Abstract
BACKGROUND/OBJECTIVES
Sonchus asper is used extensively as an herbal anti-inflammatory for treatment of bronchitis, asthma, wounds, burns, and cough; however, further investigation is needed in order to understand the underlying mechanism. To determine its mechanism of action, we examined the effects of an ethyl acetate fraction (EAF) of S. asper on nitric oxide (NO) production and prostaglandin-E2 levels in lipopolysaccharide (LPS)-stimulated RAW264.7 macrophages.
MATERIALS/METHODS
An in vitro culture of RAW264.7 macrophages was treated with LPS to induce inflammation.
RESULTS
Treatment with EAF resulted in significant suppression of oxidative stress in RAW264.7 macrophages as demonstrated by increased endogenous superoxide dismutase (SOD) activity and intracellular glutathione levels, decreased generation of reactive oxygen species and lipid peroxidation, and restoration of the mitochondrial membrane potential. To confirm its anti-inflammatory effects, analysis of expression of inducible NO synthase, cyclooxygenase-2, tumor necrosis factor-α, and the anti-inflammatory cytokines IL-1β and IL-6 was performed using semi-quantitative RT-PCR. EAF treatment resulted in significantly reduced dose-dependent expression of all of these factors, and enhanced expression of the antioxidants MnSOD and heme oxygenase-1. In addition, HPLC fingerprint results suggest that rutin, caffeic acid, and quercetin may be the active ingredients in EAF.
Oxidative stress is defined as levels of reactive oxygen species (ROS) that exceed the capacity of antioxidant defenses. Overproduction of ROS and/or decreased antioxidant capacity can result in an imbalanced redox milieu. The antioxidant system includes exogenous and endogenous components; the latter include antioxidant enzymes such as superoxide dismutases (CuZnSOD, MnSOD), glutathione peroxidase (GSPx), and heme oxygenase-1 (HO-1) [1]. The superoxide anion is an important ROS generated by metabolic and enzymatic sources, including mitochondrial respiration [2], NAD(P)H oxidases [3], cyclooxygenases, and lipoxygenases [4]. The superoxide anion reacts with nitric oxide (NO) to produce reactive nitrogen species (RNS), such as peroxynitrite. Superoxide dismutases (SODs) convert superoxide anion to the less harmful peroxide and thus regulate intracellular and extracellular levels of superoxide, peroxide, and RNS [5]. Glutathione (GSH), a key intracellular tripeptide thiol composed of glutamic acid, cysteine, and glycine, is the major endogenous antioxidant that protects cells from ROS such as free radicals and peroxides. Accumulation of free radicals associated with oxidative stress has been implicated in the pathogenesis of several disease states, including vascular diseases [6], diabetes, renal ischemia, atherosclerosis [7], pulmonary pathological states, inflammatory diseases, cancer, and aging [8]. Oxidative-stress-mediated modification of lipids and proteins and decreases in available NO lead to inflammation and dysregulated cell proliferation [9]. In the second stage of chronic inflammation, accumulation of ROS resulting from phagocytic "respiratory burst" induces production of soluble mediators, including cytokines and chemokines. These mediators activate signal transduction cascades and induce expression of cyclooxygenase-2 (COX-2), inducible nitric oxide synthase (iNOS), inflammatory cytokines including tumor necrosis factor (TNF-α), interleukin-1 (IL-1β), and IL-6, and chemokines [10].
