Abstract
BACKGROUND/OBJECTIVES
Citrus flavonoids have a variety of physiological properties such as anti-oxidant, anti-inflammation, anti-cancer, and anti-obesity. We investigated whether bioconversion of Citrus unshiu with cytolase (CU-C) ameliorates the anti-adipogenic effects by modulation of adipocyte differentiation and lipid metabolism in 3T3-L1 cells.
MATERIALS/METHODS
Glycoside forms of Citrus unshiu (CU) were converted into aglycoside forms with cytolase treatment. Cell viability of CU and CU-C was measured at various concentrations in 3T3L-1 cells. The anti-adipogenic and lipolytic effects were examined using Oil red O staining and free glycerol assay, respectively. We performed real time-polymerase chain reaction and western immunoblotting assay to detect mRNA and protein expression of adipogenic transcription factors, respectively.
RESULTS
Treatment with cytolase decreased flavanone rutinoside forms (narirutin and hesperidin) and instead, increased flavanone aglycoside forms (naringenin and hesperetin). During adipocyte differentiation, 3T3-L1 cells were treated with CU or CU-C at a dose of 0.5 mg/ml. Adipocyte differentiation was inhibited in CU-C group, but not in CU group. CU-C markedly suppressed the insulin-induced protein expression of CCAAT/enhancer-binding protein α (C/EBPα) and peroxisome proliferator-activated receptor gamma (PPARγ) as well as the mRNA levels of CEBPα, PPARγ, and sterol regulatory element binding protein 1c (SREBP1c). Both CU and CU-C groups significantly increased the adipolytic activity with the higher release of free glycerol than those of control group in differentiated 3T3-L1 adipocytes. CU-C is particularly superior in suppression of adipogenesis, whereas CU-C has similar effect to CU on stimulation of lipolysis.
Obesity is a severe health problem worldwide and associated with a insulin resistance and metabolic syndromes [1]. It is positively correlated with the occurrence of hyperlipidemia, diabetes mellitus, hypertension and cancer [2]. Adipocytes are characterized as a energy storage in the body and release various adipokines which modulate various signal pathways in adipose tissues and muscles [3]. Adipocyte differentiation is associated with changes in cell morphology, hormone sensitivity and gene expression [4]. During differentiation, the action of several transcription factors including CCAAT/enhancer-binding proteins (C/EBPs) and peroxisome proliferator-activated receptor γ (PPARγ), activates adipogenesis [56]. The C/EBPβ and C/EBPδ allow adipogenesis to proceed by inducing the expression of C/EBPα and PPARγ [7]. Sterol regulatory element binding protein 1 (SREBP1) is another transcription factor regulating later step of adipocyte differentiation and expression of genes affiliated with both lipid metabolism and cholesterol metabolism [89].
Citrus fruit peels have plenty of flavonoids among the phytochemicals and they have been used for centuries to improve bronchial and asthmatic conditions in Asian medicine and prevent cancer, inflammatory and degenerative diseases [1011]. Citrus flavonoids have been shown to improve glucose tolerance, decrease plasma lipid levels and obesity [12]. According to their molecular structures, citrus flavonoids are commonly classified as the six groups; flavones, flavanones, flavonols, isoflavones, antocyanins and flavanols [13]. Flavanones are the main forms in citrus flavonoids and they are much richer in citrus peels and seeds than in citrus tissues. Citrus flavanones occur as glycosides (naringin, narirutin, hesperidin) and aglycoside (naringenin, hesperetin) forms [14]. All these flavonones are found in plasma and excretion. The aglycoside forms such as naringenin and hesperetin are more effectively absorbed than glycoside forms [15], resulting in higher concentrations of aglycoside forms in plasma, urine and bile [14].
The peels of Citrus unshiu, which are byproducts of manufacturing processes of juices, jams, jellies, and other fruit preserves, contain many flavanones. Some flavanone glycosides are cleaved off by intestinal enzymes, and indeed the aglycosides are then rapidly absorbed and subsequently glucuronidated, causing faster absorption and higher concentrations in the body. The ones that are not hydrolysed are poorly absorbed; they pass through the digestive system until they reach the large intestine where they are degraded by the intestinal microflora. In addition, flavanone aglycoside forms exert enhanced biological activity [14]. For example, fermentation of ginseng extracts with β-glucosidase or cytolase converted substantial amounts of ginsenoside forms, leading to magnified anti-inflammatory effect in murine macrophages [16] and anticancer activity in human colon adenocarcinoma [17]. Therefore, bioconversion of citrus peel extracts with cytolase, a pectolytic mixture of various glycosidases extracted from Aspergillus niger, is thought to confer more active compounds for anti-obesity and its related metabolic syndromes.
