Abstract
BACKGROUND/OBJECTIVES
Angelica keiskei is a green leafy vegetable rich in plant pigment phytochemicals such as flavonoids and carotenoids. This study examined bioavailability of flavonoids and carotenoids in Angelica keiskei and the alteration of the antioxidant performance in vivo.
SUBJECTS AND MATERIALS
Absorption kinetics of phytochemicals in Angelica keiskei were determined in healthy older adults (> 60 y, n = 5) and subjects with metabolic syndrome (n = 5). Subjects consumed 5 g dry Angelica keiskei powder encapsulated in gelatin capsules with a low flavonoid and carotenoid liquid meal. Plasma samples were collected at baseline, 0.5, 1, 2, 3, 4, 5, 6, 7, and 8 h. Samples were analyzed for flavonoids and carotenoids using HPLC systems with electrochemical and UV detection, respectively, and for total antioxidant performance by fluorometry.
RESULTS
After ingestion of Angelica keiskei increases in plasma quercetin concentrations were observed at 1-3 and 6-8 hr in the healthy group and at all time points in the metabolic syndrome group compared to baseline (P < 0.05). Plasma lutein concentrations were significantly elevated in both the healthy and metabolic syndrome groups at 8 hr (P < 0.05). Significant increases in total antioxidant performance were also observed in both the healthy and the metabolic syndrome groups compared to baseline (P < 0.05).
Angelica keiskei, a large perennial plant, which originated in Japan, belongs to the Umbeliferae family. Since identification of some constituents of the roots of Angelica keiskei by Kozawa et al in 1961 [1] and 1978 [2,3], its biological functions, including anti-carcinogenic [4,5,6], anti-thrombotic [7], anti-bacterial [8], anti-hyperglycemic [9], and anti-hypertensive effects [10] have been reported in various animal and cell models. Although consumption of Angelica keiskei-based juice for eight weeks was reported to reduce DNA damage in smokers [11], a study showing the bioavailability of phytochemicals in Angelica keiskei in humans is still lacking. It is generally accepted that the bioavailability of phenolics in plants is relatively low due to poor absorption, which is largely dependent on food matrix, sugar moieties, interaction with other constituents, and the site of absorption [12,13]. We recently reported major phytochemicals as well as various phenolics in Angelica keiskei using an integrated high resolution mass spectrometric and informatics approach [14], and the stability of its major components in different storage conditions [15].
Phytochemicals with antioxidant properties modify the antioxidant capacities in the lipid- and water-soluble compartments of the biological system in different degrees depending on its hydrophilicity vs. lipophilicity [16]. In addition, the combination of water-soluble and fat-soluble phytochemicals may well show synergistic protective effects against oxidative stress in both compartments due to their cross-talk [17]. Therefore, studying the changes of antioxidant status after ingestion of antioxidant rich Angelica keiskei along with its phytochemical status can be biologically significant. The Total Antioxidant Performance (TAP), developed by Aldini et al. [18] and validated by Beretta et al. [19], measures not only antioxidant capacity in both the hydrophilic and lipophilic compartments of the biological system, but also their synergistic/ cooperative interactions.
The metabolic syndrome is a constellation of interrelated metabolic abnormalities, including central obesity, hyperglycemia, hypertension, and dyslipidemia. The rapid increase in prevalence of metabolic syndrome [20,21] is projected to result in future increases in diabetes [22] and cardiovascular disease [23,24]. Individuals with metabolic syndrome show low serum antioxidant concentrations, such as carotenoids [25,26,27], which is attributed in part to elevated systemic oxidative stress and/or reduced utilization of consumed nutrients.
Therefore, a pilot study was conducted in order to determine the absorption kinetics of two major pigment phytochemicals in Angelica keiskei, quercetin and lutein, and changes in plasma total antioxidant performance in subjects with and without metabolic syndrome in preparation for a clinical trial with biochemical and clinical outcomes.
Ten older adults (> 60 years) identified as either healthy (n = 5) or as having metabolic syndrome (n = 5) were enrolled in the current study. For this study, 126 potential subjects were recruited, 121 subjects were prescreened using a questionnaire, 40 subjects were screened with a physical examination and blood test, and 10 qualified subjects were admitted and successfully completed the study. All study subjects were in good health as determined by a medical history questionnaire, physical examination, and normal results of complete blood count. Subjects had normal hematological parameters, serum albumin, liver function, and kidney function, did not have a history of smoking, alcoholism, or cardiovascular, hepatic, gastrointestinal, or renal diseases, and were not exogenous hormone users. Subjects did not take any supplemental vitamin or carotenoid for more than 6 wks before the start of the study and were not heavy tea drinkers (> 2 cups/d). Subjects with metabolic syndrome met the following parameters: 1) fasting plasma glucose ≥ 5.6 mmol/L; 2) central obesity, defined as a waist-hip ratio > 0.9 for men and > 0.85 for women; and 3) either high blood pressure (≥ 130/85 mmHg) or high triacylglycerol (≥ 1.7 mmol/L).
Subjects were supplemented with a one-time dose of 5 g of Angelica keiskei, equivalent to approximately 50 g of fresh vegetable, as a dry powder in a gelatin capsule. The supplement was taken with a low flavonoid and carotenoid beverage containing coconut milk, protein powder, ginger ale, and glucose, and plain pita bread with butter. This meal was repeated again at lunch. The macronutrient composition of this meal was: 534.2 kcal, 10.6 gm fat, 94.7 gm carbohydrate, and 15 gm protein. The carotenoid/phytochemical composition was minimal, and included 4 µg β-carotene, 24 µg lutein+zeaxanthin, 0.6 mg vitamin C, and 0.4 mg α-tocopherol equivalents. The nutrient composition of the diets was calculated with the use of Nutrition Dada System for Research (NDSR), Food and Nutrient Database Version 2008, University of Minnesota.
Blood samples (15 mL each) were taken from subjects at baseline (12-h fasting), and at 30, 60, 100, 180, 240, 300, 360, 420, and 480 min following ingestion of the supplement. Samples were protected from light and centrifuged for 15 min (1,000 × g, 4℃) within 1 h after collection. Aliquots of plasma were stored at -70℃ until analysis. The study protocol was approved by the Institutional Review Board of Tufts Medical Center and Tufts University Health Sciences (IRB#8636), and all subjects provided written informed consent to participate.
