Abstract
BACKGROUND/OBJECTIVES
A variety of immunomodulators can improve the efficacy of low-dose chemotherapeutics. Active hexose correlated compound (AHCC), a mushroom mycelia extract, has been shown to be a strong immunomodulator. Whether AHCC could enhance the antitumor effect of low-dose 5-fluorouracil (5-FU) via regulation of host immunity is unknown.
MATERIALS/METHODS
In the current study Hepatoma 22 (H22) tumor-bearing mice were treated with PBS, 5-FU (10 mg·kg-1·d-1, i.p), or AHCC (360 mg·kg-1·d-1, i.g) plus 5-FU, respectively, for 5 d. CD3+, CD4+, CD8+, and NK in peripheral blood were detected by flow cytometry. ALT, AST, BUN, and Cr levels were measured by biochemical assay. IL-2 and TNFα in serum were measured using the RIA kit and apoptosis of tumor was detected by TUNEL staining. Bax, Bcl-2, and TS protein levels were measured by immunohistochemical staining and mRNA level was evaluated by RT-PCR.
RESULTS
Diet consumption and body weight showed that AHCC had no apparent toxicity. AHCC could reverse liver injury and myelosuppression induced by 5-FU (P < 0.05). Compared to mice treated with 5-FU, mice treated with AHCC plus 5-FU had higher thymus index, percentages of CD3+, CD4+, and NK cells (P < 0.01), and ratio of CD4+/CD8+ (P < 0.01) in peripheral blood. Radioimmunoassay showed that mice treated with AHCC plus 5-FU had the highest serum levels of IL-2 and TNFα compared with the vehicle group and 5-FU group. More importantly, the combination of AHCC and 5-FU produced a more potent antitumor effect (P < 0.05) and caused more severe apoptosis in tumor tissue (P < 0.05) compared with the 5-FU group. In addition, the combination of AHCC and 5-FU further up-regulated the expression of Bcl-2 associated X protein (Bax) (P < 0.01), while it down-regulated the expression of B cell lymphoma 2 (Bcl-2) (P < 0.01).
AHCC is a cultured mycelium extract obtained from Lentinus edodes of Basidiomycete mushrooms fermented in rice bran [1]. AHCC was developed by Professor Toshihiko Okamoto, Department of Pharmacology, Tokyo University. Chemical analysis showed that AHCC is composed mainly of low molecular weight oligosaccharides (~5000 MW), of which 20%-30% are α-1,4 hexose linked. The mycelia of basidiomycetes (mushroom root threads) are cultured for a period of 45-60 days and then subjected to enzymatic reaction, sterilization, concentration, and freeze drying sequentially. AHCC is available as a freeze-dried powder, and has been widely used in Japan as a dietary supplement for over 20 years and is currently used in China and the U.S.
The immunological effects of AHCC are well documented. For example, murine thymic apoptosis induced by dexamethasone could be prevented by pretreatment with AHCC [2]. Administration of AHCC resulted in increased cell proliferation and cytokine production in spleen and increased nitric oxide and cytokine production in peritoneal cells of mice in a hindlimb-unloading model of spaceflight conditions [3]. In addition, AHCC could stimulate proliferation of NK cells and killer T-cells, and production of IL-12 and TNFα [4,5]
In accordance with its immunomodulatory function, the beneficial effects of AHCC supplementation on survival and the immune response to a variety of infectious agents have been demonstrated in animals [6,7,8]. In humans, AHCC has been reported to improve the prognosis of patients with postoperative hepatocellular carcinoma [9]. The potential of AHCC to act as a biological response modifier has been reported by Cowawintaweewat et al. [10].
5-FU is one of the oldest and still most used chemotherapeutics for malignancies arising from breast, gastrointestinal tract, head and neck regions of the body [11]. In recent decades a variety of dosages of 5-FU has been used in clinical trials in treatment of several gastrointestinal tumors and its toxicity (mainly neutropenia, diarrhea, mucositis, hand-foot syndrome, and myelosuppression) increases with higher systemic exposure to 5-FU [12,13,14]. A previous study showed that low-dose 5-FU treatment could reduce immune suppression in patients with recurrent or metastatic colorectal cancer, as indicated by increased CD4/CD8 and Th1/2 ratios and decreased CD4+CD25+ T cells [15]. In addition, low-dose leucovorin plus 5-FU chemotherapy was safe and effective for elderly patients with metastatic colorectal cancer [16].
Immunotherapy is regarded as the fourth cancer therapy after the three major cancer therapies, i.e., surgery, chemotherapy, and radiotherapy. Immunotherapy may improve the efficacy of chemotherapeutics so that chemotherapeutics can achieve the same efficiency at a lower dose and with fewer side effects [17]. In the current study we evaluated the question of whether AHCC could enhance the antitumor effect of low-dose 5-FU via regulation of host immunity.