Although the anti-obesity effect of citrus flavonoids has been gradually established, citrus peel extracts of flavonoids after bioconversion have not been evaluated yet. In this study, we investigated whether Citrus unshiu peel extracts after bioconversion with cytolase enhanced the anti-adipogenic effects by analyzing lipid accumulation, adipogenesis-related genes expression and lipolysis in 3T3-L1 cells.
Citrus unshiu peel was supplied by Institute of BK Bio Co. in Jeju Island, South Korea. The Citrus unshiu peel extracts (CU) and Citrus unshiu peel extracts after bioconversion with cytolase PCL5 (DSM food specialties, Heerlen, Netherlands) (CU-C) were supplied by BK Bio Co., Ltd. (Seongnam, South Korea). Briefly, dried Citrus unshiu peel (100 g) with 0.5% (w/w) cytolase PCL5 (190 units/g) was extracted in 2,000 ml of d-H2O by incubation at pH 4.0, 60℃ for 14 h and enzyme-treated peels were filtered using filter paper with 6 µm pore size (No. 3. Whatman, Little Chalfont, England). Filtered elutes were extracted in 8,000 ml of 80% ethanol at 60℃ for 8 h and then evaporated to freeze-dried powder. The flavonoid compositions of CU and CU-C were analyzed by high-performance liquid chromatography (HPLC; YL9100HPLC, Younglin, Anyang, Korea) and separated on Eclipse plus C18 column (4.6 mm × 250 mm; Agilent Co., Santa Clara, CA, USA). The UV spectrum was recorded at 280 nm with internal standards such as narirutin, naringin, naringenin, hesperidin and hesperetin (Sigma Aldrich Co., St. Louis, MO, USA).
3T3-L1 preadipocytes were purchased from the American Type Culture Collection (ATCC, Rockville, MD, USA). Cells were cultured in Dulbecco's Modified Eagle's Medium (DMEM; Gibco, Grand Island, NY, USA) containing 10% calf serum (Gibco) and 100 U/ml penicillin-streptomycin, (PS; Lonza, Walkersville, MD, USA) at 37℃ with humidified air containing 5% CO2. Adipocyte differentiation was induced DMEM containing 10% fetal bovine serum (FBS; Gibco), 10 µg/ml insulin (Sigma), 1 µM dexamethasone (Sigma) and 0.5 µM 3-isobutyl-1-methylxanthine (Sigma) for 2 days and then cultured in DMEM containing 10% FBS, 100 U/ml PS, and 10 µg/ml insulin for 8 days. CU and CU-C were added into the medium for every 2 days during 10-day differentiation. Sinetrol (Fytexia, Vendres, France) is a polyphenolic mixture of flavanones, anthocyanins and caffeine as patented fat burning supplement. Since the general composition of Sinetrol is expected to be very similar to that of CU, we used Sinetrol as a positive control [18].
3T3-L1 cells (0.05 × 106/well) were cultured in 24-well plates with Sinetrol, CU or CU-C at doses of 0-0.5 mg/ml. The medium was replaced with 3-(4,5-dimethylthiazol-2-yl)-2,5-diphenyltetrazolium bromide (MTT; Amresco, Solon, OH, USA) dye solution [5 mg/ml in phosphate-buffered saline (PBS)] for 4 h at 37℃. The supernatant was decanted and the formazan salts were dissolved with 500 µl of isopropanol. Absorbance was measured at 570 nm using a spectrophotometer (Gen5.2; Biotek, Winooski, VT, USA).
3T3-L1 cells (0.3 × 106/well) were cultured in 6-well plates and treated with Sinetrol, CU or CU-C at a dose of 0.5 mg/ml in differentiation medium for every 2 days during 10-day differentiation. After differentiation, cells were washed with PBS, fixed with 10% formaldehyde (Merck, Darmstadt, Germany) in PBS for 1 h, and then stained with 60% Oil red O dye (Sigma). The stained lipid droplets were monitored under a microscope (Nikon, Tokyo, Japan). The developed chromogens were dissolved with isopropanol and determined at 520 nm using a spectrophotometer (Gen5.2, Biotek).
Isolation of total RNA was performed using the Trizol reagent (Invitrogen, Carlsbad, CA, USA). Total RNA (2 µg) was reverse-transcribed using reverse transcription master premix (ELPIS, Eaejeon, Korea). Real time-PCR (LightCycler, Roche Diagnostics, Basel, Switzerland) was performed with the following primers: mouse C/EBPα sense 5'-CAAGAGCCGAGATAAAGC-3', antisense 5'-TCATTGTCACTGGTCAACT-3'; mouse PPARγ sense 5'-CACCGAGATTTCCTTCAAACT-3', antisense 5'-TATGATGCTCTTCACCTTCCT-3', mouse SREBP1c sense 5'-TGTAGGTCACCGTTTCTTTG-3', antisense 5'-TCGCTCTAGGAGATGTTCA-3'; β-actin sense 5'-GGGAAGGTGACAGCATTG-3', and antisense 5'-GGAGTTGCTGTTGAAGTC-3'. The PCR results were normalized against results obtained with β-actin primers.