All-trans-β-carotene (type II), α-carotene, cryptoxanthin, lycopene, α-tocopherol, L-ascorbic acid, β-glucuronidase type II, quercetin, uric acid, human serum albumin, and phosphatidylcholine (type XVI-E) were purchased from Sigma Chemical Co. (St Louis, MO). Lutein was purchased from Kemin Industries (Des Moines, IA). The fatty acid analogue 4,4-difluoro-5-(4-phenyl-1,3-butadienyl)-4-bora-3a,4a-diaza-s-indacene-3-undeca noic acid (BODIPY 581/591) was purchased from Molecular Probes (Eugene, OR). The lipophilic radical initiator, 2,2'-azobis (4-methoxy-2,4-dimethylvaleronitrile) (MeO-AMVN) was purchased from Wako Chemicals (Richmond, VA, USA). Delipidized human serum (DHS) was purchased from SeraCare Life Sciences (Oceanside, CA). All HPLC solvents were obtained from JT Baker Chemical and were filtered through a 0.45-µm membrane filter before use.
Concentrations of plasma carotenoids and tocopherols were measured using an HPLC system as previously described with minor modifications [28]. Briefly, plasma samples (200 µL) were extracted with 2 mL of chloroform:methanol (2:1) followed by 3 mL of hexane. Samples were dried under nitrogen air and resuspended in 75 µL ethanol:methyl tert-butyl ether (2:1) of which 25 µL was injected into the HPLC. The HPLC system consisted of a Waters 2695 Separation Module, 2996 Photodiode Array Detector, a Waters 2475 Multi λ Fluorescence Detector, a C30 carotenoid column (3 µm, 150 × 3.0 mm, YMC, Wilmington, NC), and a Waters Millenium 32 data station. The mobile phase was methanol:methyl tert-butyl ether:water (85:12:3 by volume with 1.5 % ammonium acetate in water; solvent A) and methanol:methyl tert-butyl ether:water (8:90:2 by volume with 1 % ammonium acetate in water; solvent B). The gradient procedure has been previously reported [28]. Results were adjusted using an internal standard of echinenone. The coefficients of variation (CV) for inter assay (n = 25) was 4% and for intra assay was 4% (n = 9). Recovery of the internal standard averaged 97%.
Ascorbic acid was measured by an HPLC system using an electrochemical detector (Bioanalytical system, N. Lafayette, IN), as described by Behrens et al. [29]. Briefly, plasma sample was immediately deproteinized with perchloric acid (250 mM) and the supernatant was injected onto the HPLC system for ascorbic acid analysis.
Uric acid was measured using an Olympus analyzer (Olympus America Inc., Melvile, NY), as reported by Fossati et al. [30]. A series of uricase and peroxidase reactions were involved in this assay using 2,4,6,-tribromo-3-hydroxzy benzoic acid.
Concentrations of free plus phase II enzyme-conjugated flavonoids in plasma were determined using the HPLC method of Chen et al. [31]. Briefly, 20 µL of vitamin C-EDTA (200 mg ascorbic acid plus 1 mg EDTA in 1.0 mL 0.4 mol/L NaH2PO4, pH 3.6), 20 µL of a 5 mg/L internal standard (2',3',4'-trihydroxyacetophenone), and 20 µL of β-glucuronidase/sulfatase (98 units/L β-glucuronidase and 2.4 units/L sulfatase) were added to 200 µL of plasma and the mixture was incubated at 37℃ for 45 min. After enzyme digestion, flavonoids were extracted with 500 µL of acetonitrile, and then 500 µL supernatant was removed, dried under purified nitrogen, and reconstituted in 200 µL of an aqueous HPLC mobile phase. Flavonoids were determined using an HPLC system equipped with a Zorbax ODS C18 column (4.6 × 150 mm, 3.5 µm) and a Coularray 5600 A detector (ESA, Inc. Chelmsford, MA). The quantification of individual flavonoids was calculated according to calibration curves constructed using authentic standards (Sigma, St. Louis, MO), with linear relationships of R2 > 0.999. Analyses were performed in duplicate. The quantitation limit on the column for flavan-3-ols was 0.357 pmol. The CV of the intra- and inter-day assays was 3.0 and 9.0%, respectively.
Plasma total antioxidant performance (TAP) was determined using the method first developed by Aldini et al. [18] for measurement of total antioxidant capacity in both the hydrophilic and lipophilic compartments of plasma, and validated by Beretta et al [19]. It measures the rate of oxidation of 4, 4-difluoro-5-(4-phenyl-1, 3-butadienyl)-4-bora-3a,4a-diaza-s-indacene-3-undecanoic acid (BODIPY 581/591), a lipid-soluble fluorescent probe, and uses the lipid-soluble radical initiator 2,2'-azobis(4-methoxy-2,4-dimethylvaleronitrile) (MeO-AMVN). Oxidation is determined by monitoring the appearance of green fluorescence of the oxidation product of BODIPY (λex = 500 nm, λem = 520 nm) using a 1420 multilabel counter (Wallac Victor 2, Perkin Elmer Life Sciences, MA). The results are expressed as TAP values, which represent the percentage of inhibition of BODIPY oxidation in human serum with respect to that occurring in a control sample consisting of BODIPY 581/591 in phosphatidylcholine liposomes. Control samples were prepared using liposomes (phosphatidyl choline 2.5 mg/ml) in delipidized human serum.
Angelica keiskei dry powder encapsulated in gelatin capsules was provided by the National Institute of Agricultural Science and Technology, Korea. Carotenoids were analyzed as previously reported, with minor modifications [32]. Briefly, 100 mg lyophilized sample was used for carotenoid analysis. Samples were incubated with methanol for 2 hours at room temperature, followed by extraction with tetrahydrofuran at least four times. The lipid-soluble phytonutrients were quantified using high-performance liquid chromatography (HPLC) with a C30 column (3 lm, 150 × 4.6 mm, YMC, Wilmington, NC).The conditions of HPLC were the same as those used in measurement of the plasma.
Flavonoids were sequentially extracted twice with acidified methanol (methanol: glacial acetic acid:water, 50:3.7:46.3) from 100 mg of lyophilized samples over a 16-hour period. The combined extract was dried under nitrogen gas, and stored under nitrogen at -80℃. Prior to analysis by HPLC using an electrochemical detector (ECD), aglycones in the dry extract were obtained after sugar cleavage using an acid-boiling treatment as described previously [33]. Following solvent removal under nitrogen gas and reconstitution in HPLC mobile phase A, flavonoids were analyzed using the reverse phase HPLC-ECD method as described previously. The limit of quantitation for flavonoids determined by HPLC-ECD was 1 ng on the column. The linearities of calibration curves of authentic standards with concentrations range from 0.01 to 2 ng/ml were P ≥ 0.991.
