Abstract
BACKGROUND/OBJECTIVES
Increased mass of adipose tissue in obese persons is caused by excessive adipogenesis, which is elaborately controlled by an array of transcription factors. Inhibition of adipogenesis by diverse plant-derived substances has been explored. The aim of the current study was to examine the effects of the aqueous methanol extract of laver (Porphyra yezoensis) on adipogenesis and apoptosis in 3T3-L1 adipocytes and to investigate the mechanism underlying the effect of the laver extract.
MATERIALS/METHODS
3T3-L1 cells were treated with various concentrations of laver extract in differentiation medium. Lipid accumulation, expression of adipogenic proteins, including CCAAT enhancer-binding protein α, peroxisome proliferator-activated receptor γ, fatty acid binding protein 4, and fatty acid synthase, cell viability, apoptosis, and the total content and the ratio of reduced to oxidized forms of glutathione (GSH/GSSG) were analyzed.
RESULTS
Treatment with laver extract resulted in a significant decrease in lipid accumulation in 3T3-L1 adipocytes, which showed correlation with a reduction in expression of adipogenic proteins. Treatment with laver extract also resulted in a decrease in the viability of preadipocytes and an increase in the apoptosis of mature adipocytes. Treatment with laver extract led to exacerbated depletion of cellular glutathione and abolished the transient increase in GSH/GSSG ratio during adipogenesis in 3T3-L1 adipocytes.
CONCLUSION
Results of our study demonstrated that treatment with the laver extract caused inhibition of adipogenesis, a decrease in proliferation of preadipocytes, and an increase in the apoptosis of mature adipocytes. It appears that these effects were caused by increasing oxidative stress, as demonstrated by the depletion and oxidation of the cellular glutathione pool in the extract-treated adipocytes. Our results suggest that a prooxidant role of laver extract is associated with its antiadipogenic and proapoptotic effects.
Adipocytes are the main components of white adipose tissue. The primary function of adipocytes is control of energy balance through storage and mobilization of triacylglycerol. Adipose tissue is also an important endocrine organ which plays regulatory roles in glucose metabolism and energy homeostasis. However, excess adipose tissue in obese persons may cause insulin resistance, a critical risk factor in development of serious conditions such as type 2 diabetes, hyperlipidemia, and cardiovascular disease [1]. Increased mass of adipose tissue is caused by an increase in adipogenesis, the process by which preadipocytes are converted to fully differentiated adipocytes, and the accumulation of triacylglycerol in adipocytes [23]. The adipocyte differentiation process is elaborately controlled by an array of transcription factors, including CCAAT enhancer-binding proteins (C/EBPs) and peroxisome proliferator-activated receptor γ (PPAR γ) [45]. These transcription factors coordinate the expression of numerous proteins which establish phenotype of mature adipocytes. 3T3-L1 preadipocyte is a well-characterized cellular model for studying adipogenesis, which can be induced to differentiate into adipocytes by treatment with insulin, dexamethasone, and 3-isobutyl-1-methylxantine [6].
Inhibition of adipogenesis and lipid accumulation, depletion of lipids through lipolysis, and removal of adipocytes through apoptosis can be considered strategies for prevention or treatment of obesity. The complex network of adipogenesis can be affected by many factors which interfere with the adipogenesis process. Diverse plant extracts and plant-derived substances, e.g., phenolic compounds and vitamins, have been reported to have roles in prevention of obesity [7].
A variety of algal species have been reported to contain bioactive compounds which demonstrate antioxidative, anti-inflammatory, antimicrobial, and anticancer effects [8]. Marine algae are known to contain diverse bioactive primary and secondary metabolites, which play critical roles in defending themselves against a harsh environment, such as daily tidal fluctuation, desiccation, and UV-irradiation [9]. Laver, a red alga consumed predominantly in East Asia, is well known as a rich source of mycosporine-like amino acids (MAAs), such as porphyra-334 (P-334) and shinorine, which are known to function as antioxidants [10]. Nonetheless, the MAAs initially attracted attention because of their high UV-absorbing capacity [1112]. Some red algal species are also reported to contain other antioxidant substances such as carotenoids and polyphenols [1314].
The anti-obesity effects of edible seaweeds have mainly been examined using brown algae [151617]. Recently, some red algal species were reported to show an antiadipogenic effect [1819]. However, the antiadipogenic effect of laver, one of the most widely consumed red algae, has not been previously explored.
In the current study, we assessed the effect of the aqueous methanol extract of laver (Porphyra yezoensis) on adipogenesis and apoptosis in 3T3-L1 adipocytes. The effect of laver extract on cellular glutathione (γ-glutamyl-cysteinyl-glycine, GSH), a primary low molecular weight antioxidant, was also examined in order to explore the mechanism of adipogenesis regulation by laver extract.