Whole cell lysates (20 µg protein each) were resolved by 10% SDS-PAGE, transferred to a polyvinylidene difluoride membrane (Roche Diagnostics), and probed with primary antibodies specific to C/EBPα, PPARγ or β-tubulin (Santa Cruz Technology, Dallas, TX, USA). After washing, membranes were incubated with horseradish peroxidase conjugated secondary antibody (Santa Cruz). Immunodetection was performed using a chemiluminescence method (Super Signal; Pierce Biotechnology, Rockford, IL, USA) and then normalized with β-tubulin.
Fully differentiated 3T3-L1 cells were treated with the presence or absence of Sinetrol, CU or CU-C at a dose of 0.5 mg/ml for 24 h. Free glycerols were quantified in the cell culture medium using free glycerol assay reagent according to the manufacturer's instruction (Cayman, Ann Arbor, MI, USA). Briefly, the glycerol standards at doses of 0-125 µg/ml and each supernatant was transferred into a 96-well plate and 100 µl of free glycerol assay reagent was added. Samples were incubated for 15 min and the absorbance was measured at 540 nm using a spectrophotometer (Gen5.2; Biotek).
For all experiments, statistical analysis using IBM SPSS statistics programs (Ver. 20; Armonk, NY, USA) was performed by Student's t-test and one way ANOVA followed by post-hoc analysis with the Scheffe's test to detect differences between experimental groups. A value of P < 0.05 was considered statistically significant.
The flavonoid contents using HPLC chromatogram showed that CU and CU-C have 23.89% and 30.06% of total flavonoids. Cytolase, a mixture of various glycosidases, increased aglycosides by hydrolysis of rutinoses (dissacharide of rhamnose and glucose) from flavanone rutinosides (Table 1). There were substantial bioconversions of narirutin to naringenin and of hesperidin to hesperetin, respectively. Narirutin decreased from 3.45% in CU to 1.62% in CU-C and instead, naringenin increased from 0.02% in CU to 6.23% in CU-C. Also, hesperidin decreased from 20.37% in CU to 15.5% in CU-C, whereas hesperetin increased from 0.05% in CU to 6.63% in CU-C (Table 2). Naringin, neohesperidoside form, was negligible in both CU and CU-C.
To examine the anti-adipogenic effect of CU and CU-C on cell proliferation, we first evaluated the cytotoxicity of CU and CU-C in 3T3-L1 preadipocytes by treatment with various concentrations (0-0.5 mg/ml) of Sinetrol (a positive control), CU or CU-C. No inhibitory effects on cell viability were observed in cells treated with up to 0.5 mg/ml of Sinetrol, CU or CU-C, meanwhile some lower doses of Sinetrol and CU showed significantly higher cell viability than untreated preadipocytes (Fig. 1).
Next, we examined the effect of CU and CU-C on adipocyte differentiation. Adipocyte differentiation was induced with the differentiation medium containing MDI cocktail supplemented with Sinetrol, CU or CU-C at a dose of 0.5 mg/ml for every 2 days until fully differentiation. Lipid accumulations were assessed by Oil red O staining. The triglyceride level in differentiated adipocytes by insulin (a negative control) was about 3.5-fold increased, compared to preadipocyte group. CU-C at a dose of 0.5 mg/ml inhibited adipogenesis despite of no effects of Sinetrol and CU at the same dose treatment (Fig. 2).
To determine the molecular mechanisms of adipogenesis inhibition of CU and CU-C, we investigated the gene expression of adipogenic transcription factors, C/EBPα, PPARγ, and SREBP1c. As shown in Fig. 3, those genes were significantly increased in control group. Compared to control group, Sinetrol and CU at a dose of 0.5 mg/ml tended to decrease adipogenic transcription factors but did not significantly affect the genes expression of C/EBPα and SREBP1c (Fig. 3). CU at a dose of 0.5 mg/ml had significant effect on PPARγ (Fig. 3A) and much higher doses (1-1.5 mg/ml) of Sinetrol and CU significantly suppressed all these adipogenic genes (data not shown). However, CU-C at a dose of 0.5 mg/ml markedly suppressed these three adipogenic transcription factors, compared to groups of control, Sinetrol and CU (Fig. 3A).
Furthermore, we evaluated the protein expression of these transcription factors by western blot analysis. C/EBPα and PPARγ were significantly induced during adipocyte differentiation, whereas they were negligible in undifferentiated 3T3-L1 cells. Although Sinetrol and CU at a dose of 0.5 mg/ml tended to downregulate C/EBPα and PPARγ expression compared to control group but only CU-C exhibited markedly suppressive effect on protein expression of C/EBPα and PPARγ (Fig. 3B).
To explore the lipolytic activity of citrus flavonoids, we analyzed the free glycerol levels in adipocytes after differentiation. Sinetrol increased free glycerol release by 2-fold compared to control group. In addition, CU and CU-C at a dose of 0.5 mg/ml increased glycerol secretion by 162% and 166%, respectively, which means that CU and CU-C triggered lipolysis in 3T3-L1 adipocytes (Fig. 4). However, bioconversion with cytolase didn't make any significant difference between CU and CU-C.