Values are expressed as mean ± SEM. A repeated-measures analysis of variance with a Holm-Sidak multiple comparison tests was used to measure the effect of supplementation of Angelica keiskei on plasma quercetin, lutein concentrations and total antioxidant performance with respect to the baseline. When an equal variance test failed, Friedman repeated measures analysis of variance on ranks with Tukey's and multiple comparison tests were used. An unpaired t-test was used for comparison of differences in characteristics and antioxidant concentrations between the healthy and metabolic syndrome groups. Data analysis was performed using SigmaStat (Ver 3.1, Systat Software Inc, Point Richmond, CA).
The nutrient content of the Angelica keiskei consumed by study participants is shown in Table 1. This plant is a good source of antioxidant nutrients such as lutein, α-, trans β-, and 9-cis β- carotene and flavonoids; 5 g Angelica keiskei contains 2.07 mg of lutein, 1.08 mg of β-carotene, 5.75 mg of quercetin, and 2.57 mg of catechin.
Characteristics of the study subjects are shown in Table 2. Although the difference between the two groups was not significant, blood pressure tended to be higher in the metabolic syndrome group than in the healthy group. The metabolic syndrome group showed significantly higher average body mass index (BMI) (P < 0.01). Significantly higher levels of triglycerides and VLDL cholesterol were also observed in the metabolic syndrome group (P < 0.05). HDL cholesterol (P < 0.01) and adiponectin (P < 0.05) levels were significantly lower in metabolic syndrome subjects. The baseline plasma concentrations of phytochemicals in both groups are shown in Table 3. Significantly higher concentrations of uric acid and significantly lower concentrations of lutein and cryptoxanthin were observed in the metabolic syndrome group as compared to the healthy group (P < 0.05). The reduced ascorbic acid concentrations of the current study participants were in the range of 25-50% of National Health and Nutrition Examination Survey (NHANES III) serum total ascorbic acid concentration for the similar age group (50-70 yrs) [34]. Although samples were carefully collected and the reduced ascorbic acid concentrations were analyzed immediately after collecting the samples, there is a possibility that oxidation of reduced ascorbic acid might occur during the sample process. The plasma α-tocopherol concentrations of current study participants were in the range of 50-85% of National Health and Nutrition Examination Survey (NHANES III) serum vitamin E concentrations for the similar age group (50-70 yr) [34].
After ingestion of Angelica keiskei, the plasma quercetin concentrations of the healthy study participants (Fig. 1A) increased significantly at 1, 2, and 3 h as compared to baseline (P < 0.05), decreased after lunch at 4 and 5 h, and increased significantly again at 6 h, maintaining the significantly elevated level until 8 hours (P < 0.05). The plasma quercetin concentrations in the metabolic syndrome group were also significantly increased (P < 0.05) from the baseline throughout all periods (Fig. 1B). Changes of plasma lutein concentrations in the healthy (Fig. 2A) and metabolic syndrome groups are shown in Fig. 2 (Fig. 2B). The plasma lutein concentrations were significantly increased (P < 0.05) at 8 h in both healthy and metabolic syndrome groups as compared to those of the baseline after ingesting 5g of Angelica keiskei dry powder.
Changes in plasma total antioxidant performance (TAP) are shown in Fig. 3. In healthy study participants (Fig. 3A), the TAP values were significantly increased by 44 and 47% from the baseline (P < 0.05) at 1 and 3 h after ingestion of Angelica keiskei powder, decreased after lunch, and were again significantly elevated at 6 and 7 hours (P < 0.05). In the metabolic syndrome group (Fig. 3B), the values were significantly increased from the baseline at 30 minutes after ingestion of Angelica keskei, then fell slightly and showed another significant increase at 5 hours with a subsequent decrease (P < 0.05).
Findings of the current study demonstrate the bioavailability of pigment phytochemicals rich in Angelica keiskei and their ability to change antioxidant capacity in healthy elderly as well as subjects with metabolic syndrome.
Subjects exhibiting dyslipidemia (high triglyceride and low HDL cholesterol), elevated blood pressure and fasting glucose, and central obesity were classified as having metabolic syndrome [35], and the definition was used in the current study for inclusion of subjects in the metabolic syndrome. In the current study, due to the small number of subjects in each group, blood pressure and glucose level did not differ between the two groups. It is interesting to note that the metabolic syndrome group showed significantly lower adiponectin levels, consistent with its predictability of type II diabetes [36]. Consistent with the results of lower concentrations of carotenoids and vitamins C and E in individuals with metabolic syndrome shown in the Third National Health and Nutrition Examination Survey (NHANES III) [26], in the current study, we found that plasma concentrations of lutein and cryptoxanthin were significantly lower in individuals with metabolic syndrome. Similarly, a recent study examining 890 females and 646 males showed that the mean concentrations of serum α- and β-carotenes and the total concentration of the five carotenoids (α-carotene, β-carotene, β-cryptoxanthin, lutein/zeaxanthin, and lycopene) were significantly lower for subjects with metabolic syndrome compared to those without the syndrome [27]. Lower intakes of antioxidant-rich fruit and vegetables and corresponding decreased serum concentrations may contribute to progression of metabolic syndrome [37]. It is also plausible that the elevated oxidative stress in metabolic syndrome may cause increased utilization of antioxidants, resulting in lower serum concentrations of antioxidants such as carotenoids [25].
Quercetin, a flavonol, is one of the most prevalent flavonoids found in edible plants. In the current study, even though quercetin was not detected in the fasting blood before consumption of Angelica keiskei in both healthy and metabolic syndrome subjects, it should be pointed out that the baseline quercetin concentration has been reported to be approximately 50 nmol/L [13], slightly higher than the limit of quantification (33 nmol/L) of the current study. Previous studies have repeatedly demonstrated that the sugar moiety is a major determinant of the intestinal absorption of quercetin [13,38,39]. We observed that plasma quercetin level increased in healthy subjects within 1 hour after administration of Angelica keiskei, declined after lunch, and increased again in the sixth hour and remained elevated at 8 h after ingestion. Considering that 1) the current study determined the total quercetin concentrations in plasma after hydrolysis of conjugates formed from the phase II detoxification pathway and that 2) quercetin is usually bound to glucose and rutinose (a 6-o-rhamnosyl-glucose), the first peak at 1hr is probably due to quercetin glucoside and the 6 hr peak to quercetin rutinoside. This notion is supported by previous reports by Graefe et al, who reported on the pharmacokinetics of quercetin glucoside and quercetin rutinoside in humans [38]. Although subjects with metabolic syndrome also showed the quercetin peak at 30 min and a decrease at 5 hr, the plasma quercetin concentrations remained significantly high up to 8 hrs. It is possible that the altered gut microbiota [40] as well as phase 1 and II enzymes [41,42] may contribute to the differences in responses between healthy and metabolic syndrome subjects.
