Abstract
BACKGROUND/OBJECTIVES
Artemisinin (AT), an active compound in Arternisia annua, is well known as an anti-malaria drug. It is also known to have several effects including anti-oxidant, anti-inflammation, and anti-cancer activities. To date, the effect of AT on vascular disorders has not been studied. In this study, we investigated the effects of AT on the migration and proliferation of vascular smooth muscle cells (VSMC) stimulated by platelet-derived growth factor BB (PDGF-BB).
MATERIALS/METHODS
Aortic smooth muscle cells were isolated from Sprague-Dawley rats. PDGF-BB stimulated VSMC migration was measured by the scratch wound healing assay and the Boyden chamber assay. Cell viability was determined by using an EZ-Cytox Cell Viability Assay Kit. The production of reactive oxygen species (ROS) in PDGF-BB stimulated VSMC was measured through H2DCF-DA staining. We also determined the expression levels of signal proteins relevant to ROS, including measures of extracellular signal-regulated kinase (ERK) 1/2 measured by western blot analysis and matrix metalloproteinase (MMP) 9 measured by reverse transcription-polymerase chain reaction (RT-PCR).
RESULTS
AT (10 µM and 30 µM) significantly reduced the proliferation and migration of PDGF-BB stimulated VSMC in a dose-dependent manner. The production of ROS, normally induced by PDGF-BB, is reduced by treatment with AT at both concentrations. PDGF-BB stimulated VSMC treated with AT (10 µM and 30 µM) have reduced phosphorylation of ERK1/2 and inhibited MMP9 expression compared to untreated PDGF-BB stimulated VSMC.
Cardiovascular diseases, including vascular disorders, stroke, and atherosclerosis, are a major cause of death in western countries and are the second major cause of death in Koreans [1]. The pathological progression of vascular disease has a very complicated mechanism that includes the immune response and endothelial dysfunction in blood vessels [2]. Generally, the atherosclerosis patient has blood vessels narrowed by superabundant extracellular matrix (ECM) and vascular smooth muscle cells (VSMC) as well as atherosclerotic plaques [3].
The proliferation and migration of VSMC contributes to the pathogenesis of neo-intimal hyperplasia in atherosclerosis and restenosis [4]. Platelet-derived growth factor (PDGF) is a homodimer (AA and BB) and a heterodimer (AB) [5]. The abnormal motility of VSMC is stimulated by several growth factors such as insulin-like growth factor, transforming growth factor-β, and platelet-derived growth factor BB (PDGF-BB) [5,6,7]. Downstream from these pathways are reactive oxygen species (ROS), which are crucial for to regulating the abnormal motility of VSMC during arterial pathogenesis [8,9]. The mitogenactivated protein kinase(MAPK) signaling consists of the extra cellular signal-regulated kinase (ERK) 1/2, p38 MAPK, and the stress-activated protein kinase/c-Jun N-terminal kinase (JNK) [10]. Oxidative stress in blood vessels induces the abnormal migration of VSMC through signaling pathways such as MAPK pathway [11]. PDGF-BB induces phosphorylation of MAPK and ROS production [12]. Supporting the importance of this pathway, the developmental mechanism for vascular disorder is highly influenced by ERK1/2 phosphorylation [13].
Matrix metalloproteinase (MMP) 9 has been reported as a key enzyme in vascular pathogenesis and in embryogenesis [7]. Also, under the oxidative stress MMP9 contributes to the abnormal cell migration of VSMC that leads to ECM degradation and remodeling in the vascular endothelium [14].
Artemisinin (AT), an active compound in Arternisia annuais a widely used prescription medicine that is effective against malaria [15]. AT has several other effects including anti-oxidant, anti-inflammatory, and anti-cancer activities [16]. The effect of AT treatment on vascular disorders has not yet been reported. Hence, we attempted to investigate the anti-atherosclerotic effect of AT on PDGF-BB stimulated VSMC to provide fundamental data for nutraceutical development.
Purified artemisinin (AT) was purchased from Sigma (St. Louis, MO, USA). Cell culture materials were purchased from Gibco-BRL (Gaithersburg, MD, USA). The EZ-Cytox Cell Viability Assay Kit was purchased from Daeil Lab Service (Seoul, Korea). Recombinant PDFG-BB was obtained from R&D systems (Minneapolis, MN, USA). For analysis of western blots, specific antibodies for GAPDH, ERK1/2, and phospho-ERK1/2 were purchased from Santa Cruz Biotechnology (Santa Cruz, CA, USA). To measure ROS generation, the fluorescent dye 2',7'-dichlorodihydrofluorescein diacetate (H2DCFDA) was purchased from Invitrogen (Grand Island, NY, USA). Trizol was purchased from Invitrogen (Carlsbad, CA, USA). Ethidium bromide was purchased from Bio basic Inc. (Seoul, Korea). All other chemicals were purchased from Sigma (St. Louis, MO, USA).