AHCC capsules were acquired from Amino Up Chemical Co. Ltd (Sapporo, Hokkaido, Japan). Capsules were dissolved in PBS at a concentration of 100 g/L as a stock solution and stored at -20℃. 5-fluorouracil (25 g/L) was purchased from Shanghai Amino Acids Company (Shanghai, China) and diluted to 10 g/L with saline solution. PerCP-conjugated hamster anti-mouse CD3e (Armenian Hamster IgG1 κ isotype control), FITC-conjugated rat anti-mouse CD4 (Rat (LEW) IgG2b κ isotype control), PE-conjugated rat anti-mouse CD8a (Rat (LOU) IgG2a, κ and PE-conjugated rat anti-mouse CD49b (Rat (LEW) IgM κ isotype control) were purchased from BD Biosciences (San Jose, CA). Tumor TACS In situ Apoptosis kit was purchased from Roche Bioscience (Palo Alto, CA) and the RIA kit was purchased from the Institute of Radioimmunity, General Hospital of the People's Liberation Army of China (Beijing, China). Reverse Transcription System and GoTaq PCR Core System I were from Promega (Madison, WI). Mouse monoclonal anti-Bax and anti-bcl-2 were purchased from MaixinBio (Fuzhou, China), rabbit polyclonal anti-thymidylate synthase (TS) was purchased from LifeSpan Biotechnology, Inc. (Nagoya, Japan).
Male Institute of Cancer Research (ICR) mice with body weight from 18 g to 22 g were purchased from the School of Basic Medical Science, Peking University, China. The animals were maintained in a pathogen-free facility (23℃ ± 2℃, 55%± 5% humidity). Food and water were provided ad libitum. All procedures on treating mice were performed according to Animal Care Guidelines issued by the Ministry of Science and Technology of the People's Republic of China and the Animal Care Committee of Fujian University of Traditional Chinese Medicine approved our protocols (2014012). The murine H22 cell line was acquired from the School of Basic Medical Science, Peking University. Cells were cultured in DMEM (Gibco Laboratories, Grand Island, NY) supplemented with 10% FBS (Gibco Laboratories), penicillin and streptomycin (10 U/L, Gibco Laboratories) in a humidified atmosphere with 5% CO2 at 37℃.
The hepatoma model was established by subcutaneous inoculation of mouse H22 cells (1×106 cells per mouse) into the right flank of mice. Forty-eight hours after inoculation, mice were randomly divided into three groups (n = 8): control (PBS, ig), 5-FU (10 mg·kg-1·d-1, ip), and 5-FU+AHCC (5-FU 10 mg·kg-1·d-1, ip + AHCC 360 mg·kg-1·d-1, ig). On day 5 the peripheral blood was collected from the orbital plexus and tumors were excised. Tumor volume was calculated according to the following formula: Tumor Volume (TV mm3) = d2×D/2, where d and D were the shortest and longest diameter, respectively.
Each cage of mice was provided with food daily, and the remaining food was collected on the following day and weighed in order to calculate daily food intake. Body weight was collected at baseline and after 5 d of treatment.
A capillary pipette containing anticoagulant (EDTA for cell counting, heparin for flow cytometry) was inserted into the lateral canthus and blood was collected from the retroorbital sinus. Other blood samples were collected into a procoagulant tube and separated serum was frozen in a new tube. WBC in whole blood (20 µL) was measured using an Automatic Blood Cell Counter (Abbott Laboratories, Abbott Park, Illinois). Alanine aminotransferase (ALT), aspartate aminotransferase (AST), blood urea nitrogen (BUN), and creatinine (Cr) levels were measured using an Auto Biochemical Analyzer (Beckman Coulter, Inc. Brea, CA) with Sigma Diagnostic Kits (St. Louis, MO).
The thymus was resected from the mice, washed with PBS, weighed on d 5, and the thymus index (TI) was calculated according to the following formula, TI = (thymus weight mg/body weight g) ×100.
Blood (100 µL) was added to a heparin coated tube and incubated with fluorochrome-conjugated antibodies (2.5 µL) in the dark for 10 minutes. The antibodies used varied slightly with individual cases, but most often included a combination of CD3/CD4, CD3/CD8, and CD3/CD49. Erythrocytes were lysed by 2 mL of red blood cell lysis buffer (0.155 mol/L ammonium chloride, 0.01 mol/L potassium bicarbonate, 0.1 mmol/L EDTA, and 1% paraformaldehyde in PBS) in the dark for 10 min. After washing with PBS three times, the samples were resuspended with 500 µL PBS and analyzed on a FACScalibur™ flow cytometer with CELLQuest™ software (BD Bioscience, San Jose, CA).
Serum (100 µL) was measured for IL-2 and TNFα using the RIA kit according to the manufacturer's instructions in a Gamma radioimmunoassay counter (Kaipu Electromechanical Co., Ltd., Xi'an, China). The levels of two cytokines were determined by the competitive binding of the cytokines in the sample using 125I radio-labeled IL-2 and TNFα standards, respectively. The sensitivity of the assay was 0.3 ug/L.
Sections of tumors from the mice treated as above were fixed with 4% paraformaldehyde for 48 h. The 5-µm-thick sections of tumor samples were analyzed by terminal deoxynucleotidyl transferase (TdT)-mediated dUTP nick end labeling (TUNEL) staining using a TumorTACS In situ Apoptosis kit (Roche Bioscience, Palo Alto, CA) for detection of fragmented DNA according to the manufacturer's instructions. Microscopic immunohistochemical photos were captured by an Olympus microscope (Olympus, Japan) and a moticam 5000 C camera from Motic Instruments (Richmond, BC Canada) and analyzed using Motic Med 6.0 software. Positive cells and total cells were counted at five arbitrarily selected microscopic fields at 100× magnification (each 7 050 µm2 in size). A dark brown nucleus represented the positive apoptotic cells of the tumor by TUNEL staining and the apoptosis index (AI) was calculated according to the following formula: AI = number of positive cells/total number of cells.