Sonchus asper (L.) Hill produces a large number of antioxidants, including carotenoids, catechin, rutin, and quercetin [11], and has been used for treatment of cough, tonsils [12], gastrointestinal infections and inflammation, fungal infections, diabetes, and cardiac dysfunction [13]. Recently the methanol extract of S. asper was shown to prevent CCl4-induced nephrotoxicity in rats [14]. S. asper has been used extensively as an herbal anti-inflammatory for treatment of bronchitis, asthma, wounds, burns, and cough, however, further investigation is needed in order to understand the underlying mechanism. The purpose of this study was to examine the question of whether the anti-inflammatory effect of S. asper was mediated by suppression of oxidative stress in RAW264.7 macrophages.
Sonchus asper (L.) Hill aerial part at flowering was collected in Xinxiang, Henan, China in May, 2012. The harvested plant material was morphologically identified at Henan Institute of Science and Technology by Professor Li Meng. The fresh Sonchus asper (L.) Hill aerial part was air dried at room temperature for 1 week and pulverized by machine into a fine powder; 100 g powder was extracted with 1L methanol at 60℃ 3 times for 24 hours, and dried to yield 4.05 g extract. After further fractionation by n-hexane, dichloromethane, ethyl acetate, and n-butanol in a stepwise manner, the yield of the ethyl acetate fraction (EAF) was 9.3 mg; this was used for the anti-inflammatory assays in this study because we found that the ethyl acetate fraction showed the highest NO inhibition rate (93.36%) at 100 µg/mL; the NO inhibition rates of the other extracts were 24.94%, 66.80%, 46.04%, and 41.10% at 100 µg/mL, respectively (data not shown in manuscript).
RAW264.7 mouse leukaemic monocyte macrophage cell line was obtained from the American Type Cell Culture (Rockville, MD, USA) and cultured in RPMI-1640 medium (Gibco, MD, USA) supplemented with 10% FBS (Gibco), 100 U/mL penicillin, and 100 µg/mL streptomycin sulfate. The cytotoxicity and growth-inhibitory effect of EAF was assessed using the standard 3-(4,5-dimethylthiazol-2-yl)-2,5-diphenyltetra-zolium bromide (MTT) cell viability assay following the methods of Wang et al. [15].
RAW264.7 cells (5 × 105 cells/mL) were pre-incubated for 20 h, followed by incubation with EAF (100, 50, 25, and 12.5 µg/mL) with or without LPS (1 µg/mL) for 24 h; then intracellular ROS were measured using fluorescent dye 2',7'-dichlorodihydrofluorescein diacetate (DCFDA, Molecular Probes, Invitrogen) as described previously [15]. Three independent samples of 10,000 fluorescence events were analyzed for each experimental condition.
RAW264.7 macrophage cells (5 × 105 cells/mL) were incubated with EAF (100, 50, 25, and 12.5 µg/mL) with or without LPS (1 µg/mL) for 24 h; cells were harvested in PBS (pH 7.4), and the cell lysates were used to evaluate the membrane lipid peroxides. Membrane lipid peroxidation was expressed by the level of malondialdehyde (MDA) formation (nM/105 cells) according to the methods of Tiwari et al., 2010 [16].
RAW264.7 cells (5 × 105 cells/mL) were pre-incubated for 20 h, followed by incubation with EAF (100, 50, 25, 12.5 µg/mL) with or without LPS (1 µg/mL) for 24h; cells were then lysed by addition of 200 µL of lysis buffer (10 mM Tris, 20 mM EDTA, 0.25% tritonX-100, pH 8), and the homogenate was centrifuged at 12000 rpm for 10 min at 4℃; the supernatant was used for measurement of SOD activity using the nitroblue tetrazolium-NADH-phenazine methosulfate (NBT-NADH-PMS) system. The 50% inhibition of formazan formation in 1 min was taken as one unit of enzyme activity/minute [17].
After pre-incubation of RAW264.7 cells (5 × 105 cells/mL) for 20 h, EAF (100, 50, 25, and 12.5 µg/mL) with or without LPS (1 µg/mL) was added to the plate, followed by incubation for 24 h; cells were then lysed and GSH levels were quantified using the methods of Tiwari et al., 2010 [16]. The GSH level is expressed as µmoles GSH/1 × 105 cells based on a standard curve of reduced GSH.
