Abstract
BACKGROUND/OBJECTIVES
Mulberry leaves contain quercetin derivatives, which have the effects of reducing obesity and improving lipid and glucose metabolism in mice with obesity. It is not clear whether or not mulberry leaves can directly affect metabolic disorders, in the presence of obesity, because of the interaction between obesity and metabolic disorders. The aim of the current study was to assess the direct action of quercetin derivatives on metabolic disorders in non-obese conditions in short-term high-fat diet fed mice.
MATERIALS/METHODS
C57BL/6N mice were fed a high-fat diet, supplemented with either 0% (control), 1%, or 3% mulberry leaf powder (Mul) or 1% catechin powder for five days. Anthropometric parameters and blood biochemistry were determined, and hepatic gene expression associated with lipid and glucose metabolism was analyzed.
RESULTS
Body and white fat weights did not differ among the four groups. Plasma triglycerides, total cholesterol, and free fatty acids in the 1%, 3% Mul and catechin groups did not differ significantly from those of the controls, however, plasma glucose and 8-isoprostane levels were significantly reduced. Liver gene expression of gp91phox, a main component of NADPH oxidase, was significantly down-regulated, and PPAR-α, related to β-oxidation, was significantly up-regulated. FAS and GPAT, involved in lipid metabolism, were significantly down-regulated, and Ehhadh was significantly up-regulated. Glucose-metabolism related genes, L-PK and G6Pase, were significantly down-regulated, while GK was significantly up-regulated in the two Mul groups compared to the control group.
CONCLUSIONS
Our results suggest that the Mul quercetin derivatives can directly improve lipid and glucose metabolism by reducing oxidative stress and enhancing β-oxidation. The 1% Mul and 1% catechin groups had similar levels of polyphenol compound intake (0.4 × 10-5 vs 0.4 × 10-5 mole/5 days) and exhibited similar effects, but neither showed dose-dependent effects on lipid and glucose metabolism or oxidative stress.
Polyphenol compounds that are ubiquitous in edible plants (fruits, vegetables, and herbs) and plant-derived beverages have recently received increased attention due to their biologic activities. Obesity, hyperglycemia, and hypercholesterolemia are major risk factors for chronic disease in humans, including type-2 diabetes, cardiovascular disease, hypertension, and stroke [1], and have been reduced in animal by polyphenol compounds [2].
In a previous study, we reported on the antiatherogenic activities of a major polyphenol compound (quercetin 3-(6)-malonylglucoside; Q3MG) found in mulberry leaves, manifested through increased LDL resistance to oxidative modification [3]. Mulberry leaves also exhibited anti-obesity effects, reducing hepatic oxidative stress and enhancing lipid metabolism [4,5]. Obesity is related to oxidative stress, through attenuation of antioxidant enzymes levels and increased production of reactive oxygen species (ROS) [6,7]. Our previous study assessed only the relationship between quercetin derivatives and obesity; we did not at that time examine the direct effects of quercetin derivatives on metabolic disorders.
Previous studies have reported that quercetin derivatives inhibit ROS production and improve lipid metabolism by enhancing β-oxidation with obesity; however, the effects of quercetin derivatives were not clearly elucidated before development of obesity, and neither were study group differences arising as a result of the varying amounts of mulberry leaf dietary supplement. Therefore, in the current study we assessed the short-term effects of mulberry quercetin derivatives on metabolic disorders without obesity.
The dried mulberry leaf powder (Mul) contained 763 mg/100 g dry weight (1.40 × 10-3 mole/100 g diet) of Q3MG, 96 mg/100 g dry weight (0.21 × 10-3 mole/100 g diet) of quercetin 3-glucoside (Q3G), and 226 mg/100 g dry weight (0.37 × 10-3 mole/100 g diet) of rutin (quercetin 3-rutinoside).
Catechin, a typical polyphenol compound, present in green tea, consumed world-wide as a healthy drink, is associated with a low risk of developing cardiovascular and metabolic diseases [8,9]. Green-tea catechin includes epigallocatechin gallate (EGCG), epicatechin (EC), epigallocatechin (EGC), and epicatechin gallate (ECG). Catechin powder used here was made from polyphenon (Mitsui Norin Co., Ltd.) and contained 432 mg/100 g dry weight (0.94 × 10-3 mol/100g diet) of EGCG, 88 mg/100 g dry weight (0.20 × 10-3 mole/100 g diet) of ECG, 176 mg/100 g dry weight (0.38 × 10-3 mole/100 g diet) of EGC, and 80 mg/100 g dry weight (0.28 × 10-3 mole/100 g diet) of EC.