Dried laver grown and collected from Jangbong Island, Incheon, Korea was used. Ten g of dried laver was extracted with 100 mL of 80% (v/v) methanol for 24 h at 45℃. Aqueous alcohols have been widely used for extraction of secondary plant compounds, most of which are polar compounds with varying degree of polarity [2021]. The mixture was centrifuged (2,000 g, 10 min), and the clear supernatant was evaporated to dryness by rotary evaporator. The dried laver extract was re-dissolved in 20 mL of distilled water, and the solution was vigorously mixed with 160 mL of a mixture of chloroform, methanol, and distilled water (2:1:0.9) in a separating funnel. The aqueous upper layer was dried and redissolved in distilled water and stored in aliquots at -20℃.
The MAA content of laver extract was calculated from the molar absorption coefficient of P-334 at 334 nm, i.e., 42,300 M-1·cm-1 [22]. Phenolic content was measured by Folin-Ciocalteu assay using gallic acid as a standard [23]. The total phenolic content was expressed as gallic acid equivalents (GAE).
3T3-L1 preadipocytes were obtained from the Korean Cell Line Bank (Seoul, Korea). Preadipocyte cells were plated in 6-well plates and grown in DMEM (Gibco Life Technologies, Grand Island, NY, USA) supplemented with 10% bovine serum (BS, Gibco), 100 U/mL penicillin, and 100 µg/mL streptomycin until confluent at 37℃. Two days after reaching confluency (day 0), the preadipocytes were stimulated to differentiate with DMEM containing 10% fetal bovine serum (FBS, Gibco), 5 µg/mL insulin, 0.5 mM 3-isobutyl-1-methylxanthine, and 1 µM dexamethasone for 2 days. On day 2, the medium was changed to DMEM containing 10% FBS and 5 µg/mL insulin. On day 4, the medium was changed to DMEM containing 10% FBS, which was replaced every 2 days.
3T3-L1 cells were incubated with different concentrations of laver extract added to the differentiation medium during the adipogenic process from day 0. At the indicated time points, lipid content in cells was measured by Oil Red O staining [24]. Briefly, the cells were washed with phosphate-buffered saline (PBS), fixed with 10% formalin for 1 h, and stained with Oil Red O for 1 h. The stained cells were washed with PBS and microscopic images were taken. The dye was eluted from cells with isopropanol and the absorbance was measured at 510 nm. The lipid content was normalized to the protein content in each well, which was measured using the Lowry method [25] after solubilization by 1 N NaOH.
On day 9, Western blot was performed to assess the levels of adipogenic marker proteins. Briefly, whole cell lysates were prepared using radioimmunoprecipitation assay (RIPA) lysis buffer (150 mM NaCl, 1% Triton X-100, 0.5% sodium deoxycholate, 0.1% sodium dodecyl sulfate (SDS), 50 mM Tris-HCl, pH 8.0) containing protease/phosphatase inhibitor cocktail. The proteins in cell lysates, with equal amounts of total proteins (10 µg or 20 µg), were separated on a SDS-polyacrylamide gel electrophoresis gel and blotted onto a polyvinylidene fluoride (PVDF) membrane. The blot was blocked with 5% skim milk in PBS with 0.1% Tween 20 (PBST) solution and incubated with appropriate antibodies. Immunoreactive protein bands were detected using a horseradish peroxidase-conjugated secondary antibody and enhanced chemiluminescence reagents. The blot was densitometrically analyzed and each band was normalized to a respective β-actin band using Image J software.
Cell viability was determined by MTT assay. Briefly, 3T3-L1 preadipocytes were treated with different concentrations of laver extract for 48 h in 96-well plates, followed by addition of 10 µL of 5 mg/mL 3-(4, 5-dimethylthiazolyl-2)-2, 5-diphenyltetrazolium bromide (MTT) solution to each well and incubation for 4 h at 37℃. After removal of medium, the formazan product was solubilized in 200 µL of DMSO and the absorbance was measured at 560 nm.
On day 7, either preadipocytes or differentiated adipocytes were treated with laver extract for 48 h. The cells were collected and stained with annexin V-fluorescein isothiocyanate (FITC) and propidium iodide (PI) using the FITC Annexin V Apoptosis Detection kit (BD Pharmingen; San Diego, CA, USA) according to the supplier's instructions. Viable and dead cells were detected using a Gallios™ Flow Cytometer with Kaluza Flow Cytometry Analysis Software (Beckman Coulter, IN, USA).
Cellular glutathione was analyzed by HPLC method using fluorescence detection after precolumn derivatization with o-phthalaldehyde (OPA) [26]. Briefly, to determine the total glutathione content, i.e., the sum of reduced (GSH) and oxidized (GSSG) forms, a 5% perchloric acid (PCA) extract of the cells was neutralized and then reduced by 2.5 mM dithiothreitol. The reduced sample was derivatized with OPA as described previously [27] and injected onto a Supelcosil™ LC-18 column (particle size 5 µm, 25 cm × 4.6 mm; Supelco, Bellefonte, PA, USA). The GSH-OPA adduct was eluted using a methanol gradient in a 0.1 M sodium phosphate buffer, pH 5.5, and detected by monitoring fluorescence at an excitation wavelength of 350 nm and an emission wavelength of 420 nm. To determine GSSG content, a duplicate PCA extract reacted with 2 mM N-ethylmaleimide to eliminate GSH was used. GSH content was calculated from the difference between the amount of total glutathione and GSSG. Total glutathione content was expressed as GSH equivalents in nmol/mg protein. The redox status of cellular glutathione was presented as GSH/GSSG ratio. The PCA-precipitated protein pellet was solubilized by 1 N NaOH, and protein content was measured using the Lowry method [25].