In this study, we compared the anti-adipogenic effect of Citrus unshiu peel extracts before and after bioconversion with cytolase. CU-C decreased narirutin and hesperidin indicating that cytolase, a mixture of various glycosidases, deglycosylated certain citrus flavonoid glycosides, especially flavanone rutinoside forms and instead, increased naringenin and hesperetin (Table 2).
Treatment of 3T3-L1 preadipocytes with cytolase-treated CU significantly suppressed adipocyte differentiation and its relevant transcription factors, suggesting that cytolase treatment seemed to enhance bioactivity of CU. We demonstrated that bioconversion of Citrus unshiu peel extracts with cytolase increased inhibition of lipid accumulation and suppression of adipocyte-specific transcription factors, including C/EBPα, PPARγ, and SREBP1c in 3T3-L1 cells. Although all of citrus flavonoids significantly increased lipolysis in differentiated 3T3-L1 cells, deglycosylation of Citrus unshiu peel extracts did not lead to an increased free glycerol release, i.e., lipid lipolysis. These results suggest that bioconversion of Citrus unshiu peel extracts with cytolase which causes formation of aglycoside flavonoids, enhances their anti-adipogenic effects by suppression of lipid accumulation rather than by activation of lipolysis.
HPLC analysis demonstrated that treatment of Citrus unshiu peel extracts with cytolase catalyzed hydrolysis of flavanone rutinoside forms (narirutin and hesperidin) into aglycosides (naringenin and hesperetin) and rutinose (rhamnosyl-α-1,6-glucose) (Table 1 & 2). This effect is similar to that of β-glucosidase in the gastrointestinal tract. β-glucosidase, which catalyzes the hydrolysis of β-glucosidic bonds, especially terminal non-reducing residues in D-glucosides with release of glucose. Therefore, its action deglycosylates various flavonoid glycosides, especially flavanone rutinosides in citrus products. Since aglycosides are absorbed faster than glycosides [15], aglycosides are more detected than glycosides in plasma, urine, and bile [14]. In addition to bioavailability, the removal of the rhamnose improves the bioactivity of glycoside forms. However, naringin, the neohesperidoside form (rhamnosyl-α-1,2-glucose) of naringenin, was not detectable in Citrus unshiu peel extracts, suggesting that naringin is not major flavonoid form in Citrus unshiu. Since Sinetrol was reported to have a strong lipolytic effect by inhibition of cAMP-phosphodiesterase (PDE) in human adipocytes and obese humans, we used Sinetrol as a positive control. Sinetrol is a polyphenolic mixture of flavanones (expressed as mainly naringin), anthocyanins and caffeine [18].
Naringin, a flavanone neohesperidoside, has a bitter taste while narirutin and hesperidin, flavanone rutinosides, have no bitter taste [14]. A bitter taste of Sinetrol is associated with large composition of naringin, causing difficulty in diverse applications of industrial products except for pills. Since, Citrus unshiu peel extracts before and after bioconversion have negligible amounts of naringin, Citrus unshiu peel extracts have a less bitter taste even in sensory analysis (data not shown).
A suppression of lipid accumulation is associated with the inhibition of adipogenesis along with a reduction of adipocyte numbers (hyperplasia) and triglyceride accumulation (hypertrophy) of adipocytes. Citrus aurantium flavonoids induced the downregulation of lipid accumulation [19]. CU-C displayed anti-adipogenic property, which was indicated by the decreased levels of Oil red O staining (Fig. 2). These results suggest that bioconversion induced more inhibitory effect on adipogenesis, by showing that low dose of CU-C completely restrained lipid accumulation.
Lipid homeostasis may be controlled by two reciprocal processes, lipogenesis (including adipocyte differentiation and lipid accumulation) and lipolysis in adipocytes. In the metaphase of adipocyte differentiation, C/EBPα, PPARγ and SREBP1c are key transcription factors [20]. For example, since PPARγ, the key transcription regulator for lipid and carbohydrate metabolisms, is activated by hesperetin or naringenin [21], over-expression of PPARγ and C/EBPα expedited adipogenesis and PPARγ-deficient cells hindered adipocyte differentiation [22]. In the analysis of adipogenic gene expression, 0.05% of CU-C (Fig. 3A & 3B) and ≥ 0.1% Citrus flavonoids (data not shown) prevented adipocyte differentiation and lipid accumulation by suppressing mRNA and protein levels of C/EBPα, PPARγ, and/or SREBP1c, compared to those in fully differentiated adipocytes. Several studies have demonstrated that citrus