Abstract
BACKGROUND/OBJECTIVES
The root of Vitis amurensis Ruprecht, a sort of wild-growing grape, has been used in oriental medicine for treatment of skin ailments; however, its dermatological activity is not sufficiently understood. The aim of this study was to investigate tyrosinase inhibitory and anti-melanogenic activities of V. amurensis Ruprecht root methanol extract (VARM) in B16F10 mouse melanoma cells and to attempt to isolate and identify the active compound issued from VARM.
MATERIALS/METHODS
Anti-melanogenic activity of VARM was analyzed in α-melanocyte stimulating hormone (MSH)-stimulated B16F10 cells through evaluation of antioxidative activity as well as inhibited tyrosinase activity and melanin contents compared with those of kojic acid and arbutin. After anti-melanogenic analysis of VARM, serial fractionation, nuclear magnetic resonance (NMR), and thin layer chromatorgraphy (TLC) were applied for identification of active compounds contained in VARM.
RESULTS
VARM significantly inhibited oxidative stress and tyrosinase activity and attenuated α-MSH-induced melanin production in B16F10 cells. For isolation of active compounds, VARM was fractionated using a series of organic solvents, including dichloromethane (CH2Cl2), ethyl acetate (EtOAc), and n-butanol (n-BuOH). Among fractions showing anti-melanogenic activity, the CH2Cl2 fraction induced the most potent attenuation of melanogenesis without cytotoxicity and the major compound in the CH2Cl2 fraction was identified as betulinic acid. Betulinic acid isolated from the CH2Cl2 fraction of VARM significantly attenuated α-MSH-induced melanogenesis in a dose dependent manner, which was stronger than that of arbutin used as a positive control.
Melanin, a naturally synthesized pigment in melanocytes, is stored inside the melanosome, which determines the color of human skin, hair, and eyes [1]. Melanin is originally produced to protect skin from ultraviolet (UV) radiation, however, overproduction and accumulation can cause various dermatological disorders such as melanoma, freckles, age spots, and other hyperpigmentation syndromes [2,3]. Melanogenesis is a complicated pathway made up of melanin synthesis, melanin transport, and melanosome release [4]. This cascade of melanogenesis is stimulated by a variety of environmental biochemical factors, α-melanocyte stimulating hormone (α-MSH), cyclic AMP (cAMP) elevating agents, including forskolin, glycyrrhizin, isobutylmethylxanthine, and UVB exposure [5,6,7,8,9]. Among them, α-MSH, a naturally occurring melanotropic peptide, has been used as a melanogenesis inducer in many studies [10,11,12].
Enzymatic reaction for melanogenesis is associated with tyrosinase, TRP-1 (dopachrome tautomerase), and TRP-2 (DHICA oxidase) [10,13]. Tyrosinase, the critical and rate-limiting enzyme involved in the first two-step reaction of melanogenesis, hydroxylates tyrosine to 3,4-dihydroxyphenylalanine (L-DOPA) and the subsequent oxidation of L-DOPA to dopaquinone. Dopaquinone is auto-oxidized into dopachrome, which is converted to dihyroxyindole (DHI) or dihydroxyindole-2-carboxylic acid (DHICA) for production of black eumelanins, whereas similar reactions related to cysteine and glutathione conjugates of dopaquinone yield reddish-brown pheomelanins [14]. In addition, hydrogen peroxide (H2O2) and other reactive oxygen species (ROS) are synthesized during melanogenesis, which functions to enhance pigment formation by regulating levels of tyrosine [15]. Therefore, development of an effective tyrosinase inhibitor with antioxidative capacity can be a promising strategy for a potential anti-melanogenic agent.