Mitochondrial membrane potential (Δψ) was measured by the incorporation of a lipophilic cationic fluorescent dye 3,3'-diehexiloxadicarbocyanine iodide [DiOC6(3)]. Briefly, after incubation of RAW264.7 cells (5 × 105 cells/mL) and EAF (100, 50, 25, and 12.5 µg/mL) with or without LPS (1 µg/mL) for 24 h, cells were washed with PBS two times, followed by incubation with 25 nM of DiOC6 for 30 min at 37℃ in the dark. Fluorescence was measured by FACScan flow cytometry (Becton-Dickinson, San Jose, CA).
Nitrite production was determined using 5 × 105 RAW264.7 cells/mL grown in 96-well plates overnight. The cells were then incubated with EAF (100, 50, 25, and 12.5 µg/mL) and LPS for 24 h, neither LPS nor samples were added to the control. Then cell-free supernatants were collected and NO was determined using the Griess reaction (1% sulfanilamide in 5% phosphoric acid and 0.1% naphthylethylenediamine dihydrochloride in water) as reported previously [18].
Prostaglandin E2 (PGE2) concentrations in cell culture supernatants were determined using the PGE2 assay kit (Enzo life sciences Inc, New York, USA), according to the manufacturer's instructions. After incubation of cells with EAF (100, 50, 25, and 12.5 µg/mL) and LPS, neither LPS nor samples were added to the control, for 24 h. Then cell-free supernatants were collected and PGE2 production was measured. All samples were assayed in duplicate, and equivocal results were repeated.
Total RNA was extracted from cells treated with or without EAF (100, 50, 25, and 12.5 µg/mL) using Trizol reagent (Invitrogen, USA). For cDNA synthesis, 2 µg RNA was reverse transcribed with moloney murine leukemia virus reverse transcriptase (RT). Semi-quantitative PCR was performed using primers specific for TNF-α, COX-2, iNOS, IL-1β, IL-6, and GAPDH, as described by [19]. The primers (Bioneer, Seoul, Korea) for MnSOD and HO-1 were: Forward 5'-GTGACTT-TGGGTCTTTTGAG-3', Reverse, 5'-GCTAACATTCTCCCAGTTGA-3' and Forward 5'-AAGATTGCCCAG AAAGCCCTGGAC-3', Reverse, 5'-AACTGTCGCCACCAG-AAAGCT GAG-3', respectively. The products of RT-PCR were separated by electrophoresis using 1.2% agarose gel and stained with ethidium bromide. Densitometric analysis was performed using image analysis software (Gel pro 4.5 Media Cybernetics, USA).
A waters Alliance HPLC system (Waters, Milford, MA, USA), equipped with a PDA 2996 photo diode array detector (Waters, Milford, MA,USA), and a Micromass Quattro microTM API benchtop triple quadrupole mass spectrometer (Waters MS Technologies, Manchester, UK) were used.The chromatographic conditions used for determination of individual phenolic compounds were according to those reported by Enayat [20] with some modifications. The chromatographic separation was performed on a kinetex C18 (150 × 4.6 mm, i.d. 5 µm) operated at 40℃ with the mobile phase A (H2O containing 0.05% formic acid) and B (acetonitrile). Samples (10 µl) were injected into the column using an autosampler and the flow rate was maintained at 1 ml/min. The gradient program was as follows: 100:0 for 0-7 min, 80:20 for 7-47 min, 60:40 for 47-72 min, and a final conditioning step at a ratio of 100:0 for 72-75 min. The detection wavelength was set at 280 nm for gallic acid, catechin, and epigallocatechin gallate (EGCG), 320 nm for caffeic acid and coumaric acid and 360 nm for rutin and quercetin.
The effect of EAF (100, 50, 25, and 12.5 µg/mL) on viability of un-stimulated RAW264.7 cells was tested using the MTT assay. Treatment with EAF at concentrations from 12.5 to 100 µg/mL had no effect on cell growth, indicating that these doses are not cytotoxic to RAW264.7 cells (Fig. 1).