In the current study, a significant increase in plasma lutein was observed after ingestion of Angelica keiskei in both the healthy and metabolic syndrome groups. However, there was no significant alteration in plasma β-carotene concentrations in both the healthy and metabolic syndrome groups. Research indicates that absorption of carotenoids involves several factors, including interaction between carotenoids [43]. It has been reported that lutein may interfere with absorption of β-carotene and is absorbed more efficiently than β-carotene [44,45]. In addition, the two-fold larger amount of lutein than β-carotene in Angelica keiskei may affect the absorption kinetics of these carotenoids. A recent study reported that dietary phospholipid content enhanced the absorption of β-carotene and lutein [46]. However, it is unknown whether increased fat accumulation in individuals with metabolic syndrome enables such an effect. Considering that clearance of a one-time dose of pure carotenoid such as lutein or β-carotene has been shown to take more than 18 days [45], elevated plasma carotenoid concentration after consumption of Angelica keiskei would be maintained in circulation for an extended period of time. Due to the small number of subjects in each group and their large variations in response to Angelica keiskei consumption, there was no significant difference in plasma concentrations of quercetin or lutein in each time point between two groups.
In the current study, we observed an increase in antioxidant performance in healthy subjects as well as subjects with metabolic syndrome after ingestion of 5 g dry powder of Angelica keiskei. It is interesting to note that the significant changes of antioxidant capacity by physiologic dose of green leafy vegetable, Angelica keiskei, considering that endogenous plasma components such as protein and uric acid contribute greatly to overall antioxidant capacity and dietary micronutrients may, thus play a relatively small role, as discussed previously [47]. Active synergistic interactions among antioxidant phytochemicals within and across hydrophilic and lipophilic compartments of biological systems and various other phytochemicals in Angelica keiskei may contribute to the significant increase of antioxidant capacity. It may also be possible that the test meal itself might have altered plasma antioxidant capacity and such artifact could be overcome by a cross-over intervention study with a control meal without Angelica keiskei. However, in the current study, it is plausible that the meal consumed by participants may not affect the antioxidant capacity determined by a lipophilic approach [19] since it contained very low amounts of antioxidant nutrients (i.e. 0.6 mg vitamin C, 0.4 mg α-tocopherol, 24 µg lutein/zeaxanthin). Several studies failed to show the changes in various markers of antioxidant capacity such as the ferric-reducing ability of plasma (FRAP), trolox-equivalent antioxidant capacity (TEAC), and oxygen radical absorbance capacity (ORAC) after daily consumption of 600 g of fruit and vegetables [48] or 21-42 g of walnuts [49]. In the current study, it is plausible that the high contents of phytochemicals in Angelica keiskei as compared to other foods [15] and the low antioxidant status of older subjects who underwent two weeks of low fruit and vegetable diets prior to the intervention could contribute to the increase of antioxidant capacity. It should be noted however that the clinical significance of the alteration of antioxidant capacity and of the difference between healthy and metabolic syndrome subjects is not known.
Findings of the current study indicate the bioavailability of plant pigment phytochemicals such as carotenoid and flavonoid in Angelica keiskei and their association with increase of antioxidant capacity in both subjects with and without metabolic syndrome. Angelica keiskei can be beneficial for individuals under elevated systemic oxidative stress.
Figures and Tables
Fig. 1
Absorption kinetics of quercetin in plasma after ingesting 5 g of Angelica keiskei in older adults (aged ≥ 60 yr); healthy (A) and metabolic syndrome (B). Data are expressed as mean ± SEM (n = 5 in each group). ND, not detected. One-way repeated measures ANOVA with Tukey's multiple comparison was performed. When an equal variance test failed, Friedman repeated measures analysis of variance on ranks with Tukey's multiple comparison test was used. Significantly different as compared with the baseline *P < 0.05. Samples were analyzed by HPLC with ECD detection for quercetin.
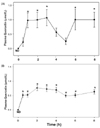
Fig. 2
Absorption kinetics of lutein in plasma after ingesting 5 g of Angelica keiskei in older adults (aged ≥ 60 yr); healthy (A) and metabolic syndrome (B). Data are expressed as mean ± SEM (n = 5 in each group). One-way repeated measures ANOVA with Tukey's multiple comparison was performed. Significantly different as compared with the baseline *P < 0.05. Samples were analyzed by HPLC with UV detection.
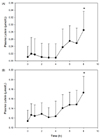
Fig. 3
Plasma total antioxidant performance after ingesting 5 g of Angelica keiskei in older adults (aged ≥ 60 yr); healthy (A) and metabolic syndrome (B). Data are expressed as mean ± SEM (n = 5 in each group). One-way repeated measures ANOVA with Tukey's multiple comparison was performed. When an equal variance test failed, Friedman repeated measures analysis of variance on ranks with Tukey's multiple comparison test was used. Significantly different as compared with the baseline *P < 0.05. Samples were analyzed by fluorometry.
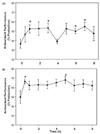
ACKNOWLEDGEMENT
We thank the volunteers who participated in this study, the Nutrition Evaluation Laboratory staff and the Metabolic Research Unit Staff at the Jean Mayer US Department of Agriculture Human Nutrition Research Center on aging at Tufts University.
References
1. Hata K, Kozawa M. Pharmacognostical studies on umbelliferous plants. XVIII. On the constituents of the roots of Angelica Keiskei Koidzumi. Yakugaku Zasshi. 1961; 81:1647–1649.