Tumor samples fixed in 10% buffered formalin for 24 h were processed conventionally for paraffin-embedded tumor sections. Sections were subjected to antigen retrieval and blocking of endogenous peroxidase activity. For immunostaining, sections were incubated with the primary antibodies mouse monoclonal anti-Bax (1:100; Maixin Bio, China), mouse monoclonal anti-Bcl-2 (1:150; Maixin Bio, China), and rabbit polyclonal anti-TS (1:150; LifeSpan Biotechnology, Inc.). Sections were then incubated with an appropriate biotinylated secondary antibody followed by conjugated horseradish peroxidase-streptavidin (Maixin Bio, China). Then 3,3'-diaminobenzidine (DAB; Sigma) was added, followed by incubation at room temperature and counterstaining with diluted Harris hematoxylin (Sigma). Cells were quantified by counting Bax-positive cells, Bcl-2-positive cells, TS-positive cells, and total number of cells at five arbitrarily selected fields from each tumor at 100 magnification. Data were presented as percentage of positive cells.
Total RNA was isolated using Trizol reagent from Invitrogen (Carlsbad, CA); 1 µg total RNA was reverse-transcribed into cDNA using the Reverse Transcription System of Promega (Madison, WI) under the following conditions: one cycle at 42℃ for 15 min, one cycle at 95℃ for 5 min, then incubation at 0℃-5℃ for 5 min. Products of cDNA were determined by PCR amplification using the GoTaq PCR Core System I of Promega (Madison, WI) in a total volume of 20 µL. The RT-PCR efficiency was controlled by using a 36B4 fragment as a housekeeping gene. PCR amplification for Bcl-2, Bax, TS, dihydropyrimidine dehydrogenase (DPD), and 36B4 was performed as follows: one cycle of denaturation at 95℃ for 5 min, 35 cycles of denaturation at 95℃ for 30 s, annealing at 57℃, 55℃, 53℃, or 55℃ for 30 s, elongation at 72℃ for 45 s, and one cycle of extension at 72℃ for 10 min. Primers of each gene are shown in Supplemental Table 1. Samples were electrophoresed in 1.5% agarose gel containing goldview in Tris-acetate/EDTA buffer and PCR product images were analyzed using the Chemidoc system and Quantity one software (Bio-Rad, Hercules, CA). All cDNA samples were synthesized in parallel, and PCR reactions were run in triplicate. mRNA levels of each gene were normalized to 36B4 mRNA levels.
Statistical analysis was performed using SPSS 16.0. Data were shown as mean ± SD. A two-sided alpha level (type I error rate) of less than 0.05 was considered to indicate statistical significance. One way analysis of variance (ANOVA) was used for analysis of the data and Fisher's Protected Least Significant Difference (PLSD) was used as a post hoc test.
Compared with the control group, both tumor weight and tumor volume were reduced by 30% (Fig. 1, P < 0.05) in the 5-FU group. Importantly, tumor weight was reduced by 50% in the 5-FU+AHCC group (P < 0.01 vs control, P < 0.05 vs 5-FU).
Significantly lower WBC and LY numbers were observed in the 5-FU group at 5 d (Table 1, P < 0.01). AHCC supplementation could almost reverse the effects of 5-FU on WBC numbers (P < 0.05).
5-FU induced deterioration of liver functions in tumor bearing mice (Table 2, ALT, P < 0.05, AST, P < 0.01). Decreased levels of ALT and AST were observed after administration of AHCC (P < 0.05). Similar levels of BUN and Cr were observed among the three groups.
TI was similar between the control group and the 5-FU group, and it was significantly higher in the 5-FU+AHCC group than in the control group and the 5-FU group (Fig. 3, P < 0.01).
The lowest percentages of CD3+ and CD4+ cells were observed in the control group. Administration of 5-FU alone could increase the percentages of CD3+, CD4+, and CD8+ cells (Table 3, P < 0.05). After treatment of tumor bearing mice with AHCC, the percentages of CD3+ and CD4+ subpopulations were remarkably increased (P < 0.01). The ratio of CD4+/CD8+ was also higher in the 5-FU+AHCC group than in the 5-FU group (P < 0.01). In addition, the highest percentages of NK cells were observed in mice in the 5-FU+AHCC group (P < 0.01).
Qualitative microscopic assessment showed that the percentages of Bax-positive cells in tumors from the 5-FU and 5-FU+AHCC groups were two fold (P < 0.01) and four fold (P < 0.01) higher than that of the control group, respectively (Fig. 6A). Although the expression of Bcl-2 mRNA was not affected by low-dose 5-FU alone, it was significantly down-regulated in the 5-FU+AHCC group (40% of the control and 5-FU groups, P < 0.01). In addition, the expression of Bax was greater in the 5-FU than in the control group, which was further increased in the 5-FU+AHCC group (P < 0.01) (Fig. 6B). RT-PCR showed similar expression of both TS and DPD mRNA among the three groups, indicating that AHCC has no effect on either the anabolism or metabolism of 5-FU (data not shown).