Intracellular ROS levels after treatment of LPS-treated RAW 264.7 cells with 100, 50, 25, or 12.5 µg/mL EAF are shown in Fig. 2. Treatment with 100, 50, 25, and 12.5 µg/mL EAF resulted in reduced LPS-induced ROS generation by 58%, 54%, 47%, and 7%, respectively. Our results clearly demonstrate that EAF inhibited generation of ROS in a dose-dependent manner in LPS-stimulated RAW264.7 cells.
Because lipid peroxidation is a well-defined mechanism of cellular damage in animals, we measured the effects of EAF on concentrations of malondialdehyde (MDA), an aldehydic secondary product of lipid peroxidation, following LPS treatment. Aldehydic products are a widely accepted indicator of oxidative damage, and MDA causes both structural and functional damage to proteins by formation of stable adducts. While LPS stimulated MDA formation in RAW264.7 cells, pre-treatment with 100, 50, 25, and 12.5 µg/mL EAF counteracted this effect (Table 1), indicating that EAF suppressed peroxidation of membrane lipids.
SOD is one of three key antioxidant enzymes produced by the human body. Together with catalase (CAT) and glutathione peroxidase (GPX), these enzymes constitute the first line of defense against oxidative stress, and many studies have shown that up-regulation of all three occurs simultaneously to restore redox balance. LPS stimulation decreased SOD activity by 31% in RAW264.7 cells, compared with the control, but after treatment with 100, 50, 25, and 12.5 µg/mL EAF for 24 h, SOD activity was restored by 27%, 21%, 18%, and 7%, respectively (Table 1). These results suggest that EAF induces up-regulation of SOD activity in LPS-stimulated RAW264.7 cells.
GSH has been implicated in immune modulation and inflammatory responses. Oxidative stress and inflammation-triggered decreases in cellular GSH levels may lead to transcriptional activation of proinflammatory mediators and antioxidant molecules [21]. LPS treatment resulted in decreased GSH levels, but treatment with EAF resulted in a dose-dependent increase in GSH content in LPS-treated cells (Table 1), suggesting that increases in GSH levels may contribute to the anti-inflammatory effect of EAF.
The effect of various concentrations of EAF on mitochondrial membrane potential in LPS-stimulated cells was measured using the fluorescent probe DiOC6(3). Compared to the control, LPS stimulation resulted in markedly decreased mitochondrial membrane potential (Δψ), but EAF restored these losses in mitochondrial membrane potential in a dose-dependent manner (by 68%, 58%, 45%, and 40% at 100, 50, 25, and 12.5 µg/mL EAF, respectively) (Fig. 3). This result indicates that EAF inhibited the LPS-induced loss of mitochondrial membrane potential.
NO is a key pro-inflammatory factor in many acute and chronic inflammatory diseases; its production is increased by stress-triggered expression of the inducible isoform of NOS (iNOS). iNOS expression is activated by proinflammatory cytokines such as tumor necrosis factor-α, and interferon-γ (IFNγ), and also by oxidative stress [22]. LPS increased NO production by iNOS expression in RAW264.7 cells, and pretreatment with EAF at 100, 50, 25, and 12.5 µg/mL for 24 h inhibited this enhancement by 95%, 84%, 67%, and 55%, respectively (Fig. 4). EAF caused significantly decreased concentration-dependent secretion of NO.
Prostaglandin E2 is reported to play an important role in the inflammatory process [23]. To determine whether EAF can inhibit PGE2 production, LPS-stimulated RAW264.7 cells were treated with 100, 50, 25, and 12.5 µg/mL of EAF for 24 h. EAF also inhibited the production of PGE2 in a concentration-dependent manner (Fig. 5).
