized. The water-soluble fraction (wsSCLE, 1.71 g) was obtained and the remainder of the sample was dissolved in dimethylsulfoxide (DMSO) to obtain the fat-soluble fraction. In present study, the wsSCLE was used.
The total phenolic content was measured by a colorimetric assay using Folin-Ciocalteu's phenol reagent [20]. A total of 0.96 mL of distilled water and 0.1 mL of 50% Folin-Ciocalteu's phenol were added to 0.04 mL of the sample, which was diluted with distilled water and incubated for 3 min. Then, 0.2 mL of 10% Na2CO3 was added, the mixture was incubated for 1 h, and the absorbance was subsequently measured at 700 nm. The total polyphenol content was calculated from a standard curve generated using gallic acid (Sigma, St. Louis, MO, USA).
The total flavonoid contents were determined according to the method described by Moreno et al. [21]. A total of 10% aluminum nitrate 0.02 mL, 1 M potassium acetate 0.02 mL, and 80% ethanol 0.86 mL were mixed with 0.4 mL of each sample, and each mixture was then incubated for 40 min at room temperature. Absorbance was measured at 415 nm using a spectrophotometer (Optizen 2120 UV, Mecasys, Korea). The total flavonoid content was determined from a calibration curve using quercetin (Wako, Osaka, Japan) as a standard.
3T3-L1 preadipocytes were purchased from the American Type Culture Collection (ATCC, Manassas, VA, USA) and cultured in a 5% CO2 incubator at 37℃ in Dulbecco's modified Eagle's medium (DMEM) supplemented with 10% bovine calf serum (Welgene, Daegu, Korea) and 1% penicillin-streptomycin (Welgene, Daegu, Korea). Cytotoxicity was determined using the 3-[4,5-dimethylthiazole-2-yl]-2,5-di-phenyl-tetrazolium bromide (MTT) reduction method [22]. 100 µL 3T3-L1 preadipocytes was aliquoted and placed into each well of a 96-well plate at a density of 1 × 104 cells/well and incubated for 24 h. Samples were then treated at each concentration in the medium without FBS and antibiotics. After incubation for 24 h, the MTT solution (5 mg/mL) was added and samples were incubated for 4 h, following which the supernatant was removed. DMSO (100 µL) was added into each well to dissolve the formazan, and the plates were incubated on a shaker. After 30 min, absorbance was measured at 540 nm using a microplate reader (EL808, BioTek, Winooski, USA). The cytotoxicity was compared using the absorbance value of untreated control cells as the baseline.
3T3-L1 cell differentiation and lipid accumulation were determined according to the method described by Oh et al. [23]. To study adipocyte differentiation, 3T3-L1 preadipocytes were aliquoted into 6-well plates at a density of 5 × 105 cells/well to ensure confluency, and the cells were further incubated for 2 days. Differentiation was subsequently induced by incubating the cells with DMEM supplemented with MDI [0.5 mM 3-isobutyl-1-methylxanthine (IBMX), 1 µM dexamethasone, 10 µg/mL insulin] solution and 10% FBS for 3 days. The media was subsequently replaced with DMEM supplemented with 10 µg/mL insulin and 10% FBS, and cells were incubated for a further 3 days. The media was subsequently replaced with DMEM supplemented with 10% FBS only and cells were incubated again for a further 3 days, which allowed for complete adipocyte differentiation and for the formation of lipid droplets as a result of lipid accumulation within cells. The accumulated lipid content was determined by fixing differentiated cells with 10% formalin and staining them for 1 h with Oil Red O staining solution (Sigma-Aldrich, St. Louis, USA). The size and quantity of the stained lipid droplets were observed under a microscope. The content of lipid droplets can be quantified and compared by extracting Oil Red O stain from lipid droplets using 100% isopropanol and measuring the absorbance using a microplate reader at 520 nm.
This study does not target the human subject, and does not use the tissues or cells of human origin. Therefore, this research plan does not need the approval of Kangwon national University Institutional Review Board.
Differentiated 3T3-L1 adipocytes were cultured with 0.1 or 0.25 mg/mL of wsSCLE and 10 µM of the PKA inhibitor H89 (Sigma-Aldrich, St. Louis, USA) for 24 h. This medium was used in measurement of glycerol release level. The medium and glycerol reference material were placed into 96-well plates and mixed with a free glycerol detection reagent (Abcam, Cambridge, UK). Cells were incubated at 37℃ for 30 min and absorbance was measured at 570 nm using a microplate reader.
Differentiated 3T3-L1 adipocytes were treated with 0.1 or 0.25 mg/mL of wsSCLE and a β-adrenergic receptor (β-AR) agonist and antagonist, 10 µM isoproterenol (Sigma-Aldrich, St. Louis, USA) and 100 µM propranolol (Sigma-Aldrich, St. Louis, USA), respectively, for 24 h. The media was removed and then cells were washed with phosphate buffer saline (PBS). The cells were lysed via a freeze-thaw method using cell lysis buffer. After cells were completely lysed, cellular debris was removed by centrifugation at 3,000 × g for 10 min, and the protein concentration was quantified using the Bradford assay (Bio-Rad Laboratories, Hercules, USA) to correct for cell count. The cAMP level was measured using a cAMP ELISA kit (Abcam, Cambridge, UK) and was quantified based on the manufacturer's instructions.
Equal concentrations of protein were mixed with the sample buffer, which was supplemented with sodium dodecyl sulfate (SDS) and β-mercapto-ethanol, at a ratio of 3:1 and were heated at 100℃ for 3 min. Each protein sample was subjected to SDS gel electrophoresis and was then transferred to a polyvinylidene fluoride membrane (0.45 µm, PVDF transfer membrane, Thermo, Rockford, IL, USA). The membrane was placed in blocking buffer consisting of tris-buffered saline (TBS) supplemented with 0.1% tween 20 and 5% skim milk and was incubated for 2 h. The membrane was subsequently incubated in buffer containing primary antibody for 2 h. The antibodies, and dilutions used, were as follows: adenylate cyclase (1000:1) (Abcam, Cambridge, UK); PKA (2500:1); p-PKA (2000:1); p-PKA substrate (2000:1); hormone-sensitive lipase (HSL; 3000:1); GAPDH (2000:1; Santa Cruz Biotechnology, Santa Cruz, CA, USA); p-HSL (Ser563; 1000:1);and p-HSL (Ser660; 1000:1; Cell Signaling Technology, Danvers, MA, USA). Membranes were then washed with TBS-T (TBS containing 0.1% tween 20) three times for 5 min each. The membrane was then incubated for 1 h in buffer including horseradish peroxidase-conjugated secondary antibody (2000:1) (Santa Cruz Biotechnology, Santa Cruz, CA, USA) and was then exposed to x-ray film using an enhanced chemiluminescence method. The intensity of detected bands was analyzed using ImageJ software (National institutes of health, Bethesda, MD, USA).