Curative treatments such as surgery, local destruction techniques, and liver transplantation for hepatocellular cancer (HCC) can only be achieved in certain patients [18]. Chemotherapy will be required for most patients. Although chemotherapy has been shown to improve the survival of patients with HCC, serious side-effects and drug resistance have been obstacles to more successful therapeutic outcome for oncologists [19,20,21]. The challenge of seeking new therapeutic approaches that can alleviate the adverse effects of chemotherapy while also improving their efficiency is clear and urgent.
Immunotherapy with chemotherapeutics can achieve the same efficiency at a lower dose and with fewer side effects. Recently, the combination therapy of intraarterial 5-FU with immune stimulants (IFN-α, CpG-containing oligodeoxynucleotide, IFN-γ, et al.) showed an outstanding response rate for intractable HCC [22,23]. Preclinical and clinical studies have shown that the mechanisms of combination therapy may include direct antitumor effects (through cell-cycle arrest and induction of apoptosis) and indirect actions (through immunocompetent cells and antiangiogenic effect) [24,25,26]. Monden et al. [27] showed that 5-FU arterial infusion combined with IFN-α exerted antitumor effects in hepatocellular carcinoma patients.
AHCC, a strong immunity modulator, has been used in combination with phenethyl isothiocyanate, Lupron, Camptosar, 5-luorouracil, and cisplatin with improved prognosis and liver function [28]. In the current study we showed that combination of AHCC and low dose 5-FU was more effective in inhibiting the growth of H22 hepatocellular cancer in a murine model.
Administration of low dose 5-FU for five days did not affect the body weight, food intake, and kidney function of tumor bearing mice, albeit liver function and hematopoiesis were slightly affected. Addition of AHCC to 5-FU resulted in alleviation of liver dysfunction and myelosuppression. These beneficial effects of AHCC on reducing adverse events caused by 5-FU are consistent with previous reports by others [29,30,31]. These results also indicated that AHCC is safe when administered at an effective dose [32]. Indeed, in a 90-day study with Sprague-Dawley rats, the no-observed-adverse-effect level of AHCC was considered to be as high as 3000 mg/kg body weight/day [33].
In addition, we also demonstrated that low-dose 5-FU could improve the immune function of tumor-bearing mice, which is in accordance with the study in cancer patients. Interestingly, AHCC plus low-dose 5-FU was more effective in regulating the immune system than 5-FU alone, as indicated by the higher percentages of T cells and NK cell populations, higher serum IL-2 and TNFα levels and TI in the AHCC plus low-dose 5-FU group. T cells and NK cells are the main cytotoxic lymphocytes responsible for anti-tumor immune responses [34]. IL-2, a T cell growth factor, promotes the proliferation, activation of T cells and B cells [35,36]. IL-2 can also stimulate NK cells and inflammatory cells, including monocytes/macrophages and neutrophils [37,38,39] and enhance the response of cytotoxic T lymphocytes against tumor cells [40]. TNFα is produced by many cell types, including activated macrophages, activated CD4 and CD8 T cells, NK cells, and other cells [41,42]. Previous reports have suggested that AHCC can increase the production of TNFα and IL-12. Our results showed that AHCC plus low-dose 5-FU could significantly increase CD4 T cells and NK cells, which may account for the increased production of IL-2 and TNFα. In healthy adults, AHCC intake reportedly increased the frequency of CD4 and CD8 T cells producing interferon-γ alone, TNFα alone, or both increased and the effects may persist for at least 30 days after discontinuing AHCC [43]. Interestingly, Daddaoua reported that AHCC administration attenuated inflammation via downregulating the expression of proinflammatory cytokines and chemokines (IL-1β, IL-1 receptor antagonist, TNF, and monocyte chemoattractant protein-1) [44]. These observations suggest that AHCC may have bidirectional effects on immune function.
The combination of AHCC and low-dose 5-FU was more potent than low-dose 5-FU alone in inhibiting tumor growth and inducing tumor apoptosis. The effect was not achieved by increasing either TS activity, a major target of 5-FU, or DPD activity, a rate-limiting enzyme in the degradation of 5-FU. Further we detected the AI and apoptosis related factors (Bcl-2 and Bax) of tumor. Our results showed that AHCC enhanced the apoptosis of low dose 5-FU at least due to regulating the expression of Bax and Bcl-2. Hunter et al. reported that AHCC alone and in combination with pegylated liposomal doxorubicin not only increased expression of Bcl-2 and induced apoptosis but also decreased the side effects of pegylated liposomal doxorubicin.. Increased apoptosis may be due in part to enhanced function of T cell and NK cell number by AHCC with low dose 5-FU. Activated T cells and NK cells can interact with death receptors (CD95, tumor necrosis factor (TNF)-related apoptosis-inducing ligand receptor) on the surface of tumor cells and induce tumor cell apoptosis by forming a death-inducing signaling complex to trigger the cascade of caspase activation [45,46].