The pro-inflammatory enzymes iNOS and COX-2 produce NO and PGE2 in LPS-activated macrophages; thus we examined the effect of EAF on expression of these enzymes. Treatment with EAF at doses of at least 25 µg/mL resulted in almost complete inhibition of iNOS expression in macrophages; this trend is similar to the effect of EAF on NO production. EAF also significantly inhibited expression of COX-2 at all concentrations tested. EAF had similar effects on mRNA levels of the pro-inflammatory cytokines TNF-α and IL-1β and it caused a dose-dependent decrease in the levels of IL-6 mRNA (Fig. 6), while, enhancing expression of HO-1 and MnSOD (Fig. 7), and their expression levels showed positive correlation with GSH levels and SOD activity. These effects on expression of pro-inflammatory enzymes and cytokines, as well as the above-described inhibition of NO production, are characteristic of an anti-inflammatory response, thus EAF might control pro-inflammatory cytokine production at the transcriptional level in association with suppression of oxidative stress. However, the effect of EAF on pro-inflammatory cytokine expression should be examined at the protein level to determine whether these effects are mechanistically linked.
Since the carotenoids catechin, rutin, and quercetin have been reported [11] as the active components in S. asper, the composition of EAF was assessed by HPLC and compared to nine standards (gallic acid, catechin, epigallocatechin gallate (EGCG), caffeic acid, p-coumaric acid, luteolin-7-β-D-glucopyranoside, luteolin, rutin, and quercetin). Comparison of retention times and masses found that EAF includes eight of these compounds, as well as additional phenolic compounds, indicated by the numerous surrounding peaks. Among these standards, rutin was the most abundant at 8.34%, while caffeic acid and quercetin were present at 3.079% and 2.158%, respectively, while the other standards were detected at low levels (Table 2).
S. asper, a wild leafy herb, has been used for centuries as a folk medicine for treatment of inflammatory conditions in South Africa [24], Pakistan [14], Egypt [25], and China, however its pharmacological properties have not been fully evaluated.
The aim of this study was to determine whether EAF has anti-inflammatory effects in LPS-stimulated RAW macrophages and whether these effects are mediated by inhibition of oxidative stress. Therefore, NO release and mRNA levels of cytokines were evaluated. Treatment with EAF at 100 µg/mL resulted in almost complete inhibition of NO production; even EAF at 25 µg/mL reduced NO levels by more than 50%. In RT-PCR analysis, EAF similarly suppressed expression of pro-inflammatory enzymes and cytokines including iNOS, COX-2, IL-1β, IL-6, and TNF-α. EAF drastically inhibited expression of iNOS, IL-1β, and TNF-α at concentrations of 25 µg/mL or greater (Fig. 7) and blocked LPS-induced enhancement of intracellular ROS levels and loss of mitochondrial membrane potential in a dose-dependent manner. High concentrations of ROS are known to activate NF-κB, which induces expression of iNOS, COX-2, IL-1β, IL-6, and TNF-α, linking oxidative stress to overproduction of inflammatory mediators [26]. Thus it appears that EAF can inhibit the expression of the pro-inflammatory cytokines involved in ROS generation.
The anti-inflammatory effects of EAF were confirmed by its enhancement of SOD activity and intracellular GSH level in LPS-activated macrophages. In addition, results of RT-PCR showed that EAF induced up-regulation of the antioxidant genes MnSOD and HO-1 in LPS-activated macrophages. Because the antioxidant enzyme SOD and the major endogenous antioxidant GSH are major contributors to intracellular redox balance, we conclude that EAF is a strong antioxidant that protects against oxidative stress in LPS-activated macrophages.