The yield from the 80% methanol extraction of dried laver was 11% (w/w). The content of phenolic compounds in laver extract was 33 mg GAE/g dried extract. The absorption spectrum of the aqueous solution of laver extract showed λmax at 334 nm, which is a characteristic of P-334 or shinorine, the MAAs [11]. Total MAA content in laver extract was 120 mg/g dried extract, as calculated using a molar extinction coefficient of P-334.
3T3-L1 cells were treated with various concentrations of laver extract in the differentiation medium from day 0 to day 9. On day 9, the content of accumulated lipid was determined by Oil Red O staining. Oil Red O is a fat-soluble dye, which stains neutral triglycerides. Treatment with the laver extract resulted in a significant dose-dependent reduction in lipid accumulation (Fig. 1), although the effective concentrations of the laver extract were higher than those of other red algal extracts reported in the literature [1819]. The levels of accumulated lipid were 58.5 ± 5.3% (P < 0.001), 27.8 ± 8.4% (P < 0.001), and 23.7 ± 6.7% (P < 0.001) of the control level (at 0 mg/mL) at 5, 10, and 15 mg/mL of laver extract concentration, respectively (Fig. 1B). The lipid content was normalized to the protein content in each well so that the percentage represents relative lipid content per mg of protein.
To verify that the decrease in lipid accumulation shown in Fig. 1 was due to downregulation of adipogenic proteins, PPARγ and C/EBPα, the key transcription factors of adipocyte differentiation, and fatty acid binding protein 4 (FABP4) and fatty acid synthase (FAS), the representative adipocyte-specific proteins, were measured by Western blot. As shown in Fig. 2, laver extract caused decreased expression of all adipogenic proteins. In particular, the levels of C/EBPα, FABP4, and FAS were almost completely reduced at concentrations of laver extract higher than 10 mg/mL.
Treatment with laver extract resulted in a significant dose-dependent decrease in the viability of preadipocytes. The viabilities of cells treated with 5, 10, and 15 mg/mL of laver extract for 48 h were reduced to 84.3 ± 16.9% (P < 0.005), 67.7 ± 12.5% (P < 0.001), and 50.3 ± 7.1% (P < 0.001) of the control level (at 0 mg/mL), respectively (Fig. 3A). The concentrations of laver extract used for treatment in this study were chosen based on this MTT result in order to examine its effects not only on adipogenesis but also on cell proliferation and death.
An apoptosis assay using flow cytometry with annexin V-FITC and PI double labeling was performed to examine whether the decrease in the viability of laver extract-treated preadipocytes shown in Fig. 3A was associated with the increase in cell death. Our results indicated that treatment with laver extract did not cause significant changes in either apoptotic or necrotic cell death in preadipocytes (Fig. 3B & C).
Contrary to the preadipocytes, the differentiated adipocytes exhibited significantly increased apoptosis by treatment with laver extract for 48 h (during days 7 to 8) at concentrations of 10 mg/mL and above (Fig. 4). However, necrotic cell death was not affected by treatment with laver extract. It is possible that the observed necrotic cell death, in either preadipocytes or adipocytes, could have occurred during the process of cell preparation for the assay.
To investigate a mechanism of the antiadipogenic and proapoptotic effects of laver extract, cellular glutathione level was monitored during the differentiation process. 3T3-L1 cells were treated with various concentrations of laver extract in the differentiation medium, and on days 0, 3, 6, and 9, the total content and redox status of glutathione (GSH/GSSG ratio) were determined.
In control adipocyte cells, i.e., cells without extract treatment, a continuous decrease in total glutathione content was demonstrated after day 3 (Fig. 5A). Conversely, a remarkable but transient increase in GSH/GSSG ratio was demonstrated. The GSH/GSSG ratio increased over 2 fold on days 3 and 6; then on day 9, it showed a drastic decrease to 41% of day 0 (P < 0.001) (Fig. 5B).
A profound and dose-dependent decrease in total glutathione content was demonstrated in laver extract-treated adipocytes (Fig. 5A). In addition, the transient increase in GSH/GSSG ratio, found in control adipocytes, was not observed; instead, a dose- and time-dependent decrease in GSH/GSSG ratio was shown (Fig. 5B).
Results of our study demonstrated that the aqueous methanol extract of laver, a red algal species, inhibited adipogenesis and induced apoptosis in 3T3-L1 adipocytes.