There are several limitations of these studies. Only 5-FU was used in treatment of the animals, while cisplatin, doxorubicin, vincristine, and 5-FU in different combinations are usually used in the clinical setting. Second, although we found that AHCC has no effect on expression of TS and DPD, in the degradation of 5-FU, we did not directly evaluate the pharmacokinetics of 5-FU. Third, although we found that the combination of AHCC and 5-FU further increased the expression of the proapoptotic proteins Bax and downregulated the expression of antiapoptotic protein Bcl-2, the underlying mechanism was not explored. Finally, clinical studies are required in order to fully define the potential of AHCC as an effective component in a new chemotherapy regimen containing lower dose of chemotherapeutics, which will be of great importance for specific patient populations, including seniors and those with compromised immune function.
Figures and Tables
Fig. 1
Enhanced inhibition of H22 tumors by active hexose correlated compound (AHCC) and low-dose 5-fluorouracil (5-FU) in H22 tumor bearing mice at d 5.
The anti-tumor effect was evaluated by measuring tumor weight (A) and volume (B). Values are mean ± SD, (n = 8). *,**Significantly different from the control group (P < 0.05, P < 0.01, respectively), #Significantly different from the 5-FU group (P < 0.05)
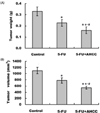
Fig. 2
Effects of AHCC and low-dose 5-FU on body weight and food intake of H22 tumor bearing mice.
The body weight (A) and the average daily food intake (B) were calculated at baseline and after 5 days' treatment. Values are mean ± SD, (n = 8). **Significantly different from the control group (P < 0.01), ##Significantly different from the 5-FU group (P < 0.01)
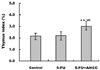
Fig. 3
Effects of AHCC and low-dose 5-FU on TI of H22 tumor bearing ICR mice at d 5.
Values are mean ± SD, (n = 8). **Significantly different from the control group (P < 0.01), ##Significantly different from the 5-FU group (P < 0.01)
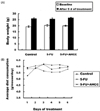
Fig. 4
Effects of AHCC and low-dose 5-FU on cytokine production of H22 tumor bearing ICR mice at d 5.
The serum levels of IL-2 (A) and TNFα (B) were determined by RIA. Values are mean ± SD, (n = 8). *,**Significantly different from the control group (P < 0.05, P < 0.01, respectively), ##Significantly different from the 5-FU group (P < 0.01)
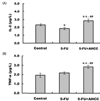
Fig. 5
Effects of AHCC and low-dose 5-FU on apoptosis of tumor cells.
AI is shown as percent of TUNEL-positive cells (A). Values are mean ± SD, (n = 8). *,**Significantly different from the control group (P < 0.05, P < 0.01, respectively), #Significantly different from the 5-FU group (P < 0.05) Representative photos of immunohistochemical staining of TUNEL for the control group (B), the 5-FU group (C), and the 5-FU+AHCC group (D) are shown. Positive cells are marked by arrows. The magnification of microscope was 100.
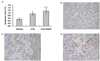
Fig. 6
Effects of AHCC and low-dose 5-FU on the expression of Bax protein (A) and Bax, Bcl-2 gene (B).
Values are mean ± SD, (n = 8). **Significantly different from the control group (P < 0.01), ##Significantly different from the 5-FU group (P < 0.01). mRNA levels of each gene were normalized to 36B4 mRNA levels and are expressed as relative to the control and a value of 1 was assigned to the control group.
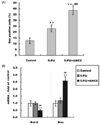
ACKNOWLEDGEMENT
We thank Dr. Robert M. Hackman (Department of Nutrition, University of California, Davis), Dr. Judith A. Smith (The University of Texas M.D. Anderson Cancer Center), and Dr. Lixing Lao (The Center for Integrative Medicine, University of Maryland School of Medicine, Baltimore, USA) for their critical review of the manuscript. We thank Dr. Jun Peng for his insightful suggestions concerning the study protocol.
Notes
This study was supported by CHEN Ke-ji Integrative Medicine Development Fund (CKJ2010020); International Science Joint Project of the Ministry of Science and Technology of the People's Republic of China (2008DFA32200); National Natural Science Foundation of China (81302954); Key Project of Fujian Province Department of Science & Technology (2008KJB-01); Fujian Province Natural Science Foundation(2012J01393); National Natural Science Foundation of China (81302954).
References
1. Kidd PM. The use of mushroom glucans and proteoglycans in cancer treatment. Altern Med Rev. 2000; 5:4–27.
2. Burikhanov RB, Wakame K, Igarashi Y, Wang S, Matsuzaki S. Suppressive effect of active hexose correlated compound (AHCC) on thymic apoptosis induced by dexamethasone in the rat. Endocr Regul. 2000; 34:181–188.
3. Aviles H, Belay T, Vance M, Sun B, Sonnenfeld G. Active hexose correlated compound enhances the immune function of mice in the hindlimb-unloading model of spaceflight conditions. J Appl Physiol (1985). 2004; 97:1437–1444.