In addition, by HPLC-MS, rutin, caffeic acid, gallic acid, p-coumaric acid, and epigallocatechin were identified as components of EAF. Although rutin is known to have strong anti-inflammatory properties, caffeic acid is a superior antioxidant that quenches radicals; i.e., singlet oxygen, thus increasing resistance to lipid peroxidation; these known compounds may contribute to the anti-inflammatory effect to some extent. However, as they compose a small proportion of EAF, conduct of further studies will be required for identification of the remaining functional components.
In this study, we examined the capacity of EAF to block NO and PGE2 production. EAF-induced expression of pro-inflammatory cytokines was associated with a reduction of oxidative stress in LPS-activated macrophages. However, the molecular mechanism linking these effects, which likely involves activation of NF-κB, should be investigated further.
Figures and Tables
Fig. 1
Effect of different concentrations of EAF of S. asper on RAW264.7 macrophage viability was measured by MTT reduction assay.
Data are expressed as the mean ± SD of three independent experiments.
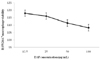
Fig. 2
Flow cytometric analysis of intracellular accumulation of ROS after EAF treatment.
The number meaning is the ROS fluorescence events after different treatment. Data are expressed as the mean ± SD of three independent experiments. (A) control (without LPS), (B) LPS, (C) 100 µg/mL with LPS, (D) 50 µg/mL with LPS (E) 25 µg/mLwith LPS, (F) 12.5 µg/mL with LPS.
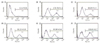
Fig. 3
Effect of EAF of S. asper on the loss of mitochondrial membrane potential of LPS-stimulated macrophages.
Data are expressed as the mean ± SD of three independent experiments. Lowercase letters indicate significant differences according to ANOVA test (a < b < c < d) (P < 0.05).
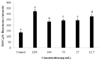
Fig. 4
Effect of EAF of S. asper on LPS-induced NO production in RAW264.7 macrophages.
Data are expressed as the mean ± SD of three independent experiments. Lowercase letters indicate significant differences according to ANOVA test (a < b < c < d) (P < 0.05).
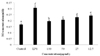
Fig. 5
Effect of EAF of S. asper on LPS-induced PGE2 production in RAW264.7 macrophages.
Data are expressed as the mean ± SD of three independent experiments. Lowercase letters indicate significant differences according to ANOVA test (a < b < c < d) (P < 0.05).
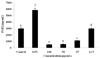
Fig. 6
Effects of EAF of S. asper on pro-inflammtory gene expression analyzed by semi-quantitative RT-PCR.
(A) EtBr staining of PCR products using primers specific for iNOS, COX-2, IL-1β, IL-6, TNF-α, and GAPDH, respectively. (B) Quantification of PCR products (A) relative to GAPDH following treatment with EAF. Data are mean ± SD of three independent experiments. Lowercase letters indicate significant differences according to ANOVA test (a < b < c < d) (P < 0.05).
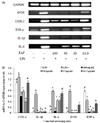
Fig. 7
Effects of EAF of S. asper on antioxidant gene expression analyzed by semi-quantitative RT-PCR.
(A) EtBr staining of PCR products using primers specific for MnSOD, HO-1, and GAPDH, respectively. (B) Quantification of PCR products (A) relative to GAPDH following treatment with EAF. Data are expressed as mean ± SD of three independent experiments. Lowercase letters indicate significant differences according to ANOVA test (a < b < c < d) (P < 0.05).
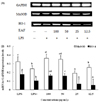
References
1. Leopold JA, Loscalzo J. Oxidative mechanisms and atherothrombotic cardiovascular disease. Drug Discov Today Ther Strateg. 2008; 5:5–13.