The laver extract used in this study contained a large quantity of MAAs, i.e., 120 mg/g dried extract, which has been suggested to possess antioxidant activities [10]. In addition to MAAs, the laver extract contained phenolic compounds in the amount of 33 mg GAE/g dried extract, equivalent to 3.63 mg GAE/g dried laver. This is estimated to be similar to or higher than the reported yields from several other red algal species [2829]. However, the yield may vary depending on the extraction methods, solvents, extraction times, and sample conditions. Phenolic compounds present in the diet have generally been recognized to be beneficial to health due to their antioxidant, anti-inflammatory, and vasodilating properties [30]. Laver also contains high quantities of vitamins, minerals, and carotenoids [8].
Adipogenesis is regulated by a complex transcriptional cascade, in which C/EBP family and PPARγ play central roles in coordinating the expression of genes, such as GLUT4, FABP4, FAS, and lipoprotein lipase, involved in insulin sensitivity, lipogenesis, and lipolysis [5]. Our results demonstrated that laver extract inhibited adipogenesis and also reduced the expression of adipogenic proteins in 3T3-L1 cells (Figs. 1 & 2), suggesting that laver extract can interfere with adipogenic transcriptional regulation to inhibit adipogenesis.
The extent of lipid accumulation in the body also correlates with the number of adipocytes in adipose tissue. Our results showed that laver extract exerted differential effects on the viability and apoptosis of preadipocytes and mature adipocytes. Results of the MTT assay of 3T3-L1 preadipocytes indicated that laver extract caused a decrease in preadipocyte viability, up to 50% at 15 mg/mL (Fig. 3A), which can eventually lead to a decrease in the number of adipocytes and thus contribute to the reduction of the mass of adipose tissue. Our results shown in Fig. 3B suggest that this decrease in viability of preadipocytes by laver extract was mainly associated with a decrease in cell proliferation, rather than the increase in apoptosis. It has been reported, particularly from the study of anticancer agents, that some compounds can cause a decrease in cell proliferation without inducing apoptosis by slowing down the cell cycle progression via reduction of transition through G1-phase [3132].
On the contrary, treatment with laver extract caused a significant increase in the apoptosis of differentiated adipocytes (Fig. 4). Therefore, our results suggest that laver extract can reduce the number of adipocytes both by slowing down the proliferation of preadipocytes and by removing adipocytes through apoptosis.
It is possible that the laver extract, at a concentration higher than 10 mg/mL, would also increase the death of cells other than adipocytes. However, considering the observed difference in the efficacies of laver extract on the inhibition of lipid accumulation and the increase in apoptosis, i.e., 76.3% reduction in lipid accumulation vs. 17.0% increase in apoptosis by 15 mg/mL of laver extract, the laver extract could still be useful as an anti-obesity agent by administering the optimum effective dose at which the adverse effects on other normal cells could be minimized.
Our results suggest that laver extract can contribute to the reduction of lipid content in adipose tissue by reducing lipid accumulation in adipocytes, reducing preadipocyte proliferation, and increasing apoptosis of mature adipocytes. These effects of laver extract could probably be attributed to the phenolic compounds present in the extract. Inhibition of adipogenesis and induction of apoptosis by some phenolic compounds, such as resveratrol [33], quercetin [34], and (-) epigallocatechin gallate (EGCG) [3536], has been reported in literature.
Besides hormonal stimuli, the regulatory roles of reactive oxygen species (ROS) in adipogenesis have been suggested in recent studies [37]. However, the effect of oxidative stress caused by ROS on adipogenesis and development of obesity has been controversially reported in the literature [38].
In our results in control 3T3-L1 cells, total glutathione content showed a significant decrease after day 3 (Fig. 5A), indicating that oxidative stress increased during the process of adipogenesis, because the depletion of cellular glutathione is considered a clear marker for oxidative stress [39]. This result shows correlation with those of other studies demonstrating progressively increased oxygen consumption and ROS generation during adipocyte differentiation [4041]. The functional and structural changes of mitochondria have been suggested to play crucial roles in the increased oxidative stress during adipocyte differentiation [41].
Our results also demonstrated that during adipogenesis, GSH/GSSG ratio, an indicator of the redox status of the glutathione pool, showed a remarkable increase on days 3 and 6 and then a drastic decrease on day 9 (Fig. 5B). This increase in GSH/GSSG ratio, which requires the function of glutathione reductase and NADPH to reduce GSSG to GSH, might be closely correlated with the increase in NADPH requirement for the synthesis of fatty acids during adipogenesis. Cellular NADPH is primarily provided by glucose 6-phosphate dehydrogenase (G6PD). It was reported that the G6PD activity increased progressively during adipocyte differentiation, along with the generation of ROS [40]. Nuclear factor erythroid-derived factor 2-related factor 2 (Nrf2) is the master regulator of the cellular adaptive response to oxidative stress, including upregulation of G6PD [42]. Nrf2 has also been shown to play a crucial role in adipogenesis by regulating the expression of adipogenic transcription factors via antioxidant response elements [43]. The drastic decrease in GSH/GSSG on day 9 could be due to the severely increased oxidative stress in late adipogenesis stage, which accelerates oxidation of GSH to GSSG.