inhibited C/EBPα, PPARγ, and/or SREBP1c expression [789], which is consistent with our study at high dose of CU before bioconversion and even at low dose of CU-C after bioconversion with cytolase. Unfortunately, commercially unavailable SREBP1c antibody did not permit convincing analysis of citrus peel extracts effects on SREBP1c protein expression, which may be responsible in part for less adipocyte differentiation in CU-C treated group (Fig. 2). Another possible mechanism for the suppression of adipogenesis is structural changes. In regard to structural requirements of flavonoids for adipogenesis, fewer hydroxyl groups and more methoxy groups particularly at the 3-position on the B-ring are needed [23]. Irrespective of methoxy group, bioconversion with cytolase replaced rutinose and/or neohesperidose of flavanones with a hydroxyl group [14] (Table 1). This addition of free hydroxyl group might be partly responsible for suppression of adipogenesis [23]. Therefore, these results suggest that bioconversion of CU with cytolase suppresses adipocyte differentiation and lipid accumulation by downregulation of C/EBPα, PPARγ, and SREBP1c at the molecular levels and that enhances capacity for inhibition of adipocyte differentiation and lipid accumulation.
The other important factor of lipid homeostasis is lipolysis. Lipolysis is a catabolic process causing break-down of triglyceride into glycerol and free fatty acids. In our study, all of CU and Sinetrol significantly increased glycerol release compared to control group, implying that CU accelerated lipolysis in mature adipocytes. Recently Citrus Sunki peel extract was shown to have anti-obesity effect via elevated β-oxidation and lipolysis in adipose tissue [11]. Citrus aurantium L. extracts exhibited lipolytic activity by reducing body fat mass in human adipocytes and obese humans, respectively [2425]. We employed Sinetrol as a positive control and it had slightly higher but the same lipolytic effect as much as CU and CU-C did. Sientrol was reported to have a strong lipolytic activity with elevated free fatty acid release by cAMP-PDE inhibition, which may be attributed to the synergetic effects of polyphenol composition of Sinetrol (flavanones, anthocyanins and caffeine) [18]. Sinetrol is obtained by crushing of citrus fruits and cold pressure of juice [18]. The general composition of Sinetrol is expected to be very similar to that of CU; this fact is reflected in this study by showing similar results for Sinetrol and CU. However, in spite of more potent capacity of CU-C in anti-adipogenesis, there was no significant difference of lipolysis between CU and CU-C. The more potent anti-adipogenic capacity of CU-C may be mainly induced by inhibitory lipogenesis of transcription factors but not by stimulatory lipolysis after adipocyte differentiation.
In conclusion, we evaluated the effects of the natural compounds and its bioconverted compounds on the anti-adipogenesis. CU-C inhibited adipocyte differentiation and lipid accumulation by modulating the mRNA and protein expression of transcription factors such as C/EBPα, PPARγ, and SREBP1c and by increasing lipolysis. Particularly CU-C is superior in suppression of adipogenesis rather than elevation of lipolysis, compared to Sinerol and CU. Taken together, bioconversion of citrus flavonoids with cytolase significantly increases the level of flavanone aglycosides, which have an increased anti-adipogenic activity compared to flavanone glycosides.
Figures and Tables
Fig. 1
Effects of Sinetrol, CU and CU-C on cell viability.
3T3-L1 cells were treated with different concentrations (0-0.5 mg/ml) of Sinetrol, CU or CU-C for 24 h. Sinetrol, a positive control. Each bar represents the mean ± SD (n = 3). * P < 0.05 and # P < 0.01 versus untreated preadipocyte.
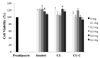
Fig. 2
Effects of Sinetrol, CU and CU-C at a dose of 0.5 mg/ml on lipid accumulation in 3T3-L1 cells.
Sinetrol, a positive control. Each bar represents the mean ± SD (n = 3). Different letters mean significant difference according to ANOVA, Scheffe's test (P < 0.05).
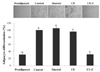
Fig. 3
Effects of Sinetrol, CU and CU-C at a dose of 0.5 mg/ml on the adipogenic genes expression in 3T3-L1 cells. 3T3-L1 preadipocytes were treated with Sinetrol, CU or CU-C at a dose of 0.5 mg/ml for 10 days in differentiation medium.
(A) mRNA levels of C/EBPα, PPARγ, and SREBP1c. Real time-PCR was performed using gene specific primers. (B) Protein expression of C/EBPα and PPARγ. Immunoblotting was performed with specific antibodies. Pre: preadipocyte; Sin: Sinetrol. Each bar represents the mean ± SD (n = 3). Different letters mean significant difference according to ANOVA, Scheffe's test (P < 0.05).