Mice diet was a high-fat (HF) diet (15 g beef tallow, 3 g cholesterol and 82 g CE-2/100 g diet), supplemented with 0% (control group), 1% (w/w, 1% Mul group) or 3% (w/w, 3% Mul group) dried mulberry leaf power. The Q3MG (molecular weight: MW 546.2), Q3G (MW 464.4) and rutin (MW 610.5) in the 1% Mul diet were 7.63 mg/100 g diet (1.40 × 10-5mole/100 g diet), 0.96 mg/100 g diet (0.21 × 10-5mole/100 g diet) and 2.26 mg/100 g diet (0.37 × 10-5mole/100 g diet), respectively; 3% Mul diet was 22.89 mg/100 g diet (4.19 × 10-5mole/100 g diet), 2.88 mg/100 g diet (0.62 × 10-5mole/100 g diet) and 6.78 mg/100 g (1.11 × 10-5mole/100 g diet), respectively. The catechin diet (catechin group) was a high-fat diet, supplemented with 1% catechin powder; in the catechin diet. EGCG (MW 458.4) was 4.32 mg/100 g (0.94 × 10-5 mole/100 g diet), ECG (MW 442.4) was 0.88 mg/100 g (0.20 × 10-5 mole/100 g diet), EGC (MW 458.4) was 1.76 mg/100 g (0.38 × 10-5 mole/100 g diet), and EC (MW 290.3) was 0.80 mg/100 g (0.28 × 10-5 mole/100 g diet). In the current study, 1% catechin was used as a positive control. The 1% Mul diet and catechin diet had almost same molecules of polyphenol compounds (1.98 vs 1.83 × 10-5 mole/100 g diet). Compositions of the experimental diets are shown in Table 1, including estimated total quercetin and catechin derivatives.
All male C57BL/6N mice, aged nine weeks, were purchased from Japan Charles River Laboratories (Yokohama, Japan). The mice were housed in the Department of Experimental Animals, Interdisciplinary Center for Science Research, Organization for Research, Shimane University, under controlled ambient conditions: relative humidity 55 ± 10%, temperature 23 ± 2℃ a 12-h light/dark cycle (07:00-19:00 and 19:00-07:00, respectively), air change 13-15 times/h and consumed food and water ad libitum. The mice were randomly divided into four groups: control group, 1% Mul group, 3% Mul group, and catechin group, with seven mice in each group. The four mice groups were fed their respective experimental diets for five days. After feeding, the mice were fasted overnight and anesthetized on day 6. Blood was collected from the heart. Epididymal adipose tissue, liver, soleus muscle, and liver were harvested and weighed; liver was stored at -80℃ until analysis. This study and all procedures were approved by the Animal Care and Use Committee of Shimane University, Japan (IZ-25-20).
Blood was placed in tubes containing EDTA2Na, and the plasma was separated immediately by centrifugation at 3,000 g for 10 min at 4℃. Plasma was stored at -80℃ until analysis. Concentration of plasma glucose, triglycerides (TG), high-density lipoprotein-cholesterol (HDL-C), total cholesterol (TC), free fatty acids (FFA), aspartate aminotransferase (AST), alanine aminotransferase (ALT), and γ-glutamyltranspeptidase (γ-GTP) were measured using enzymatic assay kits (Wako Pure Chemical, Osaka, Japan); concentrations of plasma insulin were measured using a Mouse Insulin ELISA KIT (Shibayagi, Gunma, Japan), and concentrations of 8-isoprostane were measured using an 8-Isoprostane EIA KIT (Cayman Chemical, Company, Michigan, USA), all according to the manufacturers' protocols.
Measurement of gene expression of enzymes related to hepatic glucose and lipid metabolism, β-oxidation and oxidative stress was performed by quantitative RT-PCR analysis. Total RNA was extracted from the liver using a High Pure RNA Isolation Kit (Roche, Mannheim, Germany) according to the supplier's protocol; the quality and quantity were checked by agarose gel electrophoresis and spectrophotometer. Reverse transcription was performed using a ReverTra Ace-α-cDNA Synthesis kit (Toyobo, Osaka, Japan). Quantitative RT-PCR was performed using the Applied Biosystems 7900HT system, utilizing Power SYBR Green PCR Master Mix (Life Technologies, California, USA) according to the supplier's protocol manual, with 18S rRNA used for internal control; to clearly extend oxidative stress, gp91phox, a key subunit of NADPH oxidase and involved in ROS generation, was selected. To clearly extend lipid metabolism Ehhadh, FAS and GPAT were selected, and for glucose metabolism, G6Pase, GK, and L-PK were chosen. The following primers were used in the current study: 18S rRNA (forward) aagatcgatcgccacatgtatca, (reverse) tgcgtgcttddttggtcttaga; GP91 phox (forward) ttgggtcagcactggctctg, (reverse) tggcggtgtgcagt gctatc; PPARα (forward) tcagggtaccactacggagttca, (reverse) ccgaatagttcgccgaaaga; Ehhadh (forward) gttggcttcccggaagtgat; (reverse) ctccaacgaccctgggtaga;ChREBP1 (forward) ctggggacctaaa caggagc; (reverse) gaagccaccctatagctccc;CPT1A (forward) ggatct acaattcccctctgc; (reverse) gcaaaataggtctgccgaca; SCD1 (forward) ddggagacccttagatcga; (reverse) agcctgtaaaagatttctgcaaacc; ACC (forward) agcagatccgcagcttg; (reverse)acctctgctcgctgagtgc; Aco x (forward) agcgagccagagccccag, (reverse) tcaggcagctcactcagg; FAS (forward) ttccaagacgaaaatgatgc; (reverse) aattgtgggatcagga gagc; GPAT (forward) caacaccatccccgacatc; (reverse)gtgaccttcga ttatgcgatca; GK (forward) ccctgagtggcttacagttc; (reverse) acggat gtgagtgttgaagc; G6Pase (forward) tcttgtggttgggatactgg; (reverse) gcaatgcctgacaagactc; L-PK (forward) gtcccacactttggaagcat; (reverse) cccaacactgattctaccc.
Data were analyzed using SPSS software version 12.0 J (SPSS Inc., Tokyo, Japan). The results are expressed as mean ± SD. Comparisons of the four diet groups were performed by one-way analysis of variance (ANOVA), and post hoc analyses were used with Tukey's test for multiple comparisons. P-values of less than 0.05 for ANOVA and 0.017 for post hoc analyses were used to assess significant differences.
Food intake and anthropometric parameters of the four groups are summarized in Table 2. Food and energy intake did not differ significantly among the four groups. Body weights of the four groups were nearly identical at the beginning and end of the experiment covering a five-day period. Organ (liver, epididymis adipose tissue, and soleus muscle) weights also did not differ significantly among the four groups.