In laver extract-treated 3T3-L1 cells, total glutathione pool was severely depleted, and the transient increase in GSH/GSSG ratio, which was evidently shown in control cells, was not observed (Fig. 5). These results suggest that a prooxidant role of laver extract might be important for its antiadipogenic and proapoptotic effects in adipocytes. Association of the inhibition of adipogenesis and subsequent decrease in adipose tissue mass in elderly with age-related increase in oxidative stress in adipose tissue has been reported [44]. The prooxidant effect of laver extract might be attributed to phenolic compounds present in the extract. Although phenolic compounds are generally recognized as potent antioxidant molecules, increasing evidence has shown that phenolic compound-mediated oxidative stress plays important roles in their beneficial health effects, such as chemoprevention [4546]. For example, EGCG has been suggested to induce differential mitochondrial dysfunction and oxidative stress in normal and cancer cells, selectively targeting cancer cells. A decrease in glutathione content in 3T3-L1 adipocytes by EGCG was also reported [47].
In conclusion, our results demonstrated that the aqueous methanol extract of laver inhibited adipogenesis and induced a decrease in proliferation of preadipocytes and an increase in apoptosis of mature adipocytes. Our results suggested that laver extract exerted these effects by increasing oxidative stress, as demonstrated by the decrease in total glutathione content and the oxidation of the cellular glutathione pool, which could be attributable to some phenolic compounds present in the extract.
Figures and Tables
Fig. 1
The effect of laver extract on lipid accumulation in 3T3-L1 adipocytes.
Two-day postconfluent 3T3-L1 preadipocytes (day 0) were induced to differentiate. From day 0 to day 9, the cells were treated with various concentrations of laver extract in the differentiation medium. Accumulated lipids in cells were stained with Oil Red O on day 9 (A: representative images of stained cells). Lipid contents were determined by eluting the dye with isopropanol and measuring the absorbance at 510 nm (B). The lipid content was normalized to the protein content in each well. The results are expressed as the mean ± SD (n = 6). *** P < 0.001 vs. cells without extract treatment.
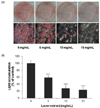
Fig. 2
The effect of laver extract on adipogenic protein levels in 3T3-L1 adipocytes.
On day 9, the 3T3-L1 adipocytes which had been treated with various concentrations of laver extract were harvested and subjected to Western blot analysis using antibodies to PPARγ, C/EBPαs, FABP4, FAS, and β-actin (A). Then the blot was densitometrically analyzed and normalized to β-actin (B). The plots show the representative of three independent experiments.
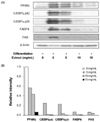
Fig. 3
The effect of laver extract on viability and apoptosis in 3T3-L1 preadipocytes.
3T3-L1 preadipocytes were treated with various concentrations of laver extract for 48 h. Cell viability was determined by MTT assay (A). Cell death was analyzed by flow cytometry with annexin V-FITC and propidium iodide (PI) staining of the cells. The percentages of apoptotic cells, i.e., cells positive for annexin V and negative for PI, and necrotic cells, i.e., cells positive for both annexin V and PI, were determined. The results are expressed as the mean ± SD (n = 16 for MTT; n = 3 for flow cytometry). ** P < 0.01; *** P < 0.001 vs. cells without extract treatment.
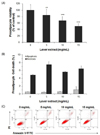
Fig. 4
The effect of laver extract on apoptosis in 3T3-L1 adipocytes.
Differentiated 3T3-L1 adipocytes were treated with various concentrations of laver extract for 48 h, i.e., during days 7-9. Cell death was analyzed by flow cytometry with annexin V-FITC and propidium iodide (PI) staining of the cells. The percentages of apoptotic cells, i.e., cells positive for annexin V and negative for PI, and necrotic cells, i.e., cells positive for both annexin V and PI, were determined. The results are expressed as the mean ± SD (n = 3). * P < 0.05 vs. cells without extract treatment.
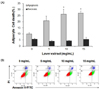
Fig. 5
The effect of laver extract on the content and redox status of glutathione in 3T3-L1 adipocytes.
From day 0 to day 9, the cells were treated with various concentrations of laver extract in the differentiation medium. On days 0, 3, 6, and 9, total glutathione content (A) and the ratio of GSH/GSSG (B) in cells were measured. The results are expressed as the mean ± SD (n = 6). *** P < 0.001 vs. cells without extract treatment on day 0.
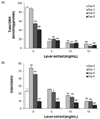
References
1. Saltiel AR, Kahn CR. Insulin signalling and the regulation of glucose and lipid metabolism. Nature. 2001; 414:799–806.