After separating the water soluble fraction (wsSCLE) from the Smilax china L. leaf ethanol extract, total phenolic and total flavonoid contents measured using gallic acid and quercetin as standards were found to be 275.12 ± 13.67 mg/g and 137.61 ± 11.82 mg/g, respectively (Fig. 1A). The cytotoxicity to 3T3-L1 preadipocytes was examined using 0.05, 0.1, 0.25, 0.5, and 1 mg/mL of wsSCLE, where the polyphenol and flavonoid contents were verified. The results show that no cytotoxicity was observed up to 0.25 mg/mL, but the percent cell viability was 88.01 ± 4.61% and 73.23 ± 4.33%, at 0.5 mg/mL and 1 mg/mL, respectively, confirming significant cytotoxicity at those concentrations (Fig. 1B). Based upon the results of the cytotoxicity assay, wsSCLE concentrations below 0.25 mg/mL were used in all subsequent studies.
Oil Red O staining was performed to examine the effects of wsSCLE on lipid accumulation in 3T3-L1 adipocytes. The results verified that the quantity of lipid droplets in adipocytes treated with 0.1 and 0.25 mg/mL of wsSCLE decreased more than the quantity of lipid droplets in untreated differentiated adipocytes (Fig. 2A). In addition, the absorbance was measured by extracting Oil Red O stain from lipid droplets with 100% isopropanol. The results show that the absorbance in adipocytes (0.89 ± 0.08) increased significantly more than that of preadipocytes (0.65 ± 0.11), and that the absorbance of adipocytes was decreased following treatment with 0.1 and 0.25 mg/mL wsSCLE (0.66 ± 0.10 and 0.64 ± 0.07, respectively). The decreased absorbance observed following wsSCLE treatment is similar to that observed in preadipocytes.
To examine the effects of wsSCLE on lipolysis in 3T3-L1 adipocytes, glycerol release into the incubating medium was measured. The results revealed that 6.60 ± 0.69 and 10.46 ± 0.66 nM of glycerol were detected in cell culture medium treated with 0.1 and 0.25 mg/mL of wsSCLE, respectively. When compared with the control (3.98 ± 0.21 nM), the release of glycerol was shown to increase in a concentration-dependent manner with increasing wsSCLE (Fig. 3A).In addition, increased glycerol release (10.22 ± 0.16 nM) following treatment with 0.25 mg/mL of wsSCLE decreased (5.18 ± 0.33 nM) with treatment with the PKA inhibitor, H89 (10 µM).This was not statistically different from the glycerol content (3.95 ± 0.36 nM) observed in adipocytes that were treated only with 10 µM of H89.
Based upon the results showing that wsSCLE-induced glycerol release decreased following treatment with the PKA inhibitor, H89 (10 µM), the effect of wsSCLE on AC and PKA was examined. As shown in Fig. 4A, the expression of AC was affected following treatment with wsSCLE, but this was not statistically significant. The expression of total PKA was not found to be affected following treatment with wsSCLE; however, there was a significant change in the expression of phosphorylated PKA. In the same way that the PKA phosphorylation that was induced by the β-AR agonist (Isoproterenol, 10 µM) was subsequently decreased by the β-AR antagonist (propranolol, 100 µM), the phosphorylation of PKA that was induced by wsSCLE (0.25 mg/mL) was subsequently decreased following treatment with propranolol. In addition, the phosphorylation of PKA substrate was shown to increase following treatment with wsSCLE, which was shown to be inhibited by treatment of H89 (Fig. 4B).
Based on the finding that wsSCLE induced the phosphorylation of PKA, the effect of wsSCLE on cAMP synthesis was examined. As shown in Fig. 5, the results showed that wsSCLE induced cAMP synthesis in a concentration-dependent manner. As the cAMP level (1.88 ± 0.23) that was increased by β-AR agonist (isoproterenol, 10 µM) was decreased (0.79 ± 0.11) in response to the β-AR antagonist (propranolol, 100 µM), cAMP levels (3.21 ± 0.55) that were increased by wsSCLE (0.25 mg/mL) were decreased (0.37 ± 0.47) following treatment with propranolol (100 µM).
The above test results indicate that wsSCLE activated the β-AR-cAMP-PKA signaling pathway. Based on these findings, the effect of PKA on the phosphorylation of HSL was examined. As shown in Fig. 6, the result showed that HSL was phosphorylated on Ser563 when treated with wsSCLE (0.25 mg/mL). Following the same pattern as described for the cAMP level and the p-PKA expression above, the level of p-HSL (Ser563) that increased in response to the β-AR agonist (isoproterenol, 10 µM) was decreased in response to treatment with the PKA inhibitor, H89. Similarly, the level of p-HSL (Ser563) that was increased following treatment with wsSCLE (0.25 mg/mL) decreased in response to treatment with H89. However, the level of Ser660, which affected the localization of HSL to the lipid droplets, did not increase significantly following treatment with wsSCLE.
Previous studies have investigated the antioxidant activity of Smilax china L. leaf extracts, including their electron donating ability, nitrite radical scavenging activity, antibacterial activity [1424], and antidiabetic activity, including the inhibition of α-amylase and α-glucosidase [25]. However, there have been few studies on the antiobesity activity of the extracts. Recently, Park et al. [26] reported that an ethanol extract of Smilax china L. leafs inhibited the lipid accumulation in 3T3-L1 adipocytes, but its mechanism has not been studied. Thus, in the present study, an ethanol extract of Smilax china L. leaf was divided into water-soluble and fat-soluble fractions, and the water-soluble fraction was used to investigate its antiobesity effects and identify the mechanism(s) involved.