2. Kozawa M, Morita N, Baba K, Hata K. Chemical components of the roots of Angelica keiskei Koidzumi. II. The structure of the chalcone derivatives (author's transl). Yakugaku Zasshi. 1978; 98:210–214.


3. Kozawa M, Morita N, Baba K, Hata K. Chemical components of the roots of Angelica keiskei Koidzumi. III. The structure of a new dihydrofurocoumarin (author's transl). Yakugaku Zasshi. 1978; 98:636–638.


4. Motani K, Tabata K, Kimura Y, Okano S, Shibata Y, Abiko Y, Nagai H, Akihisa T, Suzuki T. Proteomic analysis of apoptosis induced by xanthoangelol, a major constituent of Angelica keiskei, in neuroblastoma. Biol Pharm Bull. 2008; 31:618–626.


5. Kimura Y. New anticancer agents: in vitro and in vivo evaluation of the antitumor and antimetastatic actions of various compounds isolated from medicinal plants. In Vivo. 2005; 19:37–60.
6. Akihisa T, Tokuda H, Hasegawa D, Ukiya M, Kimura Y, Enjo F, Suzuki T, Nishino H. Chalcones and other compounds from the exudates of Angelica keiskei and their cancer chemopreventive effects. J Nat Prod. 2006; 69:38–42.