2. Camp HS, Ren D, Leff T. Adipogenesis and fat-cell function in obesity and diabetes. Trends Mol Med. 2002; 8:442–447.


3. Couillard C, Mauriège P, Imbeault P, Prud'homme D, Nadeau A, Tremblay A, Bouchard C, Després JP. Hyperleptinemia is more closely associated with adipose cell hypertrophy than with adipose tissue hyperplasia. Int J Obes Relat Metab Disord. 2000; 24:782–788.


6. Gregoire FM, Smas CM, Sul HS. Understanding adipocyte differentiation. Physiol Rev. 1998; 78:783–809.


7. Rayalam S, Della-Fera MA, Baile CA. Phytochemicals and regulation of the adipocyte life cycle. J Nutr Biochem. 2008; 19:717–726.


8. Lee JC, Hou MF, Huang HW, Chang FR, Yeh CC, Tang JY, Chang HW. Marine algal natural products with anti-oxidative, anti-inflammatory, and anti-cancer properties. Cancer Cell Int. 2013; 13:55.


10. Yuan YV, Walsh NA. Antioxidant and antiproliferative activities of extracts from a variety of edible seaweeds. Food Chem Toxicol. 2006; 44:1144–1150.


11. Gröniger A, Sinha RP, Klisch M, Häder DP. Photoprotective compounds in cyanobacteria, phytoplankton and macroalgae--a database. J Photochem Photobiol B. 2000; 58:115–122.


12. Sinha RP, Singh SP, Häder DP. Database on mycosporines and mycosporine-like amino acids (MAAs) in fungi, cyanobacteria, macroalgae, phytoplankton and animals. J Photochem Photobiol B. 2007; 89:29–35.