As previously stated, Wang et al. [18] reported that polyphenol intake has beneficial effects on antiobesity. Thus, the polyphenol and flavonoid contents of wsSCLE, the test material of the present study, were measured using standard curves generated using gallic acid and quercetin. As a result, total phenolic and total flavonoid contents were measured as 275 mg/g and 134 mg/g, respectively, in this study, which were over 2-fold higher than those measured at 128 mg/g and 42 mg/gin a previous study using a water extract of Smilax china L. leaf by Kim et al. [20]. This suggests that the extraction method used in the present study may be used to increase the content of phenolic compounds in Smilax china L. leaf extracts in future studies. Cytotoxicity of 3T3-L1 preadipocytes was examined using wsSCLE with confirmed polyphenol and flavonoid contents, and cytotoxicity was not observed at concentrations of 0.25 mg/mL or below. This level of toxicity is higher than that found in a study using raw 264.7 cells by Kim et al. [20], and might be attributed to the difference in cell type or the higher polyphenol and flavonoid contents in the extracts. However, further study is required for a more clear explanation.
Based upon the results reported by Park et al. [26], accumulation of lipid droplets in 3T3-L1 adipocytes was examined to confirm the antiobesity effects of wsSCLE. The result showed that the accumulation of lipid droplets decreased with treatment of wsSCLE. In addition, the glycerol content in cell culture medium increased in a concentration-dependent manner along with wsSCLE treatment. The increased glycerol release decreased following treatment with the PKA inhibitor, H89. This suggests that wsSCLE was able to break down the triglycerides that had accumulated in 3T3-L1 adipocytes, and its strong correlation with PKA activity could be predicted. Thus, the expression of adenylate cyclase (AC), which is capable of synthesizing the cAMP that is necessary for PKA activation, was examined in order to identify the mechanism of PKA activation. The expression and phosphorylation of PKA were also observed. Brasaemle et al. [27] showed that lipolytic activity stimulated by the β-AR agonist isoproterenol was inhibited by the β-AR antagonist, propranolol. An application of the test was carried out in this study. The result showed that treatment with isoproterenol and wsSCLE tended to increase the expression of AC, although this was not statistically significant. However, the expression of phosphorylated PKA increased significantly even though the expression of total PKA was not affected with the treatment of isoproterenol and wsSCLE. A previous study reported that β-adrenergic stimulation activated PKA, which in turn, induced phosphorylation of PKA substrate [28]. Based upon the results that phosphorylation of PKA increased with the treatment of wsSCLE, changes in the expression of phosphorylated PKA substrate were examined to determine whether the β-AR-PKA pathway was activated. The results showed that phosphorylation of PKA substrate increased with the treatment of wsSCLE, confirming that the β-AR-PKA pathway was activated.
Previous studies showed that PKA activation resulted from the activation of AC and the subsequent increase in cAMP levels [29]. Therefore, cAMP levels in 3T3-L1 adipocytes were measured in the present study to investigate this further. The result showed that the level of cAMP increased in a concentration-dependent manner when treated with wsSCLE, and that this increase was inhibited following treatment with propranolol, consistent with the findings of previous studies. This indicates that β-AR was stimulated following treatment with wsSCLE, which led to an increase in cAMP and in turn, the activation of PKA. In addition, this cAMP-increase was reduced when β-adrenergic stimulation was inhibited. Although wsSCLE was not found to have a significant effect on the protein expression of AC, the results of the present study suggest that wsSCLE can activate AC by the stimulation of β-AR.
Lastly, based upon the previous results showing activation of the β-AR-cAMP-PKA pathway, we examined the phosphorylation of HSL, which is the most important enzyme involved in the breakdown of triglycerides into fatty acid and glycerol. As previously stated, HSL can be regulated by various mechanisms depending on the site of phosphorylation. In this study, phosphorylation of Ser563 and Ser660 were examined [567]. The results showed that wsSCLE did not have an effect on the phosphorylation of Ser660 but it had a significant effect on the phosphorylation of Ser563. In addition, phosphorylation of Ser563, which increased with the treatment of wsSCLE as isoproterenol, decreased with the treatment of H89. Ser660 phosphorylation was increased by isoproterenol in the same was as Ser563 phosphorylation, but the former did not increase with the treatment of wsSCLE. This verified that wsSCLE activated HSL by phosphorylation of HSL Ser563 via the β-AR-cAMP-PKA pathway and the subsequent break down of triglycerides in 3T3-L1 adipocyte into fatty acid and glycerol.
In conclusion, wsSCLE contains polyphenol and flavonoid in abundance compared to water extracts and can phosphorylate HSL Ser563 by activating the β-AR-cAMP-PKA pathway, which increases HSL activation, leading to the breakdown of triglycerides in 3T3-L1 adipocyte into fatty acid and glycerol. In a future study, the in vivo effects of wsSCLE may be examined to provide a basic data for its application as a functional material for antiobesity.
References
1. McMorrow AM, Connaughton RM, Lithander FE, Roche HM. Adipose tissue dysregulation and metabolic consequences in childhood and adolescent obesity: potential impact of dietary fat quality. Proc Nutr Soc. 2015; 74:67–82. PMID: 25497038.