2. Turrens JF, Boveris A. Generation of superoxide anion by the NADH dehydrogenase of bovine heart mitochondria. Biochem J. 1980; 191:421–427.


3. Griendling KK, Sorescu D, Ushio-Fukai M. NAD(P)H oxidase: role in cardiovascular biology and disease. Circ Res. 2000; 86:494–501.
4. Romano M, Claria J. Cyclooxygenase-2 and 5-lipoxygenase converging functions on cell proliferation and tumor angiogenesis: implications for cancer therapy. FASEB J. 2003; 17:1986–1995.


5. Kinnula VL, Crapo JD. Superoxide dismutases in the lung and human lung diseases. Am J Respir Crit Care Med. 2003; 167:1600–1619.


6. Cai H, Harrison DG. Endothelial dysfunction in cardiovascular diseases: the role of oxidant stress. Circ Res. 2000; 87:840–844.


7. Lakshmi SV, Padmaja G, Kuppusamy P, Kutala VK. Oxidative stress in cardiovascular disease. Indian J Biochem Biophys. 2009; 46:421–440.
8. Benz CC, Yau C. Ageing, oxidative stress and cancer: paradigms in parallax. Nat Rev Cancer. 2008; 8:875–879.


9. Yung LM, Leung FP, Yao X, Chen ZY, Huang Y. Reactive oxygen species in vascular wall. Cardiovasc Hematol Disord Drug Targets. 2006; 6:1–19.


10. Reuter S, Gupta SC, Chaturvedi MM, Aggarwal BB. Oxidative stress, inflammation, and cancer: how are they linked? Free Radic Biol Med. 2010; 49:1603–1616.


11. Balasundram N, Sundram K, Samman S. Phenolic compounds in plants and agro industrial by-products: antioxidant activity, occurrence, and potential uses. Food Chem. 2006; 99:191–203.


12. Jeruto P, Lukhoba C, Ouma G, Otieno D, Mutai C. An ethnobotanical study of medicinal plants used by the Nandi people in Kenya. J Ethnopharmacol. 2008; 116:370–376.


13. Sabeen M, Ahmad SS. Exploring the folk medicinal flora of Abbotabad City, Pakistan. Ethnobot Leafl. 2009; 13:810–833.
14. Khan RA, Khan MR, Sahreen S, Bokhari J. Prevention of CCl4-induced nephrotoxicity with Sonchus asper in rat. Food Chem Toxicol. 2010; 48:2469–2476.
15. Wang L, Xu ML, Hu JH, Rasmussen SK, Wang MH. Codonopsis lanceolata extract induces G0/G1 arrest and apoptosis in human colon tumor HT-29 cells--involvement of ROS generation and polyamine depletion. Food Chem Toxicol. 2011; 49:149–154.


16. Tiwari M, Dwivedi UN, Kakkar P. Suppression of oxidative stress and pro-inflammatory mediators by Cymbopogon citratus D. Stapf extract in lipopolysaccharide stimulated murine alveolar macrophages. Food Chem Toxicol. 2010; 48:2913–2919.


17. Kakkar P, Das B, Viswanathan PN. A modified spectrophotometric assay of superoxide dismutase. Indian J Biochem Biophys. 1984; 21:130–132.
18. Cho JY, Baik KU, Jung JH, Park MH. In vitro anti-inflammatory effects of cynaropicrin, a sesquiterpene lactone, from Saussurea lappa. Eur J Pharmacol. 2000; 398:399–407.


19. Lee HJ, Hyun EA, Yoon WJ, Kim BH, Rhee MH, Kang HK, Cho JY, Yoo ES. In vitro anti-inflammatory and anti-oxidative effects of Cinnamomum camphora extracts. J Ethnopharmacol. 2006; 103:208–216.


20. Enayat S, Banerjee S. Comparative antioxidant activity of extracts from leaves, bark and catkins of Salix aegyptiaca sp. Food Chem. 2009; 116:23–28.


21. Rahman I, MacNee W. Regulation of redox glutathione levels and gene transcription in lung inflammation: therapeutic approaches. Free Radic Biol Med. 2000; 28:1405–1420.


22. Kleinert H, Euchenhofer C, Ihrig-Biedert I, Förstermann U. Glucocorticoids inhibit the induction of nitric oxide synthase II by down-regulating cytokine-induced activity of transcription factor nuclear factor-kappa B. Mol Pharmacol. 1996; 49:15–21.
23. Hennebert O, Pelissier MA, Le Mee S, Wülfert E, Morfin R. Anti-inflammatory effects and changes in prostaglandin patterns induced by 7beta-hydroxy-epiandrosterone in rats with colitis. J Steroid Biochem Mol Biol. 2008; 110:255–262.


24. Afolayan AJ, Jimoh FO. Nutritional quality of some wild leafy vegetables in South Africa. Int J Food Sci Nutr. 2009; 60:424–431.