Vitis amurensis Ruprecht, a species of grape native to Asian countries, exhibited various biological activities, including antioxidative [16,17,18], neuroprotective [19,20,21], anti-tumorogenic [22,23], antimicrobial [24], and anti-inflammatory activities [25,26]. Hence, many researchers have attempted to identify its functional compounds, which were proved as phenolic compounds [17,27], procyanidin [28], oligostilbenes such as amurensin A, B, and G [29,30], and stilbenes such as resveratrol and its tetramer, heyneanol A [19,22]. In addition, root of V. amurensis has also been used as a medicinal herb in oriental medicine. Although root of V. amurensis has been reported to possess anti-tumorigenic [22,31] and anti-inflammatory activity [32], no investigation of anti-melanogenic activity has been reported.
The aim of the current study is to investigate anti-oxidative and anti-melanogenic effects of V. amurensis Ruprecht root methanol extract (VARM) in B16F10 cells, and then identify its functional compounds among various solvent fractions.
1,1-diphenyl-2-picryl hydrazyl (DPPH), mushroom tyrosinase, α-MSH, L-DOPA, and betulinic acid were purchased from Sigma (St. Louis, MO, USA). Dulbecco's modified Eagle medium (DMEM), fetal bovine serum (FBS), and L-glutamine were obtained from Invitrogen Corporation (Carlsbad, CA, USA). Antibodies were purchased from Santa Cruz Biotechnology (Santa Cruz, CA, USA) for tyrosinase, actin, and secondary antibodies. Nitrocellulose membrane was purchased from GE Healthcare (Little Chalfont, Buckinghamshire, UK). All other chemicals were of the highest commercial grade available.
V. amurensis Ruprecht root, which was purchased from Daehan Natural Drug (Busan, Republic of Korea), originated from Yeong cheon in Korea. Dried powder of V. amurensis Ruprecht root (5 kg) was extracted four times with five volumes of absolute (99.8%) methanol at 65℃. The extract was evaporated, freeze dried, and stored at 4℃ until use. Methanol extract of V. amurensis Ruprecht root (VARM, 527.6 g) was suspended in water and serially fractionated into dichloromethane (CH2Cl2, C fraction, 21.3 g), ethyl acetate (EtOAc, E fraction, 81.1 g), n-butanol (n-BuOH, B fraction, 71.3 g), and water (H2O, W fraction, 246.1 g). The C fraction, which exhibited potent anti-melanogenic activity without cytotoxicity, was applied onto a silica gel (70-230 mesh, Merk, Darmstadt, Germany) column. The column was eluted using mixtures of n-hexane:EtOAc under gradient conditions [10:1, 5:1, 3:1, 2:1, 1:1 (v/v)] to yield the four fractions (fraction 1 to 4). Among four fractions, fraction 3 was further purified by a silica gel once more to yield six fractions (fraction 1 to 6). Among them, fraction 3 was identified as a betulinic acid through 1H nuclear magnetic resonance (NMR) and 13C-NMR analyses. For identification of betulinic acid, TLC was performed on a precoated Merck Kieselgel 60 F254 plate (0.25 mm) and the spots were compared with standard betulinic acid purchased from Sigma.
Antioxidative activity of VARM and its solvent fractions were determined using a DPPH radical scavenging assay as described previously [33]. Various concentrations of reagents and standard compound (Ascorbic acid) were added to DPPH solution. Absorbance was measured at 520 nm after 30 min incubation protected from light.
The effect of VARM on mushroom tyrosinase activity was determined as previously described [34]. In brief, 50 µL of mushroom tyrosinase (700 unit/mL), 50 µL of various concentrations of reagents, and 100 µL of 0.1 M phosphate buffer (pH 6.8) were added to a 96-well plate and mixed, 100 µL of 2.5 mML-DOPA was added, followed by incubation for 20 min at room temperature. The amount of dopachrome formed in the reaction mixture was measured at 475 nm. Kojic acid was used as a positive control.
B16F10 murine melanoma cells (CRL-6475) were obtained from the American Type Culture Collection (ATCC, Rockville, MD, USA). Cells were maintained in DMEM supplemented with 10% FBS and 100 units/mL of penicillin/streptomycin at 37℃ in a humidified atmosphere containing 5% CO2. Cells were treated with either α-MSH alone or reagents together. Cells not treated with α-MSH served as the negative control, while cells treated with α-MSH alone served as the positive control.