3. Okabe Y, Shimada T, Horikawa T, Kinoshita K, Koyama K, Ichinose K, Aburada M, Takahashi K. Suppression of adipocyte hypertrophy by polymethoxyflavonoids isolated from Kaempferia parviflora. Phytomedicine. 2014; 21:800–806. PMID: 24629599.


4. Chaves VE, Frasson D, Kawashita NH. Several agents and pathways regulate lipolysis in adipocytes. Biochimie. 2011; 93:1631–1640. PMID: 21658426.


5. Holm C. Molecular mechanisms regulating hormone-sensitive lipase and lipolysis. Biochem Soc Trans. 2003; 31:1120–1124. PMID: 14641008.


6. Okumura T, Harada K, Oue K, Zhang J, Asano S, Hayashiuchi M, Mizokami A, Tanaka H, Irifune M, Kamata N, Hirata M, Kanematsu T. Phospholipase C-related catalytically inactive protein (PRIP) regulates lipolysis in adipose tissue by modulating the phosphorylation of hormone-sensitive lipase. PLoS One. 2014; 9:e100559. PMID: 24945349.


7. Kim SO, Sakchaisri K, Asami Y, Ryoo IJ, Choo SJ, Yoo ID, Soung NK, Kim YS, Jang JH, Kim BY, Ahn JS. Illudins C2 and C3 stimulate lipolysis in 3T3-L1 adipocytes and suppress adipogenesis in 3T3-L1 preadipocytes. J Nat Prod. 2014; 77:744–750. PMID: 24597820.