13. Esteban R, Martínez B, Fernández-Marín B, María Becerril J, García-Plazaola JI. Carotenoid composition in Rhodophyta: insights into xanthophyll regulation in Corallina elongata. Eur J Phycol. 2009; 44:221–230.


14. Olsen EK, Hansen E, Isaksson J, Andersen JH. Cellular antioxidant effect of four bromophenols from the red algae, Vertebrata lanosa. Mar Drugs. 2013; 11:2769–2784.


15. Wang H, Fu XM, Han CC. The potential applications of marine bioactives against diabetes and obesity. Am J Mar Sci. 2014; 2:1–8.
16. Kim KJ, Lee OH, Lee BY. Fucoidan, a sulfated polysaccharide, inhibits adipogenesis through the mitogen-activated protein kinase pathway in 3T3-L1 preadipocytes. Life Sci. 2010; 86:791–797.


17. Maeda H, Hosokawa M, Sashima T, Takahashi N, Kawada T, Miyashita K. Fucoxanthin and its metabolite, fucoxanthinol, suppress adipocyte differentiation in 3T3-L1 cells. Int J Mol Med. 2006; 18:147–152.


18. Jeon HJ, Seo MJ, Choi HS, Lee OH, Lee BY. Gelidium elegans, an edible red seaweed, and hesperidin inhibit lipid accumulation and production of reactive oxygen species and reactive nitrogen species in 3T3-L1 and RAW264.7 cells. Phytother Res. 2014; 28:1701–1709.


19. Kim HM, Kang SI, Shin HS, Ko HC, Hong YS, Kang SW, Yoon SA, Kim JH, Kim SJ. Anti-obesity effect of Komulkosiraegi [Gracilaria vermiculophylla (Ohmi) Papenfuss] extract in 3T3-L1 cells. Food Sci Biotechnol. 2012; 21:83–89.


20. Gironi F, Piemonte V. Temperature and solvent effects on polyphenol extraction process from chestnut tree wood. Chem Eng Res Des. 2011; 89:857–862.


21. Volkmann M, Gorbushina AA. A broadly applicable method for extraction and characterization of mycosporines and mycosporine-like amino acids of terrestrial, marine and freshwater origin. FEMS Microbiol Lett. 2006; 255:286–295.


22. Klisch M, Häder DP. Wavelength dependence of mycosporine-like amino acid synthesis in Gyrodinium dorsum. J Photochem Photobiol B. 2002; 66:60–66.


23. Singleton VL, Rossi JA. Colorimetry of total phenolics with phosphomolybdic-phosphotungstic acid reagents. Am K Enol Vitic. 1965; 16:144–158.
24. Ramírez-Zacarías JL, Castro-Muñozledo F, Kuri-Harcuch W. Quantitation of adipose conversion and triglycerides by staining intracytoplasmic lipids with Oil red O. Histochemistry. 1992; 97:493–497.


25. Lowry OH, Rosebrough NJ, Farr AL, Randall RJ. Protein measurement with the Folin phenol reagent. J Biol Chem. 1951; 193:265–275.


26. Neuschwander-Tetri BA, Roll FJ. Glutathione measurement by high-performance liquid chromatography separation and fluorometric detection of the glutathione-orthophthalaldehyde adduct. Anal Biochem. 1989; 179:236–241.


27. Kim S, You DH, Han T, Choi EM. Modulation of viability and apoptosis of UVB-exposed human keratinocyte HaCaT cells by aqueous methanol extract of laver (Porphyra yezoensis). J Photochem Photobiol B. 2014; 141:301–307.


28. Zubia M, Fabre MS, Kerjean V, Deslandes E. Antioxidant and cytotoxic activities of some red algae (Rhodophyta) from Brittany coasts (France). Bot Mar. 2009; 52:268–277.


29. Hwang ES, Thi ND. Effects of extraction and processing methods on antioxidant compound contents and radical scavenging activities of laver (Porphyra tenera). Prev Nutr Food Sci. 2014; 19:40–48.


30. Landete JM. Updated knowledge about polyphenols: functions, bioavailability, metabolism, and health. Crit Rev Food Sci Nutr. 2012; 52:936–948.


31. Martin-Fernandez C, Bales J, Hodgkinson C, Welman A, Welham MJ, Dive C, Morrow CJ. Blocking phosphoinositide 3-kinase activity in colorectal cancer cells reduces proliferation but does not increase apoptosis alone or in combination with cytotoxic drugs. Mol Cancer Res. 2009; 7:955–965.