Cell viability was determined using a 3-(4,5-dimethylthiazol-2-yl)-5-(3-carboxymethoxyphenyl)-2-(4-sulfophenyl)-2H-tetrazolium, inner salt (MTS) assay purchased from Daeil Lab Service (Seoul, Korea) according to the manufacturer's instructions [35]. After treatment with various concentrations of VARM and its solvent fractions, cells were incubated with MTS for 1 h and the viability was quantified by measurement at OD450 using a multi-plate reader (Paradigm, Beckman, CA, USA).
Effect of VARM and its solvent fractions on α-MSH induced melanogenesis on B16F10 cells was investigated according to the previous research, which was slightly modified [36]. Briefly, cells were stimulated with α-MSH (200 nM) and treated with VARM and its solvent fractions for 72 hr. After treatment, the cells were dissolved in 1 N NaOH/10% DMSO for 1 hr at 80℃, and solubilized melanin was measured at 475 nm.
Effect of VARM and its solvent fractions on α-MSH induced tyrosinase activity on B16F10 cells was investigated according to the previous research, which was slightly modified [36]. Briefly, cells were stimulated with α-MSH (200 nM) and treated with VARM and its solvent fractions for 72 hr. After treatment, the cells were washed with 1 × phosphate buffered saline (PBS), and then lysed into protein lysis buffer (Cell Signaling Technology, Boston, MA, USA). Each extract was placed in each well of a 96-well plate and the enzymatic assay was commenced by addition of L-DOPA. After incubation, dopachrome formation was assayed by measuring absorbance at 475 nm.
After treatment, the cells were washed with ice-cold 1 × PBS and lysed with cell lysis buffer (pH 7.4). The cell lysates were incubated on ice for 30 min and the homogenates were centrifuged at 13,000 × g for 10 min at 4℃. The supernatants were collected and protein concentration was determined using the Bradford protein assay. Protein samples (50 µg) were mixed with 2 × loading buffer, and boiled at 100℃ for 5 min. The samples were separated on a 10% sodium dodecyl sulfate (SDS)-polyacrylamide gel and electrotransferred to nitrocellulose membranes (WhatmanTM, GE Healthcare, Little Chalfont, UK). The membranes were blocked with 5% nonfat dry milk in 1 × PBST buffer (0.1% Tween 20 in PBS) for 1 hr at room temperature and incubated overnight with primary antibodies in blocking buffer at 4℃. The membranes were washed, followed by incubation with anti-rabbit-IgG or anti-goat-IgG with horseradish peroxidase for 1 hr at room temperature. After washing the membrane, final detection and quantification were performed using a chemiluminescence detection system (FluoChem® FC2, Alpha Innotech, CA, USA).
Thus far, a potent DPPH scavenger among natural resources has been regarded as a potential antioxidant candidate. In this study, VARM was evaluated for its anti-oxidative activity by DPPH, a stable free radical, scavenging capacity. As shown in Fig. 1A, VARM scavenged free radicals in a dose dependent manner, with an inhibitory rate of 15.6, 50.2, and 95.8% at 2.56, 12.8, and 64 µg/mL, respectively. Dose for IC50 was calculated as 12.8 µg/mL. As shown in Fig. 1B, VARM suppressed the enzyme activity of mushroom tyrosinase, with an inhibitory rate of 6.7, 46.0, and 87.9% at 20, 100, and 500 µg/mL, respectively. The IC50 value was calculated as 148.1 µg/mL. IC50 values of DPPH radical scavenging and tyrosinase inhibitory activity issued from VARM-treated B16F10 cells are shown in Table 1.