The effects of Mul and catechin on plasma parameters are summarized in Table 2. Fasting plasma glucose levels were significantly lower in the two Mul and catechin groups compared to the control group, but did not differ significantly among themselves. Plasma triglyceride, total cholesterol, HDL-cholesterol, free fatty acids, insulin, AST, ALT, and γ-GTP did not differ significantly among the four groups. Significantly lower plasma 8-isoprostane was observed in the two Mul and catechin groups compared with the control group.
The effects of Mul and catechin on hepatic gene expression are shown in Fig. 1. The gene expression of gp91phox, a key subunit of NADPH oxidase with involvement in ROS generation was significantly down-regulated in the two Mul and catechin groups, compared to the control group. Expression of PPAR-α, with and important role in β-oxidation, was significantly up-regulated in the Mul groups compared to the control group. Ehhadh was significantly up-regulated in the two Mul groups, while FAS and GPAT were significantly down-regulated in the two Mul and catechin groups, compared to the control group. G6Pase and L-PK gene expression were significantly down-regulated and GK gene expression was significantly up-regulated in the two Mul groups compared to the control group. Expression of Acox, CPT1A, SCD1, ACC, and ChREBP1 did not differ significantly among the four groups, and no significant differences in gene expression were observed among the two Mul and catechin groups. Comparison of gene expression in the 1% and 3% Mul groups showed no significant difference for oxidative stress (gp91 phox), β-oxidation (PPARα), lipid metabolism (Ehhadh, FAS, and GPAT) or glucose metabolism (GK, G6Pase, and L-PK).
In this study, there were no significant differences in food intake, organ weight, plasma triglycerides, total cholesterol, or free fatty acids. However, compared to the control group, blood glucose levels were significantly different in the 1%, 3% Mul and catechin groups, but no significant difference was observed for gene expression in the liver.
In the current study, we investigated the enhancement of lipid and glucose metabolism occurring as a direct result of the effects of quercetin derivatives from mulberry leaves in mice by short-feeding without obesity influences. Our results clearly show that diet supplementation with quercetin-rich Mul derivatives directly improves lipid and glucose metabolism, attenuating oxidative stress and enhancing β-oxidation.
Phenolic antioxidants from plants may act as regulators of lipid metabolism in mice or rats fed high-fat diets [10,11]. In our previous studies, the effect of quercetin derivatives on improvement of lipid metabolism was accompanied by a decrease in expression of hepatic fatty acid syntheses and enhanced β-oxidation. Quercetin derivatives also exhibited antioxidant activities, which might stimulate hepatic lipid metabolism, reduce plasma lipid levels, and suppress fat accumulation in the liver and adipose tissue. Quercetin derivatives can be beneficial in suppression of high-fat diet-induced obesity by modulation of hepatic lipid metabolism [5,8,9]. However, in studies based on long-term-feeding experiments with obesity, the effects of quercetin derivatives were unclear in short-term feeding without obesity. Obesity is an important cause of increased oxidative stress, and is a metabolic disturbance resulting from an imbalance between fat synthesis (lipogenesis) and lipolysis (oxidation) [11,12]. In previous studies, obesity was induced in mice by long-term feeding of HF diet. Accordingly, to better assess and evaluate benefits of quercetin derivatives, we designed a short-feeding experiment by feeding an HF diet that minimized the influence of obesity.
In the current study, initial and final body weights, body weight gains and liver weight were nearly identical, and plasma lipid (TG total, cholesterol, HDL-cholesterol, and free acids) levels did not differ among the four groups (two Mul, catechin and control groups), with no differences from the effects of obesity. We surmised that the quercetin and catechin derivatives would have direct effects on lipid and glucose metabolism, compared to the control group, and we sought to compare any such effects. Our results clearly showed that supplementation with Mul and catechin derivatives led to improved lipid and glucose metabolism in the liver compared with the non-supplemented control, and that the effects of the derivatives were independent of the anti-obesity effects. We also found that quercetin derivatives directly improved hepatic lipid metabolism by stimulating β-oxidation, enhancing PPAR-α, and suppressing ROS production in short-term feeding without obesity.
The liver plays a vital role in lipid metabolism and regulation of β-oxidation, which in turn may contribute to increased lipid levels in plasma and the liver [9,13]. PPAR-α is highly expressed in the liver and plays a crucial role in the induction of hepatic fatty acid oxidation and regulation of lipid metabolism [14,15]. Polyphenol compounds have been reported to improve lipid metabolism by enhancing PPAR-α and other mRNA expression related β-oxidation in the liver [3,16]. Therefore, we investigated the effects of quercetin derivatives from mulberry leaves on the gene expression of PPAR-α related to β-oxidation in the liver for the purpose of improving lipid metabolism.