7. Fujita T, Sakuma S, Sumiya T, Nishida H, Fujimoto Y, Baba K, Kozawa M. The effects of xanthoangelol E on arachidonic acid metabolism in the gastric antral mucosa and platelet of the rabbit. Res Commun Chem Pathol Pharmacol. 1992; 77:227–240.
8. Inamori Y, Baba K, Tsujibo H, Taniguchi M, Nakata K, Kozawa M. Antibacterial activity of two chalcones, xanthoangelol and 4-hydroxyderricin, isolated from the root of Angelica keiskei KOIDZUMI. Chem Pharm Bull (Tokyo). 1991; 39:1604–1605.


9. Kawabata K, Sawada K, Ikeda K, Fukuda I, Kawasaki K, Yamamoto N, Ashida H. Prenylated chalcones 4-hydroxyderricin and xanthoangelol stimulate glucose uptake in skeletal muscle cells by inducing GLUT4 translocation. Mol Nutr Food Res. 2011; 55:467–475.


10. Shimizu E, Hayashi A, Takahashi R, Aoyagi Y, Murakami T, Kimoto K. Effects of angiotensin I-converting enzyme inhibitor from Ashitaba (Angelica keiskei) on blood pressure of spontaneously hypertensive rats. J Nutr Sci Vitaminol (Tokyo). 1999; 45:375–383.


11. Kang MH, Park YK, Kim HY, Kim TS. Green vegetable drink consumption protects peripheral lymphocytes DNA damage in Korean smokers. Biofactors. 2004; 22:245–247.


12. Williamson G, Manach C. Bioavailability and bioefficacy of polyphenols in humans. II. Review of 93 intervention studies. Am J Clin Nutr. 2005; 81:243S–255S.


13. Manach C, Williamson G, Morand C, Scalbert A, Rémésy C. Bioavailability and bioefficacy of polyphenols in humans. I. Review of 97 bioavailability studies. Am J Clin Nutr. 2005; 81:230S–242S.