The cytotoxicity of VARM for 48 hr was determined using a colorimetric MTS assay in B16F10 cells. Fig. 2A shows the percent cell viability of VARM-treated cells compared with that of vehicle treated cells. No remarkable cytotoxicity was observed in cells treated with VARM under the concentration of 200 µg/mL, which was applied for the following cascade of experiments. As shown in Fig. 2B, melanin content of α-MSH stimulated cells was 2.5 times higher than that of vehicle treated cells, indicating that melanogenesis was successfully induced by α-MSH treatment. On the other hand, α-MSH-stimulated melanin production was significantly (P < 0.5) inhibited by treatment with VARM in a dose-dependent manner (Fig. 2B).
VARM was serially fractionated with CH2Cl2, EtOAc, n-BuOH, and H2O for identification of active compounds possessing anti-melanogenic activity (Fig. 3). The effect of each fraction on B16F10 cell viability was evaluated in advance of the downstream experiment. As shown in Fig. 4A, VARM, C, and B fractions did not show any cytotoxicity below 250 µg/mL, while the E fraction exhibited a cytotoxic effect over 50 µg/mL. Followed by the result for cell viability, anti-melanogenic effect of each fraction was evaluated on non-cytotoxic doses. As shown in Fig. 4B, the C fraction induced the most potent inhibition of α-MSH induced melanogenesis among all fractions that exhibited an anti-melanogenic effect. In the W fraction, neither cytotoxic nor anti-melanogenic activities were detected.
To confirm the anti-melanogenic effect of VARM and its solvent fractions, cellular tyrosinase enzymatic activity and protein expression level were analyzed. As shown in Fig. 5A, elevated tyrosinase enzyme activity induced by α-MSH was down-regulated by VARM and its solvent fractions, including C, E, and B. In addition, tyrosinase protein expression was also inhibited by VARM and its solvent fractions, which was in accordance with melanin contents and tyrosinase activity (Fig. 5B). According to the results, anti-melanogenic effects of VARM and its solvent fractions originated from the inhibited tyrosinase activity. Among all fractions of VARM, the C fraction showed the most potent anti-melanogenic activity without cytotoxicity (Fig. 6A).
Identification and purification of the functional compounds contained in the C fraction, which showed the most potent anti-melanogenic activity, was attempted. As a result, various compounds were identified as methyl palmitate, palmitate, methyl stearate, oleanolic acid, and betulinic acid. Among them, only betulinic acid significantly attenuated α-MSH-induced melanogenesis in a dose dependent manner, which was stronger than that of arbutin, a well-known depigmenting agent (Fig. 6B).
Appropriate melanogenesis provides an effective protection mechanism against harmful ultraviolet radiation; however, abnormal production of melanin and accumulation lead to various dermatological disorders. Therefore, many scientists have attempted to find new agents to alleviate hyperpigmentation and melanin accumulation. In this study, anti-melanogenic activity of V. amurensis Reprecht root was evaluated through analysis of tyrosinase and melanin content in α-MSH stimulated B16F10 cells. In addition, isolation and identification of the active compound possessing anti-melanogenic activity in V. amurensis Reprecht root was attempted.
During melanogenesis stimulatory cascade, α-MSH is an important component in both human melanocytes and murine melanoma cells [37]. Melanocortin 1 receptor (MC1R) bound to α-MSH regulates melanocytic pigmentation through modulation of G protein-coupled receptor (GPCR)-cAMP-microphthalmia-associated transcription factor (MITF) signaling cascade. Once MITF was activated, downstream target genes including TRP-1 and 2, which play crucial role in melanogenesis, were activated serially [38]. Results of the current study indicate that α-MSH-initiated melanin synthesis was abolished by treatment with VARM and its solvent fractions, suggesting that V. amurensis Reprecht root possesses the potential to disrupt melanin production cascade in melanocytes.