Aortic VSMC were isolated from male Sprague-Dawley (SD) rats following previously described protocols [17]. VSMC were cultured in DMEM low glucose containing 10% fetal bovine serum (FBS), 100 U/mL penicillin, 100 g/mL streptomycin, and 200 mM glutamine at 37℃ under a humidified 95% air/5% CO2 mixture (v/v).
VSMC were seeded at 2 × 103 cells/well in a 96-well micro plate containing DMEM with 10% FBS, and incubated for 24 h. Cells were incubated with different concentrations of artemisinin (10 µM and 30 µM) and PDFG-BB (10 ng/mL) for 24 h. The proliferation rate was determined using an EZ-Cytox Cell Viability Assay Kit from the absorbance at 450 nm. Cell morphology was recorded using an inverted microscope (IX71; Olympus, Tokyo, Japan).
VSMC (2 × 104) were seeded a 6 well dish then incubated in serum-free media for 24 h. A transverse scratch wound on each monolayer of VSMC was made by using a sterilized 200 µL-tip. The scratch wounded VMSC monolayers were then stimulated with PDGF-BB (10 ng/mL) and various concentrations of artemisinin (0-30 µM) for an additional 24 h, at which point the transverse scratch wounds were re-examined and analyzed using image J software.
The PDGF-BB-mediated VSMC migration assay was performed using a Boyden chamber, as previously described [17].
Total RNA was isolated from VSMC using TRI-reagent according to the manufacturer's instructions. Synthesis of cDNA was performed using the ImProm II reverse transcription system™ also following the manufacturer's instructions. PCR conditions used for MMP9 and GAPDH RT-PCR were as follows: initial denaturation at 95℃ for 10 min, then 30 cycles of denaturation at 94℃ for 10 s, annealing at 58℃ for 30 s and, extension at 72℃ for 30 s. The primer sequences were as follows: MMP9, (sense) 5'-CCC TGC GTA TTT CCA TTC ATC-3' and (antisense) 5'-ACC CCA CTT CTT GTC AGC GTC-3'; GAPDH, (sense) 5'-TTC CAG TAT GAC TCT ACC CAC G-3' and (antisense) 5'-CAG CTT CTG AGT GGC AGT GA-3'
To analyze the level of protein expression, cell lysates were separated by electrophoresis using 12% acrylamide gels and then transferred to polyvinylidenedifluoride (PVDF) membranes in transfer buffer at 4℃ for 2 h. The membrane was treated with blocking solution (5% BSA in Tris-buffered saline) at room temperature for 1 h and then incubated overnight at 4℃ with antibodies to phosphorylated ERK1/2 (p-ERK1/2) or total ERK1/2 (T-ERK1/2) and GAPDH at 1:1000 dilution. The membranes were washed and then incubated with a 1:1000 dilution of anti-IgG secondary antibody conjugated to horseradish peroxidase. The expression levels of the proteins were analyzed via chemiluminescence and quantified using Image J Software.
The results were expressed as the mean ± standard error (SE) of at least three independent experiments (n≥ 3). The statistical differences between experimental groups were assessed using a Student's t-test and one-way ANOVA followed by Duncan's multiple range test. A P< 0.05 was considered to significance.
To determine the inhibitory effect of AT on PDGF-BB-induced cell proliferation we used an XTT assay. VSMC were pretreated with AT (10 µM and 30 µM) for 1 h, and then incubated in the presence or absence of PDGF-BB (10 ng/mL) for additional 24 h. As shown in Fig. 1B, PDGF-BB (10 ng/mL) increased VSMC proliferation by 90.7 ± 5.2% compared with the untreated group. In the presence of AT, PDGF-BB-induced VSMC proliferation was reduced to 41.9 ± 5.2% for the 10 µM treatment and to 80.2 ± 5.2% with 30 µM of AT (Fig. 1B).
Next, we assessed the inhibitory effect of AT on cell migration in the artificial scratch wound of PDGF-BB-induced VSMC. As shown in Fig. 2, AT significantly reduced cell numbers in the scratch wound area for PDGF-BB-stimulated VSMC. Migration was inhibited by the following percentages: 12.1 ± 3.1 for the 10 µM dose of AT and 56.5 ± 6.4 for 30 µM AT.
We performed the Boyden chamber assay to assess whether AT can inhibit cell migration in PDGF-BB-stimulated VSMC. VSMC were treated with AT (10 µM and 30 µM) and PDGF-BB (10 ng/mL) for 90 min. As shown in Fig. 3, the migration of VSMC increased to 270.1 ± 11.7% in the presence of PDGF-BB (10 ng/mL). PDGF-BB-induced cell migration was significantly inhibited by AT in a dose-dependent manner. In the presence of 10 µM AT migration decreased to 166.6 ± 2.7% and treatment with 30 µM AT reduced migration to 271.9 ± 4.9%.