Ehhadh, ChREBP1, FAS, and GPAT, included in lipid-metabolism, are transcriptionally regulated by PPAR-α [17,18]. We found that the gene expression of PPAR-α and Ehhadh was significantly up-regulated, while FAS and GPAT were significantly down-regulated in the two Mul groups. We hypothesized that Mul quercetin derivatives would promote fatty acid oxidation and increase energy expenditure by enhancing the expression of these enzymes related to β-oxidation and would regulate fatty acid synthesis by reducing the enzymes associated with lipogenesis and enhancing lipolysis in the short term without development obesity. However, no significant differences in plasma lipids were observed among the four groups. In this short-term study, quercetin derivatives improved the effects of lipid metabolism only in the liver.
Glucose-metabolism plays an important part in hepatic metabolism, and polyphenol compounds have been reported to improve glucose metabolism [19]. A similar phenomenon from Mul quercetin derivatives was noted in our previous studies [3,4,5]. The current study also showed that the plasma glucose levels of the two Mul groups were significantly lower than those of the control group, with an enhanced GK and decreased L-PK and G6Pase gene expression in the liver. Therefore, we believe that quercetin derivatives from mulberry leaves can reduce plasma glucose levels by their influence on hepatic gene expression.
Oxidative stress is a result of excess ROS production and/or deficient antioxidant capacity in diet-induced obesity [6]. NADPH oxidase has a major role in ROS generation and increased gp91phox induces ROS overproduction [20,21]. In the current study, gp91phox expression was significantly down-regulated in the two Mul groups and the catechin group, indicating that oxidative stress may be attenuated. We believe that Mul quercetin derivatives can directly protect or attenuate oxidative stress and contribute to improved lipid metabolism without obesity influences in short-term feeding.
Several reports have proposed a mechanism of the anti-obesity effects which improve lipid and glucose metabolism: EGCG inhibits ROS production by suppression of NADPH oxidase expression, enhances β-oxidation by stimulating expression of PPARα and increases energy expenditure [22,23,24,25]. The action of Mul quercetin derivatives and catechin derivatives might involve a similar mechanism by changing the gene expression profile in the liver: protecting against ROS generation by reducing gp91phox expression and improving lipid metabolism by enhancing PPAR-α expression.
For the 1% Mul and catechin groups, which had virtually identical molecular mass intake levels of polyphenol compounds (0.4 × 10-5mole/5 days), the magnitude of 1% Mul supplementation was similar to that of the catechin supplementation in hepatic gene expression related to oxidative stress and β-oxidation. Our findings here show a parallel trend for the Mul quercetin and the catechin derivatives in regulation of gene expression related to hepatic lipid and glucose metabolism attenuating the increase of blood lipid and glucose levels. No significant differences in hepatic gene expression were observed between the 1% and 3% Mul groups. In addition, our results indicate that the effects of 1% and 3% Mul were not dose-dependent, as 1% Mul provided the maximum effective molecular mass of polyphenol compounds to improve the profile of hepatic gene expression related lipid and glucose metabolism.
The current study has some limitations. The concentrations of 8-isoprotane were significantly different in the two Mul and catechin groups compared to the control group. Although we realized that the effects of quercetin derivatives improved the suppression of ROS generation and enhanced lipid metabolism, the interaction between ROS generation and lipid metabolism was not fully elucidated in this experiment.
In conclusion, the current study shows that quercetin derivatives from mulberry leaves directly prevent hepatic metabolism in high-fat-fed mice, and these effects may be related to decreased oxidative stress and lipid metabolism, enhanced β-oxidation and improved glucose metabolism in the liver of short time feeding without development of obesity.
Figures and Tables
Fig. 1
Gene expression changes in the liver induced by mulberry and catechin powder.
Each values expressed means ± SD. 7 mice in each group (control (0% Mul), 1% Mulberry leaves (1% Mul), 3% Mulberry leaves (3% Mul), Catechin). The statistical significance of differences among values was analyzed by one-way ANOVA (P < 0.05). Asterisk means Tukey HSD significant difference with post-hoc test compared to control group (P < 0.017).
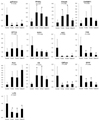
References
1. Knekt P, Kumpulainen J, Järvinen R, Rissanen H, Heliövaara M, Reunanen A, Hakulinen T, Aromaa A. Flavonoid intake and risk of chronic diseases. Am J Clin Nutr. 2002; 76:560–568.