4. Uno K, Kosuna K, Sun B, Fujii H, Wakame K, Chikumaru S, Hosokawa G, Ueda Y. Active hexose correlated compound (AHCC) improves immunological parameters and performance status of patients with solid tumors. Biotherapy (Tokyo). 2000; 14:303–309.
5. Aviles H, O'Donnell P, Orshal J, Fujii H, Sun B, Sonnenfeld G. Active hexose correlated compound activates immune function to decrease bacterial load in a murine model of intramuscular infection. Am J Surg. 2008; 195:537–545.


6. Nogusa S, Gerbino J, Ritz BW. Low-dose supplementation with active hexose correlated compound improves the immune response to acute influenza infection in C57BL/6 mice. Nutr Res. 2009; 29:139–143.


7. Ritz BW, Nogusa S, Ackerman EA, Gardner EM. Supplementation with active hexose correlated compound increases the innate immune response of young mice to primary influenza infection. J Nutr. 2006; 136:2868–2873.


8. Wang S, Welte T, Fang H, Chang GJ, Born WK, O'Brien RL, Sun B, Fujii H, Kosuna K, Wang T. Oral administration of active hexose correlated compound enhances host resistance to West Nile encephalitis in mice. J Nutr. 2009; 139:598–602.


9. Matsui Y, Uhara J, Satoi S, Kaibori M, Yamada H, Kitade H, Imamura A, Takai S, Kawaguchi Y, Kwon AH, Kamiyama Y. Improved prognosis of postoperative hepatocellular carcinoma patients when treated with functional foods: a prospective cohort study. J Hepatol. 2002; 37:78–86.


10. Cowawintaweewat S, Manoromana S, Sriplung H, Khuhaprema T, Tongtawe P, Tapchaisri P, Chaicumpa W. Prognostic improvement of patients with advanced liver cancer after active hexose correlated compound (AHCC) treatment. Asian Pac J Allergy Immunol. 2006; 24:33–45.
11. Casale F, Canaparo R, Serpe L, Muntoni E, Pepa CD, Costa M, Mairone L, Zara GP, Fornari G, Eandi M. Plasma concentrations of 5-fluorouracil and its metabolites in colon cancer patients. Pharmacol Res. 2004; 50:173–179.
12. Baglan KL, Frazier RC, Yan D, Huang RR, Martinez AA, Robertson JM. The dose-volume relationship of acute small bowel toxicity from concurrent 5-FU-based chemotherapy and radiation therapy for rectal cancer. Int J Radiat Oncol Biol Phys. 2002; 52:176–183.