8. Jaworski K, Sarkadi-Nagy E, Duncan RE, Ahmadian M, Sul HS. Regulation of triglyceride metabolism. IV. Hormonal regulation of lipolysis in adipose tissue. Am J Physiol Gastrointest Liver Physiol. 2007; 293:G1–G4. PMID: 17218471.
9. Chaput JP, St-Pierre S, Tremblay A. Currently available drugs for the treatment of obesity: Sibutramine and orlistat. Mini Rev Med Chem. 2007; 7:3–10. PMID: 17266632.


10. Collins P, Williams G. Drug treatment of obesity: from past failures to future successes? Br J Clin Pharmacol. 2001; 51:13–25. PMID: 11167661.


11. Li M, Cheung BM. Pharmacotherapy for obesity. Br J Clin Pharmacol. 2009; 68:804–810. PMID: 20002075.


12. Vickers SP, Cheetham SC, Headland KR, Dickinson K, Grempler R, Mayoux E, Mark M, Klein T. Combination of the sodium-glucose cotransporter-2 inhibitor empagliflozin with orlistat or sibutramine further improves the body-weight reduction and glucose homeostasis of obese rats fed a cafeteria diet. Diabetes Metab Syndr Obes. 2014; 7:265–275. PMID: 25061325.


13. Kumar P, Bhandari U, Jamadagni S. Fenugreek seed extract inhibit fat accumulation and ameliorates dyslipidemia in high fat diet-induced obese rats. Biomed Res Int. 2014; 2014:606021. PMID: 24868532.


14. Cha BC, Lee EH. Antioxidant activities of flavonoids from the leaves of Smilax china linne. Korean J Pharmacogn. 2007; 38:31–36.
15. Kim SH, Ahn JH, Jeong JY, Kim SB, Jo YH, Hwang BY, Lee MK. Tyrosinase inhibitory phenolic constituents of Smilax china leaves. Korean J Pharmacogn. 2013; 44:220–223.
16. Aguirre L, Fernández-Quintela A, Arias N, Portillo MP. Resveratrol: anti-obesity mechanisms of action. Molecules. 2014; 19:18632–18655. PMID: 25405284.


17. Park UH, Jeong JC, Jang JS, Sung MR, Youn H, Lee SJ, Kim EJ, Um SJ. Negative regulation of adipogenesis by kaempferol, a component of Rhizoma Polygonati falcatum in 3T3-L1 cells. Biol Pharm Bull. 2012; 35:1525–1533. PMID: 22975504.
18. Wang S, Moustaid-Moussa N, Chen L, Mo H, Shastri A, Su R, Bapat P, Kwun I, Shen CL. Novel insights of dietary polyphenols and obesity. J Nutr Biochem. 2014; 25:1–18. PMID: 24314860.


19. Sakagami Y. Inhibitory effect of the extract of Prunus mume Sieb. et Zucc. on vero-toxin production by enterohemorrhagic Escherichia coli O157 : H7. Biocontrol Sci. 2001; 6:53–56.
20. Kim KK, Kang YH, Kim DJ, Kim TW, Choe M. Comparison of antioxidant, α-glucosidase inhibition and anti-inflammatory activities of the leaf and root extracts of Smilax china L. J Nutr Health. 2013; 46:315–323.
21. Moreno MI, Isla MI, Sampietro AR, Vattuone MA. Comparison of the free radical-scavenging activity of propolis from several regions of Argentina. J Ethnopharmacol. 2000; 71:109–114. PMID: 10904153.


22. Yadav D, Chaudhary AA, Garg V, Anwar MF, Rahman MM, Jamil SS, Khan HA, Asif M. In vitro toxicity and antidiabetic activity of a newly developed polyherbal formulation (MAC-ST/001) in streptozotocin-induced diabetic Wistar rats. Protoplasma. 2013; 250:741–749. PMID: 23053765.


23. Oh SD, Kim M, Min BI, Choi GS, Kim SK, Bae H, Kang C, Kim DG, Park BJ, Kim CK. Effect of Achyranthes bidentata Blume on 3T3-L1 adipogenesis and rats fed with a high-fat diet. Evid Based Complement Alternat Med. 2014; 2014:158018. PMID: 24963319.
24. Jin TY, Park JR, Kim JH. Electron donating abilities, nitrite scavenging effects and antimicrobial activities of Smilax china leaf. J Korean Soc Food Sci Nutr. 2004; 33:621–625.
25. Lee SY, Kim JH, Park JM, Lee IC, Lee JY. Antioxidant activity and inhibition activity against α-amylase and α-glucosidase of Smilax china L. Korean J Food Preserv. 2014; 21:254–263.


26. Park HS, Kim GH, Shim SM. Different effect of methanol extracts and bioaccessible fraction of Smilax china on triglyceride accumulation in adipocytes. J Food Biochem. 2014; 38:1–5.