32. Hamtiaux L, Hansoulle L, Dauguet N, Muccioli GG, Gallez B, Lambert DM. Increasing antiproliferative properties of endocannabinoids in N1E-115 neuroblastoma cells through inhibition of their metabolism. PLoS One. 2011; 6:e26823.


33. Rayalam S, Yang JY, Ambati S, Della-Fera MA, Baile CA. Resveratrol induces apoptosis and inhibits adipogenesis in 3T3-L1 adipocytes. Phytother Res. 2008; 22:1367–1371.


34. Yang JY, Della-Fera MA, Rayalam S, Ambati S, Hartzell DL, Park HJ, Baile CA. Enhanced inhibition of adipogenesis and induction of apoptosis in 3T3-L1 adipocytes with combinations of resveratrol and quercetin. Life Sci. 2008; 82:1032–1039.


35. Kim H, Hiraishi A, Tsuchiya K, Sakamoto K. (-) Epigallocatechin gallate suppresses the differentiation of 3T3-L1 preadipocytes through transcription factors FoxO1 and SREBP1c. Cytotechnology. 2010; 62:245–255.


36. Andersen C, Rayalam S, Della-Fera MA, Baile CA. Phytochemicals and adipogenesis. Biofactors. 2010; 36:415–422.


37. Liu GS, Chan EC, Higuchi M, Dusting GJ, Jiang F. Redox mechanisms in regulation of adipocyte differentiation: beyond a general stress response. Cells. 2012; 1:976–993.


38. Gummersbach C, Hemmrich K, Kröncke KD, Suschek CV, Fehsel K, Pallua N. New aspects of adipogenesis: radicals and oxidative stress. Differentiation. 2009; 77:115–120.


39. Mytilineou C, Kramer BC, Yabut JA. Glutathione depletion and oxidative stress. Parkinsonism Relat Disord. 2002; 8:385–387.


40. Torres-Ramrez N, Baiza-Gutman LA, García-Macedo R, Ortega-Camarillo C, Contreras-Ramos A, Medina-Navarro R, Cruz M, Ibáñez-Hernández MÁ, Díaz-Flores M. Nicotinamide, a glucose-6-phosphate dehydrogenase non-competitive mixed inhibitor, modifies redox balance and lipid accumulation in 3T3-L1 cells. Life Sci. 2013; 93:975–985.


41. Ducluzeau PH, Priou M, Weitheimer M, Flamment M, Duluc L, Iacobazi F, Soleti R, Simard G, Durand A, Rieusset J, Andriantsitohaina R, Malthièry Y. Dynamic regulation of mitochondrial network and oxidative functions during 3T3-L1 fat cell differentiation. J Physiol Biochem. 2011; 67:285–296.


42. Maher J, Yamamoto M. The rise of antioxidant signaling--the evolution and hormetic actions of Nrf2. Toxicol Appl Pharmacol. 2010; 244:4–15.


43. Hou Y, Xue P, Bai Y, Liu D, Woods CG, Yarborough K, Fu J, Zhang Q, Sun G, Collins S, Chan JY, Yamamoto M, Andersen ME, Pi J. Nuclear factor erythroid-derived factor 2-related factor 2 regulates transcription of CCAAT/enhancer-binding protein beta during adipogenesis. Free Radic Biol Med. 2012; 52:462–472.


44. Findeisen HM, Pearson KJ, Gizard F, Zhao Y, Qing H, Jones KL, Cohn D, Heywood EB, de Cabo R, Bruemmer D. Oxidative stress accumulates in adipose tissue during aging and inhibits adipogenesis. PLoS One. 2011; 6:e18532.


45. Tao L, Park JY, Lambert JD. Differential prooxidative effects of the green tea polyphenol, (-)-epigallocatechin-3-gallate, in normal and oral cancer cells are related to differences in sirtuin 3 signaling. Mol Nutr Food Res. 2015; 59:203–211.


46. Kucinska M, Piotrowska H, Luczak MW, Mikula-Pietrasik J, Ksiazek K, Wozniak M, Wierzchowski M, Dudka J, Jäger W, Murias M. Effects of hydroxylated resveratrol analogs on oxidative stress and cancer cells death in human acute T cell leukemia cell line: prooxidative potential of hydroxylated resveratrol analogs. Chem Biol Interact. 2014; 209:96–110.