We determined the effect of AT on production of ROS in PDGF-BB-stimulated VSMC through H2DCF-DA staining. VSMC were pretreated with AT (10 µM and 30 µM) for 1 h, and then treated with PDGF-BB (10 ng/mL) for additional 15 min. As shown in Fig. 4, PDGF-BB alone elevated ROS generation, whereas this effect was inhibited by 10 and 30 µM artemisinin (Fig. 4A). Next, we confirmed the inhibitory effect of AT on H2O2-induced migration of VSMC. H2O2 (100 µM) increased migration to 206.4 ± 31.4%, and the effect was reduced to 179.7 ± 15.4% and 233.0 ± 5.7% by 10 µM and 30 µM AT, respectively (Fig. 4B, C).
We tested whether AT inhibits the expression of phosphorylated ERK1/2 and MMP9 in PDGF-induced VSMC using western blot analysis and RT-PCR, respectively. As shown in Fig. 5, the phosphorylation of ERK1/2 was reduced in a dosedependent manner to 31.3 ± 2.3%, and 105.0 ± 6.0% after treatment with 10 µM, and 30 µM AT, respectively (Fig. 5A). In the case of MMP9 mRNA expression, the intensity of the PCR product in the PDGF-BB treated group was measured at 221.4 ± 15.7%, whereas in cells treated with AT intensity was 103.3 ± 3.0%, 12.6% in response to 10 µM and 30 µM AT, respectively (Fig. 5B)
It has been reported that Arternisia annua, called 'Gaeddongssuk' in Korea, exerts various effects against cancer, inflammation, and common pain [16]. Artemisinin (AT), an active compound in Arternisia annua, is a well-known anti-malaria drug [15]. In this study, we attempted to identify novel functions of AT, and our results showed that AT inhibited cell migration and proliferation in PDGF-BB-stimulated VSMC. Here, we first demonstrated the anti-atherosclerotic effect of AT, which was exerted through the ERK1/2 and MMP9 pathways. The physical data on migration of VSMC were obtained by using the scratch wound healing assay and Boyden chamber assay. Moreover, we showed direct evidence for signal molecule modulation demonstrated by western blot and reverse-transcription PCR (RT-PCR). This result implied that the antioxidant effect of AT could modulate PDGF-BB-induced VSMC migration.
We evaluated the migration of VSMC through the scratch wound healing assay at non-cytotoxic concentrations of AT (10 µM and 30 µM). We show that the closing rate on scratch wounds in PDGF-BB-stimulated VSMC is significantly reduced by AT. Moreover, we also observed the inhibitory effect of AT on proliferation in PDGF-BB-induced VSMC. Previous reports showed that AT could induce apoptosis in several cancer cell lines [18]. Therefore, we suggest that AT may be able to inhibit PDGF-BB-induced VSMC proliferation through apoptosis.
Abnormal migration of VSMC is a critical factor in the development of atherosclerosis or its early pathogenic progressive phase in the blood vessel [4]. Numerous studies have focused on the inhibition of cell migration in response to PDGF-BB [8, 18,19]. Our result showed that the migration of PDGF-BBstimulated VSMC was inhibited by AT in a dose-dependent manner. However, we wondered about the mechanism of action for AT, and how it inhibits VSMC migration. To answer this question, we evaluated cell migration in H2O2-stimulated VSMC using the Boyden chamber assay. Interestingly, AT showed an anti-migration effect on H2O2-stimulated VSMC and similarly reduced the effect of PDGF-BB on cell migration in a concentration-dependent manner. Moreover, we confirmed that AT attenuated intercellular ROS generation in PDGF-BBstimulated VSMC. Taken together, these data suggest that the anti-migratory activity of AT is involved in the ROS generation signaling pathway.
This result implied that AT could regulate cellular migration that was related to ROS. It has been reported that several growth factors generate ROS and phosphorylate MAPKs in VSMC, which can activate cell migration, proliferation, or the contraction and relaxation of vascular tissue [2,13]. Typically, PDGF is used to induce VSMC migration, which requires the phosphorylation of ERK 1/2 [12,13]. Previously, Jiang et. al. demonstrated the anti-migration mechanism of Luteolin, which as an antioxidant, reduced PDGF-BB generated reactive oxygen species (ROS) and phosphorylation of MAPK [20]. Konneh et. al. has been established to use vitamin E to care of in vitroand in vivo[21]. We suggest that the antioxidant effect of AT has decreased PDGF-BB-induced ERK1/2 phosphorylation in ROS-induced migration of VSMC.
Recently, a report showed that MAPK is involved in regulating MMP9 expression in response to the migration of PDGF-BB-induced VSMC [14]. We also showed that AT decreased PDGF-BB-induced MMP9 expression.