Fig. 4
Effect of Sinetrol, CU and CU-C at a dose of 0.5 mg/ml on lipolytic activity in 3T3-L1 adipocytes.
The differentiated 3T3-L1 adipocytes were cultured in the presence or absence of Sinetrol, CU or CU-C at a dose of 0.5 mg/ml for 24 h. Cell culture supernatants were assayed for free glycerol release. Each bar represents the mean ± SD (n = 3). Different letters mean significant difference according to ANOVA, Scheffe's test (P < 0.05).
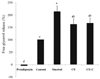
References
2. Ailhaud G, Guesnet P, Cunnane SC. An emerging risk factor for obesity: does disequilibrium of polyunsaturated fatty acid metabolism contribute to excessive adipose tissue development? Br J Nutr. 2008; 100:461–470.


3. Kershaw EE, Flier JS. Adipose tissue as an endocrine organ. J Clin Endocrinol Metab. 2004; 89:2548–2556.


4. Park HS, Kim SH, Kim YS, Ryu SY, Hwang JT, Yang HJ, Kim GH, Kwon DY, Kim MS. Luteolin inhibits adipogenic differentiation by regulating PPARγ activation. Biofactors. 2009; 35:373–379.


5. Farmer SR. Regulation of PPARgamma activity during adipogenesis. Int J Obes (Lond). 2005; 29:Suppl 1. S13–S16.
7. Zuo Y, Qiang L, Farmer SR. Activation of CCAAT/enhancer-binding protein (C/EBP) α expression by C/EBP β during adipogenesis requires a peroxisome proliferator-activated receptor-γ-associated repression of HDAC1 at the C/EBP α gene promoter. J Biol Chem. 2006; 281:7960–7967.