13. Dencausse Y, Sturm J, Hartung G, Dietzler P, Edler L, Bambach M, Wojatschek C, Lindemann H, Qeisser W. Adjuvant radio-chemotherapy in stage II-III rectal cancer with 24-hour infusion of high-dose 5-fluorouracil and folinic acid: evaluation of feasibility. Onkologie. 2001; 24:476–480.


14. Grem JL, McAtee N, Steinberg SM, Hamilton JM, Murphy RF, Drake J, Chisena T, Balis F, Cysyk R, Arbuck SG, Sorensen JM, Chen AP, Goldstein L, Jordan E, Setser A, Goldspiel B, DeCarvalho M, Allegra CJ. A phase I study of continuous infusion 5-fluorouracil plus calcium leucovorin in combination with N-(phosphonacetyl)-L-aspartate in metastatic gastrointestinal adenocarcinoma. Cancer Res. 1993; 53:4828–4836.
15. Kobayashi R, Yoshimatsu K, Yokomizo H, Katsube T, Ogawa K. Low-dose chemotherapy with leucovorin plus 5-fluorouracil for colorectal cancer can maintain host immunity. Anticancer Res. 2007; 27:675–679.
16. Yoshimatsu K, Yokomizo H, Fujimoto T, Umehara A, Otani T, Matsumoto A, Osawa G, Shiozawa S, Katsube T, Naritaka Y, Ogawa K. First-line chemotherapy with low-dose leucovorin plus 5-fluorouracil (LV/5-FU) for elderly patients with metastatic colorectal cancer. Anticancer Res. 2007; 27:1641–1644.
17. Butterfield LH, Ribas A. Immunotherapy of hepatocellular carcinoma. Expert Opin Biol Ther. 2002; 2:123–133.


18. Thomas MB, Jaffe D, Choti MM, Belghiti J, Curley S, Fong Y, Gores G, Kerlan R, Merle P, O'Neil B, Poon R, Schwartz L, Tepper J, Yao F, Haller D, Mooney M, Venook A. Hepatocellular carcinoma: consensus recommendations of the National Cancer Institute Clinical Trials Planning Meeting. J Clin Oncol. 2010; 28:3994–4005.


19. Burroughs A, Hochhauser D, Meyer T. Systemic treatment and liver transplantation for hepatocellular carcinoma: two ends of the therapeutic spectrum. Lancet Oncol. 2004; 5:409–418.


20. Ganne-Carrié N, Trinchet JC. Systemic treatment of hepatocellular carcinoma. Eur J Gastroenterol Hepatol. 2004; 16:275–281.
21. Llovet JM, Bruix J. Systematic review of randomized trials for unresectable hepatocellular carcinoma: Chemoembolization improves survival. Hepatology. 2003; 37:429–442.


22. Kasai K, Ushio A, Kasai Y, Sawara K, Miyamoto Y, Oikawa K, Kuroda H, Takikawa Y, Suzuki K. Therapeutic efficacy of combination therapy with intra-arterial 5-fluorouracil and systemic pegylated interferon α-2b for advanced hepatocellular carcinoma with portal venous invasion. Cancer. 2012; 118:3302–3310.


23. Nagano H, Wada H, Kobayashi S, Marubashi S, Eguchi H, Tanemura M, Tomimaru Y, Osuga K, Umeshita K, Doki Y, Mori M. Long-term outcome of combined interferon-α and 5-fluorouracil treatment for advanced hepatocellular carcinoma with major portal vein thrombosis. Oncology. 2011; 80:63–69.


24. Noda T, Nagano H, Takemasa I, Yoshioka S, Murakami M, Wada H, Kobayashi S, Marubashi S, Takeda Y, Dono K, Umeshita K, Matsuura N, Matsubara K, Doki Y, Mori M, Monden M. Activation of Wnt/beta-catenin signalling pathway induces chemoresistance to interferon-alpha/5-fluorouracil combination therapy for hepatocellular carcinoma. Br J Cancer. 2009; 100:1647–1658.


25. Wada H, Nagano H, Yamamoto H, Noda T, Murakami M, Kobayashi S, Marubashi S, Eguchi H, Takeda Y, Tanemura M, Umeshita K, Doki Y, Mori M. Combination of interferon-alpha and 5-fluorouracil inhibits endothelial cell growth directly and by regulation of angiogenic factors released by tumor cells. BMC Cancer. 2009; 9:361.