In conclusion, AT, as a ROS scavenger, could significantly inhibit the migration of VSMC by reducing the expression of MMP9 and suppression of ERK1/2 phosphorylation. Therefore, artemisinin could be helpful in treatment of atherosclerosis through either reducing the onset or suppressing abnormal proliferation and migration in vascular smooth muscle cells.
Figures and Tables
Fig. 1
The effect of artemisinin on proliferation in PDGF-BB-stimulated VSMC. (A) The chemical structure of artemisinin. (B) VSMC were seeded (2 × 103 cells/well) and incubated for 12 h. VSMC were incubated in serum-free media for 24 h and then cells were treated with the indicated concentrations of AT (10 µM and 30 µM) in the presence or absence of PDGF-BB for 24 h. The graph indicated the representative cell viability from three independent experiments. Data are mean ± standard error. Values with the same superscript letter are not significantly different by Duncan's multiple range test (P < 0.05).
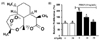
Fig. 2
Effects of AT on scratch wound healing in PDGF-BB-stimulated VSMC. VSMC were treated with AT (10 µM and 30 µM) and PDGF-BB (10 ng/mL) for 24 h. (A) Dotted white lines indicate the wounded area from the initial scratches. Magnification, × 200. Bar = 500 µm. The number in the top right of each image is concentration of PDGF-BB/concentration of AT. (B) Amount of migration relative to the untreated control (%). Results are presented as mean ± standard error. Values with the same superscript letter are not significantly different by Duncan's multiple range test (P < 0.05).
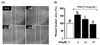
Fig. 3
Effects of AT on migration in PDGF-BB-stimulated VSMC. VSMC were treated with AT (10 µM and 30 µM) and PDGF-BB (10 ng/mL) for 90 min. (A) Migrating VSMC on membranes, the spots are Diff quick-stained cells. The number at the top right corner of each image is the concentration of PDGF-BB/concentration of AT. Magnification, × 200. Bar = 500 µm. (B) Migrated cell counts indicated relative to the untreated control (%). Results are presented as mean ± standard error. Values with the same superscript letter are not significantly different by Duncan's multiple range test (P < 0.05).
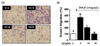
Fig. 4
Effects of AT on migration in H2O2-stimulated VSMC. (A) ROS production was assessed using 1 µg/mL DAPI (upper panel) or H2DCFDA (lower panel) in PDGF-BB-induced proliferating VSMC, Magnification, × 400. Bar = 50 µm. (B) Migrating VSMC on membranes, the spots are Hoescht stained cells. VSMC were treated with AT (10 µM and 30 µM) and H2O2 (100 µM) for 90 min. The number at the top right corner of each image is the concentration of H2O2/concentration of AT. Magnification, 200 ×. Bar = 500 µm. (C) Migrating cell number relative to the untreated control (%). Results are presented as mean ± standard error. Values with the same superscript letters are not significantly different by Duncan's multiple range test (P < 0.05).
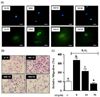
Fig. 5
Effect of AT on PDGF-BB-induced phosphorylation of ERK1/2 and MMP9 transcription in VSMC. (A) VSMC were pretreated with or without AT (10 µM and 30 µM) for 1 h and then stimulated with 10 ng/mL of PDGF-BB for 15 min. For statistical analysis, densitometry of the band representing the phosphorylated form of ERK1/2 normalized to the total expression of ERK is considered 100%. (B) The levels of MMP9 mRNA were determined by RT-PCR. VSMC were pretreated with or without AT (10 µM and 30 µM) for 1 h and then stimulated with 10 ng/mL of PDGF-BB for 6 h. cDNA was synthesized using 1 µg of total RNA each treatment group. After PCR and electrophoresis on a 1% agarose gel, the bands were measured using densitometry of the ethidium bromide stained DNA under UV light. The background intensity is considered 100%, and the expression of MMP9 is defined relative to the GAPDH control. Results are presented as the mean ± standard error of three independent experiments. Values with the same superscript letters are not significantly different by Duncan's multiple range test (P < 0.05).
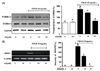
References
1. Ministry of Health and Welfare, Korea Centers for Disease Control and Prevention. Korea Health Statistics 2012: Korea National Health and Nutrition Examination Survey (KNHANES V-3). Cheongwon: Korea Centers for Disease Control and Prevention;2013.
2. Buttari B, Profumo E, Businaro R, Saso L, Capoano R, Salvati B, Riganò R. Oxidized haemoglobin-driven endothelial dysfunction and immune cell activation: novel therapeutic targets for atherosclerosis. Curr Med Chem. 2013; 20:4806–4814.