2. Bose M, Lambert JD, Ju J, Reuhl KR, Shapses SA, Yang CS. The major green tea polyphenol, (-)-epigallocatechin-3-gallate, inhibits obesity, metabolic syndrome, and fatty liver disease in high-fat-fed mice. J Nutr. 2008; 138:1677–1683.


3. Katsube T, Imawaka N, Kawano Y, Yamazaki Y, Shiwaku K, Yamane Y. Antioxidant flavonol glycosides in mulberry (Morus alba L.) leaves isolated based on LDL antioxidant activity. Food Chem. 2006; 97:25–31.


4. Wang L, Yamasaki M, Katsube T, Sun X, Yamasaki Y, Shiwaku K. Antiobesity effect of polyphenolic compounds from molokheiya (Corchorus olitorius L.) leaves in LDL receptor-deficient mice. Eur J Nutr. 2011; 50:127–133.


5. Enkhmaa B, Shiwaku K, Katsube T, Kitajima K, Anuurad E, Yamasaki M, Yamane Y. Mulberry (Morus alba L.) leaves and their major flavonol quercetin 3-(6-malonylglucoside) attenuate atherosclerotic lesion development in LDL receptor-deficient mice. J Nutr. 2005; 135:729–734.


6. Furukawa S, Fujita T, Shimabukuro M, Iwaki M, Yamada Y, Nakajima Y, Nakayama O, Makishima M, Matsuda M, Shimomura I. Increased oxidative stress in obesity and its impact on metabolic syndrome. J Clin Invest. 2004; 114:1752–1761.


8. Thielecke F, Boschmann M. The potential role of green tea catechins in the prevention of the metabolic syndrome - a review. Phytochemistry. 2009; 70:11–24.


9. Murase T, Nagasawa A, Suzuki J, Hase T, Tokimitsu I. Beneficial effects of tea catechins on diet-induced obesity: stimulation of lipid catabolism in the liver. Int J Obes Relat Metab Disord. 2002; 26:1459–1464.