26. Yin H, Xie F, Zhang J, Yang Y, Deng B, Sun J, Wang Q, Qu X, Mao H. Combination of interferon-α and 5-fluorouracil induces apoptosis through mitochondrial pathway in hepatocellular carcinoma in vitro. Cancer Lett. 2011; 306:34–42.


27. Monden M, Sakon M, Sakata Y, Ueda Y, Hashimura E. FAIT Research Group. 5-fluorouracil arterial infusion + interferon therapy for highly advanced hepatocellular carcinoma: A multicenter, randomized, phase II study. Hepatol Res. 2012; 42:150–165.


28. Ghoneum M, Wimbley M, Salem F, McKlain A, Attallah N, Gill G. Immu-nomodulatory and anticancer effects of active hexose correlated compound (AHCC). Int J Immunother. 1995; 11:23–28.
29. Hirose A, Sato E, Fujii H, Sun B, Nishioka H, Aruoma OI. The influence of active hexose correlated compound (AHCC) on cisplatin-evoked chemotherapeutic and side effects in tumor-bearing mice. Toxicol Appl Pharmacol. 2007; 222:152–158.


30. Shigama K, Nakaya A, Wakame K, Nishioka H, Fujii H. Alleviating effect of active hexose correlated compound (AHCC) for anticancer drug-induced side effects in non-tumor-bearing mice. J Exp Ther Oncol. 2009; 8:43–51.
31. Sun B, Wakame K, Sato E, Nishioka H, Aruoma OI, Fujii H. The effect of active hexose correlated compound in modulating cytosine arabinoside-induced hair loss, and 6-mercaptopurine- and methotrexate-induced liver injury in rodents. Cancer Epidemiol. 2009; 33:293–299.


32. Hunter RJ, Fujii H, Wakame K, Gaikwad A, Wolf JK, Smith JA. Evaluation of active hexose correlated compound (AHCC) in combination with pegylated liposomal doxorubicin for treatment of ovarian cancer. Int J Appl Res Nat Prod. 2011; 4:6–14.
33. Fujii H, Nishioka N, Simon RR, Kaur R, Lynch B, Roberts A. Genotoxicity and subchronic toxicity evaluation of Active Hexose Correlated Compound (AHCC). Regul Toxicol Pharmacol. 2011; 59:237–250.


35. Morgan DA, Ruscetti FW, Gallo R. Selective in vitro growth of T lymphocytes from normal human bone marrows. Science. 1976; 193:1007–1008.


37. Dillman RO. The clinical experience with interleukin-2 in cancer therapy. Cancer Biother. 1994; 9:183–209.


38. Ferrante A. Activation of neutrophils by interleukins-1 and -2 and tumor necrosis factors. Immunol Ser. 1992; 57:417–436.
39. Zhu HG, Zollner TM, Klein-Franke A, Anderer FA. Activation of human monocyte/macrophage cytotoxicity by IL-2/IFN gamma is linked to increased expression of an antitumor receptor with specificity for acetylated mannose. Immunol Lett. 1993; 38:111–119.


40. Ibayashi Y, Tokuda Y, Saks ER, Sarna GP, Golub SH. In vivo and in vitro activation of NK cytotoxicity with IL-2. Prog Clin Biol Res. 1987; 244:275–285.
41. Chatzidakis I, Mamalaki C. T cells as sources and targets of TNF: implications for immunity and autoimmunity. Curr Dir Autoimmun. 2010; 11:105–118.


42. Yagita A, Maruyama S, Wakasugi S, Sukegawa Y. H-2 haplotype-dependent serum IL-12 production in tumor-bearing mice treated with various mycelial extracts. In Vivo. 2002; 16:49–54.
43. Yin Z, Fujii H, Walshe T. Effects of active hexose correlated compound on frequency of CD4+ and CD8+ T cells producing interferon-γ and/or tumor necrosis factor-α in healthy adults. Hum Immunol. 2010; 71:1187–1190.


44. Daddaoua A, Martínez-Plata E, López-Posadas R, Vieites JM, González M, Requena P, Zarzuelo A, Suárez MD, de Medina FS, Martínez-Augustin O. Active hexose correlated compound acts as a prebiotic and is antiinflammatory in rats with hapten-induced colitis. J Nutr. 2007; 137:1222–1228.


45. Li JH, Rosen D, Ronen D, Behrens CK, Krammer PH, Clark WR, Berke G. The regulation of CD95 ligand expression and function in CTL. J Immunol. 1998; 161:3943–3949.