3. Ross R. The pathogenesis of atherosclerosis: a perspective for the 1990s. Nature. 1993; 362:801–809.


4. Shah PK. Inflammation, neointimal hyperplasia, and restenosis: as the leukocytes roll, the arteries thicken. Circulation. 2003; 107:2175–2177.
5. Kondo T, Konishi F, Inui H, Inagami T. Differing signal transductions elicited by three isoforms of platelet-derived growth factor in vascular smooth muscle cells. J Biol Chem. 1993; 268:4458–4464.


6. Ross R, Raines EW, Bowen-Pope DF. The biology of platelet-derived growth factor. Cell. 1986; 46:155–169.


7. Park SS, Kim WJ, Moon SK. Role of decursin in TNF-α-stimulated migration, invasion, and matrix metalloproteinase-9 induction in vascular smooth muscle cells. Food Sci Biotechnol. 2011; 20:913–918.


8. Moon CY, Ku CR, Cho YH, Lee EJ. Protocatechuic aldehyde inhibits migration and proliferation of vascular smooth muscle cells and intravascular thrombosis. Biochem Biophys Res Commun. 2012; 423:116–121.


9. Lyle AN, Griendling KK. Modulation of vascular smooth muscle signaling by reactive oxygen species. Physiology (Bethesda). 2006; 21:269–280.


10. Kyaw M, Yoshizumi M, Tsuchiya K, Kirima K, Tamaki T. Antioxidants inhibit JNK and p38 MAPK activation but not ERK 1/2 activation by angiotensin II in rat aortic smooth muscle cells. Hypertens Res. 2001; 24:251–261.


11. Zhou Y, Yan H, Guo M, Zhu J, Xiao Q, Zhang L. Reactive oxygen species in vascular formation and development. Oxid Med Cell Longev. 2013; 2013:374963.


12. Park J, Ha H, Seo J, Kim MS, Kim HJ, Huh KH, Park K, Kim YS. Mycophenolic acid inhibits platelet-derived growth factor-induced reactive oxygen species and mitogen-activated protein kinase activation in rat vascular smooth muscle cells. Am J Transplant. 2004; 4:1982–1990.


13. Bornfeldt KE, Raines EW, Nakano T, Graves LM, Krebs EG, Ross R. Insulin-like growth factor-I and platelet-derived growth factor-BB induce directed migration of human arterial smooth muscle cells via signaling pathways that are distinct from those of proliferation. J Clin Invest. 1994; 93:1266–1274.


14. Visse R, Nagase H. Matrix metalloproteinases and tissue inhibitors of metalloproteinases: structure, function, and biochemistry. Circ Res. 2003; 92:827–839.
15. Kohler M, Haerdi W, Christen P, Veuthey JL. Extraction of artemisinin and artemisinic acid from Artemisia annua L. using supercritical carbon dioxide. J Chromatogr A. 1997; 785:353–360.


16. Ho WE, Peh HY, Chan TK, Wong WS. Artemisinins: pharmacological actions beyond anti-malarial. Pharmacol Ther. 2014; 142:126–139.


17. Won KJ, Lee SC, Lee CK, Lee HM, Lee SH, Fang Z, Choi OB, Jin M, Kim J, Park T, Choi WS, Kim SK, Kim B. Cordycepin attenuates neointimal formation by inhibiting reactive oxygen species-mediated responses in vascular smooth muscle cells in rats. J Pharmacol Sci. 2009; 109:403–412.


18. Singh NP, Lai HC. Artemisinin induces apoptosis in human cancer cells. Anticancer Res. 2004; 24:2277–2280.
19. Choi OB, Park JH, Lee YJ, Lee CK, Won KJ, Kim J, Lee HM, Kim B. Olibanum extract inhibits vascular smooth muscle cell migration and proliferation in response to platelet-derived growth factor. Korean J Physiol Pharmacol. 2009; 13:107–113.