27. Brasaemle DL, Levin DM, Adler-Wailes DC, Londos C. The lipolytic stimulation of 3T3-L1 adipocytes promotes the translocation of hormone-sensitive lipase to the surfaces of lipid storage droplets. Biochim Biophys Acta. 2000; 1483:251–262. PMID: 10634941.


28. Egan JJ, Greenberg AS, Chang MK, Londos C. Control of endogenous phosphorylation of the major cAMP-dependent protein kinase substrate in adipocytes by insulin and beta-adrenergic stimulation. J Biol Chem. 1990; 265:18769–18775. PMID: 2172232.


29. Guellich A, Mehel H, Fischmeister R. Cyclic AMP synthesis and hydrolysis in the normal and failing heart. Pflugers Arch. 2014; 466:1163–1175. PMID: 24756197.


Fig. 1
Total phenolic content of a water-soluble fraction of Smilax china L. leaf ethanol extract (wsSCLE) and its effects on cell viability.
(A) The total phenolic and total flavonoid contents were expressed as mg of gallic acid and quercetin equivalents per g of dry extract. (B) Effect of wsSCLE on the viability of 3T3-L1 cells. Cells were treated with wsSCLE at 0.05, 0.1, 0.25, 0.5, or 1 mg/mL for 24 h. Cell viability was determined using a MTT assay kit. Data are expressed as mean ± SD of triplicate experiments, *
P < 0.05 compared to control cells (white bar).
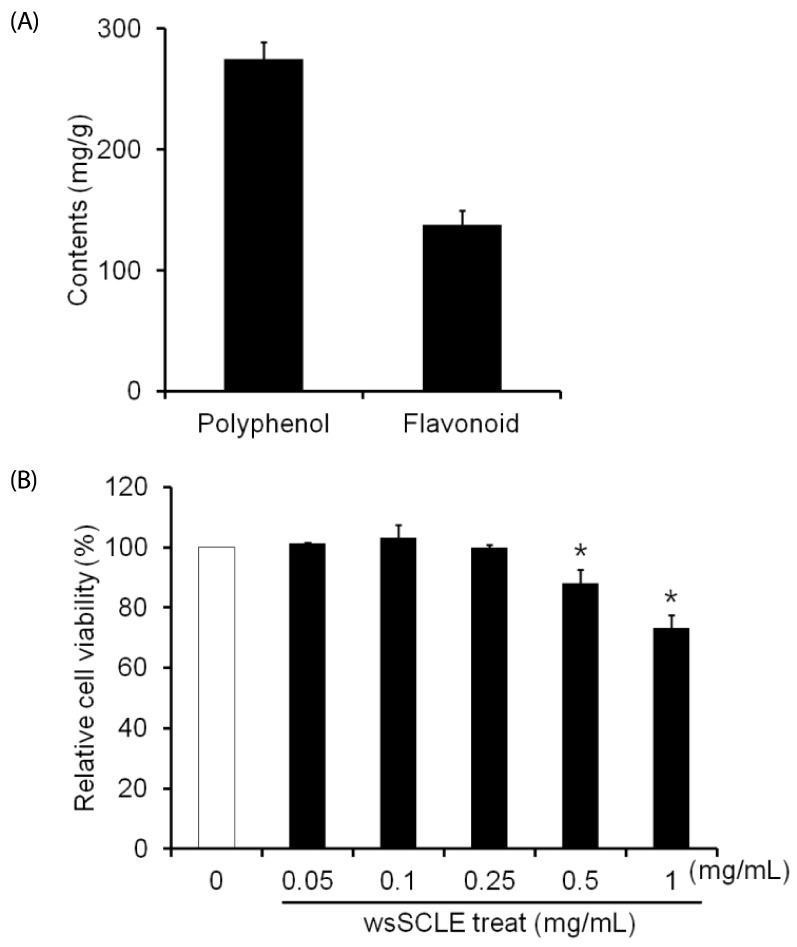
Fig. 2
A water-soluble fraction of Smilax china L. leaf ethanol extract (wsSCLE) treatment inhibits lipid accumulation in 3T3-L1 adipocytes.