8. Wang X, Briggs MR, Hua X, Yokoyama C, Goldstein JL, Brown MS. Nuclear protein that binds sterol regulatory element of low density lipoprotein receptor promoter. II. Purification and characterization. J Biol Chem. 1993; 268:14497–14504.


9. Kim JB, Spiegelman BM. ADD1/SREBP1 promotes adipocyte differentiation and gene expression linked to fatty acid metabolism. Genes Dev. 1996; 10:1096–1107.


10. Ejaz S, Ejaz A, Matsuda K, Lim CW. Limonoids as cancer chemopreventive agents. J Sci Food Agric. 2006; 86:339–345.


11. Kang SI, Shin HS, Kim HM, Hong YS, Yoon SA, Kang SW, Kim JH, Kim MH, Ko HC, Kim SJ. Immature Citrus sunki peel extract exhibits antiobesity effects by β-oxidation and lipolysis in high-fat diet-induced obese mice. Biol Pharm Bull. 2012; 35:223–230.


12. Nichols LA, Jackson DE, Manthey JA, Shukla SD, Holland LJ. Citrus flavonoids repress the mRNA for stearoyl-CoA desaturase, a key enzyme in lipid synthesis and obesity control, in rat primary hepatocytes. Lipids Health Dis. 2011; 10:36–40.


13. Peterson J, Dwyer J. Flavonoids: dietary occurrence and biochemical activity. Nutr Res. 1998; 18:1995–2018.


14. Tripoli E, Guardia ML, Giammanco S, Majo DD, Giammanco M. Citrus flavonoids: molecular structure, biological activity and nutritional properties: a review. Food Chem. 2007; 104:466–479.


15. Fuhr U, Kummert AL. The fate of naringin in humans: a key to grapefruit juice-drug interactions? Clin Pharmacol Ther. 1995; 58:365–373.


16. Seo JY, Lee JH, Kim NW, Her E, Chang SH, Ko NY, Yoo YH, Kim JW, Seo DW, Han JW, Kim YM, Choi WS. Effect of a fermented ginseng extract, BST204, on the expression of cyclooxygenase-2 in murine macrophages. Int Immunopharmacol. 2005; 5:929–936.


17. Yang G, Park D, Lee J, Song BS, Jeon TH, Kang SJ, Jeon JH, Shin S, Jeong H, Lee H, Kim Y. Suppressive effects of red ginseng preparations on SW480 colon cancer xenografts in mice. Food Sci Biotechnol. 2011; 20:1649–1653.


18. Dallas C, Gerbi A, Tenca G, Juchaux F, Bernard FX. Lipolytic effect of a polyphenolic citrus dry extract of red orange, grapefruit, orange (SINETROL) in human body fat adipocytes. Mechanism of action by inhibition of cAMP-phosphodiesterase (PDE). Phytomedicine. 2008; 15:783–792.


19. Kim GS, Park HJ, Woo JH, Kim MK, Koh PO, Min W, Ko YG, Kim CH, Won CK, Cho JH. Citrus aurantium flavonoids inhibit adipogenesis through the Akt signaling pathway in 3T3-L1 cells. BMC Complement Altern Med. 2012; 12:31.


20. Rayalam S, Della-Fera MA, Baile CA. Phytochemicals and regulation of the adipocyte life cycle. J Nutr Biochem. 2008; 19:717–726.


21. Liu L, Shan S, Zhang K, Ning ZQ, Lu XP, Cheng YY. Naringenin and hesperetin, two flavonoids derived from Citrus aurantium up-regulate transcription of adiponectin. Phytother Res. 2008; 22:1400–1403.


22. Akune T, Ohba S, Kamekura S, Yamaguchi M, Chung UI, Kubota N, Terauchi Y, Harada Y, Azuma Y, Nakamura K, Kadowaki T, Kawaguchi H. PPARγ insufficiency enhances osteogenesis through osteoblast formation from bone marrow progenitors. J Clin Invest. 2004; 113:846–855.


23. Matsuda H, Kogami Y, Nakamura S, Sugiyama T, Ueno T, Yoshikawa M. Structural requirements of flavonoids for the adipogenesis of 3T3-L1 cells. Bioorg Med Chem. 2011; 19:2835–2841.