14. Aldini G, Regazzoni L, Pedretti A, Carini M, Cho SM, Park KM, Yeum KJ. An integrated high resolution mass spectrometric and informatics approach for the rapid identification of phenolics in plant extract. J Chromatogr A. 2011; 1218:2856–2864.


15. Li L, Aldini G, Carini M, Oliver Chen CY, Chun HK, Cho SM, Park KM, Correa CR, Russell RM, Blumberg JB, Yeum KJ. Characterisation, extraction efficiency, stability and antioxidant activity of phytonutrients in Angelica keiskei. Food Chem. 2009; 115:227–232.


16. Yeum KJ, Aldini G, Chung HY, Krinsky NI, Russell RM. The activities of antioxidant nutrients in human plasma depend on the localization of attacking radical species. J Nutr. 2003; 133:2688–2691.


17. Yeum KJ, Beretta G, Krinsky NI, Russell RM, Aldini G. Synergistic interactions of antioxidant nutrients in a biological model system. Nutrition. 2009; 25:839–846.


18. Aldini G, Yeum KJ, Russell RM, Krinsky NI. A method to measure the oxidizability of both the aqueous and lipid compartments of plasma. Free Radic Biol Med. 2001; 31:1043–1050.


19. Beretta G, Aldini G, Facino RM, Russell RM, Krinsky NI, Yeum KJ. Total antioxidant performance: a validated fluorescence assay for the measurement of plasma oxidizability. Anal Biochem. 2006; 354:290–298.


20. Ford ES, Giles WH, Mokdad AH. Increasing prevalence of the metabolic syndrome among U.S. adults. Diabetes Care. 2004; 27:2444–2449.


21. Ervin RB. Prevalence of metabolic syndrome among adults 20 years of age and over, by sex, age, race and ethnicity, and body mass index: United States, 2003-2006. Natl Health Stat Report. 2009; 1–7.
22. Ford ES, Schulze MB, Pischon T, Bergmann MM, Joost HG, Boeing H. Metabolic syndrome and risk of incident diabetes: findings from the European Prospective Investigation into Cancer and Nutrition-Potsdam Study. Cardiovasc Diabetol. 2008; 7:35.


23. Grundy SM. A constellation of complications: the metabolic syndrome. Clin Cornerstone. 2005; 7:36–45.
24. Malone DC, Boudreau DM, Nichols GA, Raebel MA, Fishman PA, Feldstein AC, Ben-Joseph RH, Okamoto LJ, Boscoe AN, Magid DJ. Association of cardiometabolic risk factors and prevalent cardiovascular events. Metab Syndr Relat Disord. 2009; 7:585–593.


25. Hansel B, Giral P, Nobecourt E, Chantepie S, Bruckert E, Chapman MJ, Kontush A. Metabolic syndrome is associated with elevated oxidative stress and dysfunctional dense high-density lipoprotein particles displaying impaired antioxidative activity. J Clin Endocrinol Metab. 2004; 89:4963–4971.


26. Ford ES, Mokdad AH, Giles WH, Brown DW. The metabolic syndrome and antioxidant concentrations: findings from the Third National Health and Nutrition Examination Survey. Diabetes. 2003; 52:2346–2352.
27. Coyne T, Ibiebele TI, Baade PD, McClintock CS, Shaw JE. Metabolic syndrome and serum carotenoids: findings of a cross-sectional study in Queensland, Australia. Br J Nutr. 2009; 102:1668–1677.


28. Zhao X, Aldini G, Johnson EJ, Rasmussen H, Kraemer K, Woolf H, Musaeus N, Krinsky NI, Russell RM, Yeum KJ. Modification of lymphocyte DNA damage by carotenoid supplementation in postmenopausal women. Am J Clin Nutr. 2006; 83:163–169.


29. Behrens WA, Madère R. A highly sensitive high-performance liquid chromatography method for the estimation of ascorbic and dehydroascorbic acid in tissues, biological fluids, and foods. Anal Biochem. 1987; 165:102–107.


30. Fossati P, Prencipe L, Berti G. Use of 3,5-dichloro-2-hydroxybenzenesulfonic acid/4-aminophenazone chromogenic system in direct enzymic assay of uric acid in serum and urine. Clin Chem. 1980; 26:227–231.


31. Chen CY, Milbury PE, Lapsley K, Blumberg JB. Flavonoids from almond skins are bioavailable and act synergistically with vitamins C and E to enhance hamster and human LDL resistance to oxidation. J Nutr. 2005; 135:1366–1373.


32. Cho YS, Yeum KJ, Chen CY, Beretta G, Tang G, Krinsky NI, Yoon S, Lee-Kim YC, Blumberg JB, Russell RM. Phytonutrients affecting hydrophilic and lipophilic antioxidant activities in fruits, vegetables and legumes. J Sci Food Agric. 2007; 87:1096–1107.