Differentiation was induced in 3T3-L1 cells 2 days after they attained confluence. Cells were then treated with wsSCLE (0.1 and 0.25 mg/mL) for 9 days. At the end of the treatment period, lipid content was analyzed using Oil Red O staining. (A) Representative images of Oil Red O staining. (B) Quantification of lipid accumulation based on the optical density values at 520 nm of destained Oil Red O extracted from the 3T3-L1 cells. Data are expressed as mean ± SD of triplicate experiments. #
P < 0.05 compared to control cells (white bar), *
P < 0.05 compared to adipocytes.
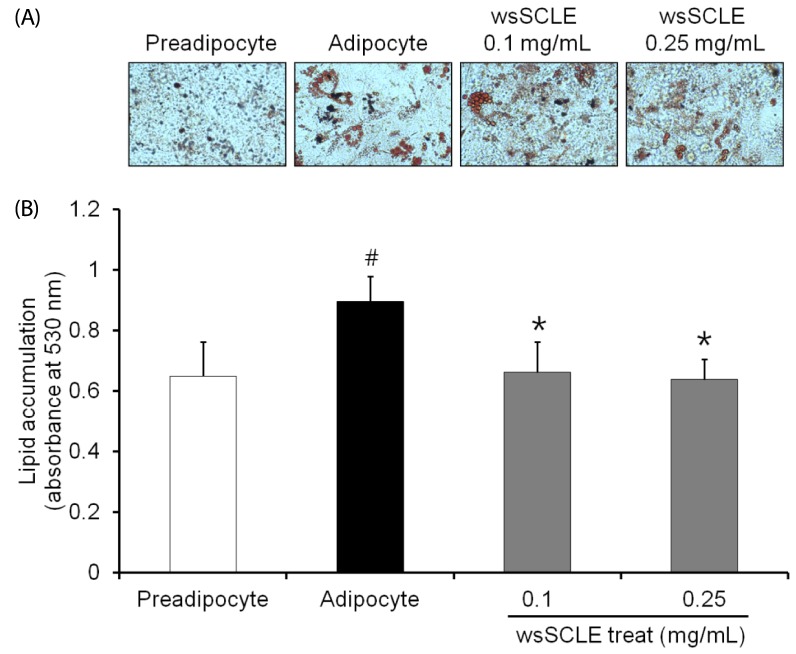
Fig. 3
Effects of a water-soluble fraction of Smilax china L. leaf ethanol extract (wsSCLE) treatment on lipolysis in mature 3T3-L1 adipocytes.
(A) wsSCLE (0.1 and 0.25 mg/mL) increased glycerol release from differentiated mature 3T3-L1 cells. (B) Lipolytic effect of wsSCLE was inhibited by treatment with the protein kinase A (PKA) inhibitor (H89, 10 µM). After differentiation, the mature 3T3-L1 cells were incubated with wsSCLE. Glycerol release was measured using a commercial glycerol assay kit. Data are expressed as mean ± SD of triplicate experiments, #
P < 0.05 compared to control cells (white bar), *
P < 0.05 compared to wsSCLE 0.25 mg/mL.
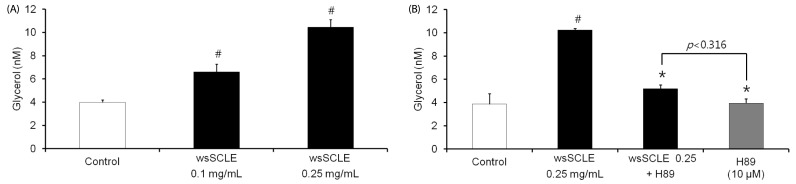
Fig. 4
A water soluble fraction of Smilax china L. leaf ethanol extract (wsSCLE) stimulates protein kinase A (PKA) signaling.
(A) The expression of adenylate cyclase (AC) and PKA by wsSCLE treatment. Mature 3T3-L1 adipocytes were incubated with wsSCLE (0.1 and 0.25 mg/mL) for 24 h. The β-adrenergic receptors were induced (by Iso, an agonist) and inhibited (by Pro, an antagonist). (B) The induction of the phosphorylation (p) of PKA substrates (PKAs) by wsSCLE treatment. H89 treatment (10 µM) decreased wsSCLE-induced pPKAs. Data are expressed as mean ± SD of triplicate experiments, ##