10. Feillet-Coudray C, Sutra T, Fouret G, Ramos J, Wrutniak-Cabello C, Cabello G, Cristol JP, Coudray C. Oxidative stress in rats fed a high-fat high-sucrose diet and preventive effect of polyphenols: Involvement of mitochondrial and NAD(P)H oxidase systems. Free Radic Biol Med. 2009; 46:624–632.
11. Kuda T, Iwai A, Yano T. Effect of red pepper Capsicum annuum var. conoides and garlic Allium sativum on plasma lipid levels and cecal microflora in mice fed beef tallow. Food Chem Toxicol. 2004; 42:1695–1700.


12. Han LK, Sumiyoshi M, Zhang J, Liu MX, Zhang XF, Zheng YN, Okuda H, Kimura Y. Anti-obesity action of Salix matsudana leaves (Part 1). Anti-obesity action by polyphenols of Salix matsudana in high fat-diet treated rodent animals. Phytother Res. 2003; 17:1188–1194.


13. Aoki F, Honda S, Kishida H, Kitano M, Arai N, Tanaka H, Yokota S, Nakagawa K, Asakura T, Nakai Y, Mae T. Suppression by licorice flavonoids of abdominal fat accumulation and body weight gain in high-fat diet-induced obese C57BL/6J mice. Biosci Biotechnol Biochem. 2007; 71:206–214.


14. Nemali MR, Usuda N, Reddy MK, Oyasu K, Hashimoto T, Osumi T, Rao MS, Reddy JK. Comparison of constitutive and inducible levels of expression of peroxisomal beta-oxidation and catalase genes in liver and extrahepatic tissues of rat. Cancer Res. 1988; 48:5316–5324.
15. Hashimoto T, Fujita T, Usuda N, Cook W, Qi C, Peters JM, Gonzalez FJ, Yeldandi AV, Rao MS, Reddy JK. Peroxisomal and mitochondrial fatty acid beta-oxidation in mice nullizygous for both peroxisome proliferator-activated receptor alpha and peroxisomal fatty acyl-CoA oxidase. Genotype correlation with fatty liver phenotype. J Biol Chem. 1999; 274:19228–19236.


16. Shimoda H, Tanaka J, Kikuchi M, Fukuda T, Ito H, Hatano T, Yoshida T. Effect of polyphenol-rich extract from walnut on diet-induced hypertriglyceridemia in mice via enhancement of fatty acid oxidation in the liver. J Agric Food Chem. 2009; 57:1786–1792.


17. Ide T, Ashakumary L, Takahashi Y, Kushiro M, Fukuda N, Sugano M. Sesamin, a sesame lignan, decreases fatty acid synthesis in rat liver accompanying the down-regulation of sterol regulatory element binding protein-1. Biochim Biophys Acta. 2001; 1534:1–13.


18. Kajikawa S, Harada T, Kawashima A, Imada K, Mizuguchi K. Highly purified eicosapentaenoic acid prevents the progression of hepatic steatosis by repressing monounsaturated fatty acid synthesis in high-fat/high-sucrose diet-fed mice. Prostaglandins Leukot Essent Fatty Acids. 2009; 80:229–238.


19. Honda S, Aoki F, Tanaka H, Kishida H, Nishiyama T, Okada S, Matsumoto I, Abe K, Mae T. Effects of ingested turmeric oleoresin on glucose and lipid metabolisms in obese diabetic mice: a DNA microarray study. J Agric Food Chem. 2006; 54:9055–9062.


20. Yamazaki T, Kishimoto K, Miura S, Ezaki O. Dietary beta-conglycinin prevents fatty liver induced by a high-fat diet by a decrease in peroxisome proliferator-activated receptor gamma2 protein. J Nutr Biochem. 2012; 23:123–132.


21. Roberts CK, Barnard RJ, Sindhu RK, Jurczak M, Ehdaie A, Vaziri ND. Oxidative stress and dysregulation of NAD(P)H oxidase and antioxidant enzymes in diet-induced metabolic syndrome. Metabolism. 2006; 55:928–934.


22. Tachibana H, Koga K, Fujimura Y, Yamada K. A receptor for green teapolyphenol EGCG. Nat Struct Mol Biol. 2004; 11:380–381.
23. Rains TM, Agarwal S, Maki KC. Antiobesity effects of green tea catechins: a mechanistic review. J Nutr Biochem. 2011; 22:1–7.