33. Zhang Z, Kou X, Fugal K, McLaughlin J. Comparison of HPLC methods for determination of anthocyanins and anthocyanidins in bilberry extracts. J Agric Food Chem. 2004; 52:688–691.


34. Institute of Medicine. Dietary Reference Intakes for Vitamin C, Vitamin E, Selenium, and Carotenoids. Washington, D.C.: The National Academies Press;2000.
35. Alberti KG, Eckel RH, Grundy SM, Zimmet PZ, Cleeman JI, Donato KA, Fruchart JC, James WP, Loria CM, Smith SC Jr. International Diabetes Federation Task Force on Epidemiology and Prevention. Hational Heart, Lung, and Blood Institute. American Heart Association. World Heart Federation. International Atherosclerosis Society. International Association for the Study of Obesity. Harmonizing the metabolic syndrome: a joint interim statement of the International Diabetes Federation Task Force on Epidemiology and Prevention; National Heart, Lung, and Blood Institute; American Heart Association; World Heart Federation; International Atherosclerosis Society; and International Association for the Study of Obesity. Circulation. 2009; 120:1640–1645.
36. Torres S, Salgado-Ceballos H, Torres JL, Orozco-Suarez S, Díaz-Ruíz A, Martínez A, Rivera-Cruz M, Ríos C, Lara A, Collado C, Guizar-Sahagún G. Early metabolic reactivation versus antioxidant therapy after a traumatic spinal cord injury in adult rats. Neuropathology. 2010; 30:36–43.


37. Montonen J, Knekt P, Järvinen R, Reunanen A. Dietary antioxidant intake and risk of type 2 diabetes. Diabetes Care. 2004; 27:362–366.


38. Graefe EU, Wittig J, Mueller S, Riethling AK, Uehleke B, Drewelow B, Pforte H, Jacobasch G, Derendorf H, Veit M. Pharmacokinetics and bioavailability of quercetin glycosides in humans. J Clin Pharmacol. 2001; 41:492–499.


39. Wiczkowski W, Romaszko J, Bucinski A, Szawara-Nowak D, Honke J, Zielinski H, Piskula MK. Quercetin from shallots (Allium cepa L. var. aggregatum) is more bioavailable than its glucosides. J Nutr. 2008; 138:885–888.


40. Vijay-Kumar M, Aitken JD, Carvalho FA, Cullender TC, Mwangi S, Srinivasan S, Sitaraman SV, Knight R, Ley RE, Gewirtz AT. Metabolic syndrome and altered gut microbiota in mice lacking Toll-like receptor 5. Science. 2010; 328:228–231.


41. Dey A, Williams RS, Pollock DM, Stepp DW, Newman JW, Hammock BD, Imig JD. Altered kidney CYP2C and cyclooxygenase-2 levels are associated with obesity-related albuminuria. Obes Res. 2004; 12:1278–1289.


42. Furukawa S, Fujita T, Shimabukuro M, Iwaki M, Yamada Y, Nakajima Y, Nakayama O, Makishima M, Matsuda M, Shimomura I. Increased oxidative stress in obesity and its impact on metabolic syndrome. J Clin Invest. 2004; 114:1752–1761.


43. van Het Hof KH, West CE, Weststrate JA, Hautvast JG. Dietary factors that affect the bioavailability of carotenoids. J Nutr. 2000; 130:503–506.


44. van den Berg H. Effect of lutein on beta-carotene absorption and cleavage. Int J Vitam Nutr Res. 1998; 68:360–365.
45. Kostic D, White WS, Olson JA. Intestinal absorption, serum clearance, and interactions between lutein and beta-carotene when administered to human adults in separate or combined oral doses. Am J Clin Nutr. 1995; 62:604–610.


46. Marisiddaiah R, Baskaran V. Bioefficacy of beta-carotene is improved in rats after solubilized as equimolar dose of beta-carotene and lutein in phospholipid-mixed micelles. Nutr Res. 2009; 29:588–595.


47. Talegawkar SA, Beretta G, Yeum KJ, Johnson EJ, Carithers TC, Taylor HA Jr, Russell RM, Tucker KL. Total antioxidant performance is associated with diet and serum antioxidants in participants of the diet and physical activity substudy of the Jackson Heart Study. J Nutr. 2009; 139:1964–1971.


48. Dragsted LO, Pedersen A, Hermetter A, Basu S, Hansen M, Haren GR, Kall M, Breinholt V, Castenmiller JJ, Stagsted J, Jakobsen J, Skibsted L, Rasmussen SE, Loft S, Sandström B. The 6-a-day study: effects of fruit and vegetables on markers of oxidative stress and antioxidative defense in healthy nonsmokers. Am J Clin Nutr. 2004; 79:1060–1072.