P < 0.001 compared to Iso, *
P < 0.05 compared to control (white bar), ¶¶
P < 0.001 compared to wsSCLE 0.25 mg/mL. Iso, isoproterenol (10 µM); Pro, propranolol (100 µM).
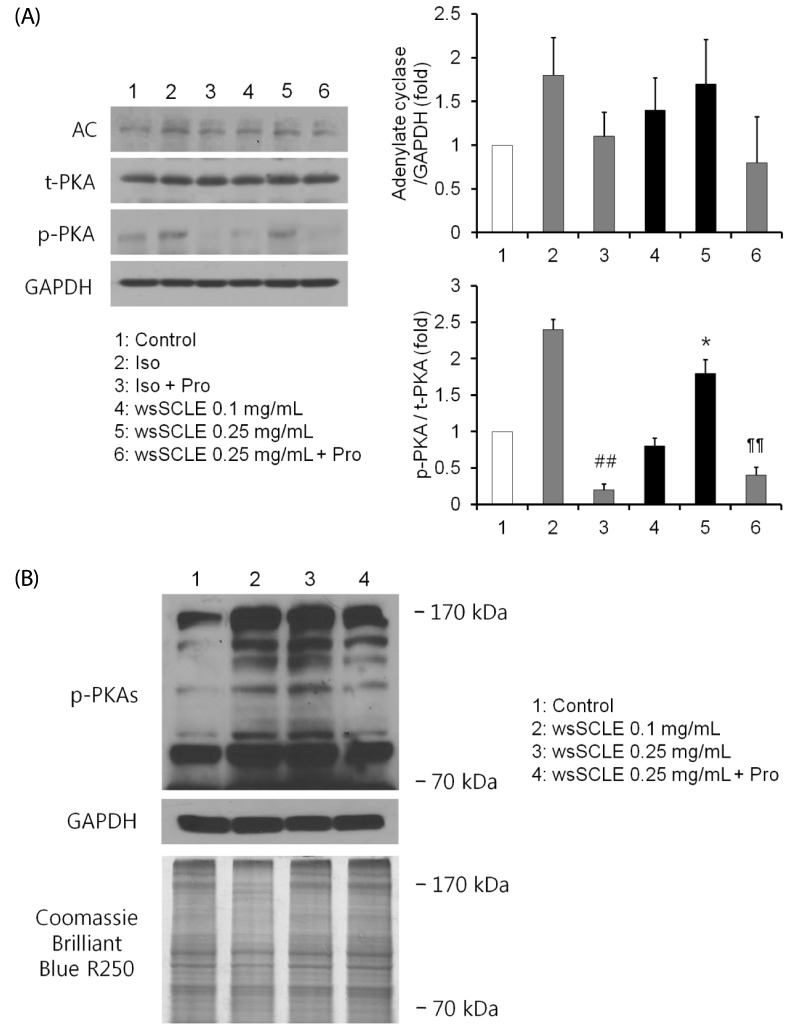
Fig. 5
A water-soluble fraction of Smilax china L. leaf ethanol extract (wsSCLE) induces lipolysis via activation of the cyclic adenosine monophosphate (cAMP) signaling.
Mature 3T3-L1 adipocytes were incubated with wsSCLE (0.1 and 0.25 mg/mL) for 24 h. A β-adrenergic receptor agonist and antagonist (Iso and Pro, respectively) were used as cAMP inducer and inhibitor, respectively. Intracellular cAMP levels were measured using an enzyme-linked immunosorbent assay (ELISA). Data are expressed as mean ± SD of triplicate experiments. *
P < 0.05, **
P < 0.001. Iso, isoproterenol (10 µM); Pro, propranolol, (100 µM).
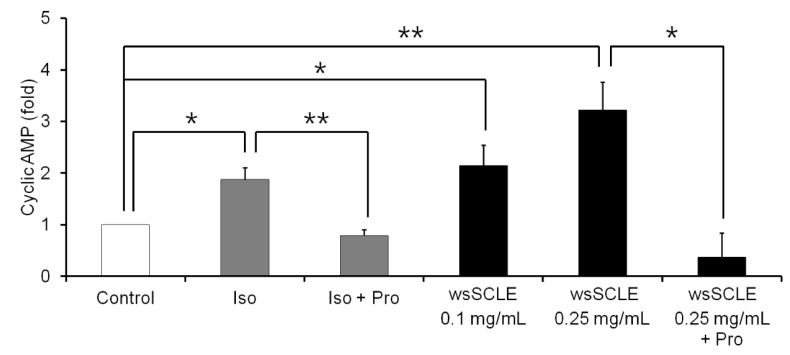
Fig. 6
A water-soluble fraction of Smilax china L. leaf ethanol extract (wsSCLE) induces phosphorylation of hormone-sensitive lipase (HSL).
Mature 3T3-L1 adipocytes were incubated with wsSCLE (0.1 and 0.25 mg/mL) for 24 h. The β-adrenergic receptor agonist and antagonist (Iso and Pro, respectively) were used to induce phosphorylation and dephosphorylation, respectively, of HSL. Data are expressed as mean ± SD of triplicate experiments, ##
P < 0.001 compared to Iso,*
P < 0.05 compared to control, ¶
P < 0.05 compared to wsSCLE 0.25 mg/mL. Iso, isoproterenol (10 µM); Pro, propranolol (100 µM).
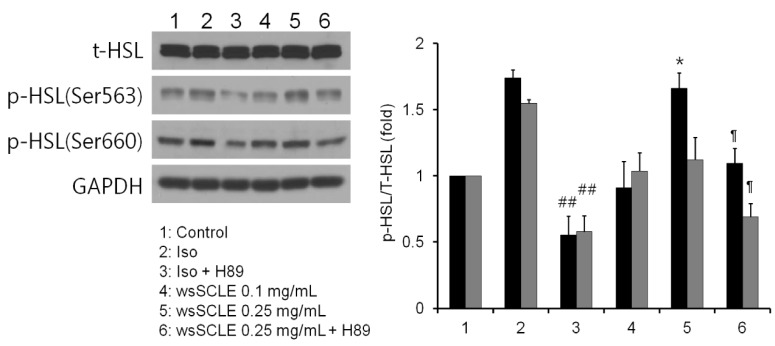