Because tyrosinase is a rate-limiting enzyme related to the first two steps of melanogenesis, agents possessing tyrosinase inhibitory activity can be regarded as potential candidates for a depigmentation strategy [39]. Therefore, tyrosinase activity was analyzed for evaluation of the involved mechanisms of VARM and its solvent fractions-induced antimelanogenesis in B16F10 cells. In this study, two kinds of tyrosinases were investigated; an intracellular tyrosinase and a mushroom tyrosinase. Intracellular tyrosinase activity was analyzed by measurement of generated dopachrome in α-MSH stimulated B16F10 cells. Treatment with VARM and its solvent fractions resulted in significantly attenuated intracellular tyrosinase activity as evidenced by a decrease in the quantity of dopachrome. Mushroom tyrosinase activity was also analyzed to determine whether VARM and its solvent fractions-induced intracellular tyrosinase inhibitory effect originated directly from inhibited enzyme activity or disruption the enzyme synthesis pathway. In accordance with the result of intracellular tyrosinase assay, VARM and its solvent fractions attenuated dopachrome formation through modulation of mushroom tyrosinase activity, indicating direct inhibition of tyrosinase activity by VARM.
When the skin is exposed to UV radiation and other environmental oxidizing pollutants, largely generated ROS and reactive nitrogen species (RNS) can induce lipid peroxidation and enzyme inactivation in cutaneous tissue. Therefore, antioxidative compounds that can counteract oxidative damage have been considered as beneficial agents for skin. For example, oligomeric proanthocyanidins from grape seeds effectively inhibit UV-induced oxidative stress considered to promote melanogenesis [40]. VARM and its solvent fractions-induced melanin decrease may be attributed to its depletion of ROS and RNS.
These results suggest that V. amurensis Reprecht root possesses antimelanogenic activity by regulation of cellular tyrosinase activity in melanocytes. Therefore, an active compound contained in V. amurensis Reprecht root was isolated and confirmed as betulinic acid. Betulinic acid is a naturally occurring pentacyclic triterpenoid found in the bark of several species of plants [41]. Although various biological activities, including anti-inflammatory, antibacterial, and anticarcinogenic activities from various plants have been reported for betulinic acid, this is the first finding that not only does betulinic acid originate from V. amurensis Ruprecht root it also possesses anti-melanogenic activity [41,42,43,44]. Although melanogenesis inhibitory compounds were also found from Saussureae Radix, that was not betulinic acid but betulinic acid methyl ester. Furthermore that compound showed lower activity than arbutin used as a positive control [45].
In this study, anti-oxidative and anti-melanogenic activities of VARM and its solvent fractions were evaluated. VARM and all fractions possessed potent anti-melanogenic activities originating from the direct inhibition of cellular tyrosinase activity, which mainly originated from the active compound, betulinic acid. These results suggest that V. amurensis Ruprecht root and betulinic acid may be utilized as potential depigmenting agents by modulation of tyrosinase activity in melanocytes.
Figures and Tables
Fig. 1
DPPH radical scavenging activity (A) and tyrosinase inhibition activity (B) of VARM. Ascorbic acid (C) and Kojic acid (D) are used as positive controls for DPPH radical scavenging activity and tyrosinase inhibition activity, respectively.
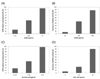
Fig. 2
Effect of VARM on B16F10 cell viability (A) and α-MSH induced melanogenesis (B). Arbutin is used as a positive control. Values are expressed as mean ± SD (n = 3). *, ** Significantly different from the vehicle treated control and α-MSH treated control, respectively (P < 0.05).
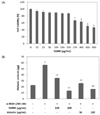
Fig. 3
Scheme of the preparation and isolation of VARM, its solvent fractions, and active compounds originated from the C fraction of VARM
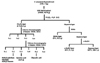
Fig. 4
Effect of VARM and its solvent fractions on B16F10 cell viability (A) and α-MSH induced melanogenesis (B). M, VARM; C, CH2Cl2 fraction; E, EtOAc fraction; B, BuOH fraction; W, H2O fraction. Arbutin is used as a positive control. *, **Significantly different from the vehicle treated control and α-MSH treated control, respectively (P < 0.05).
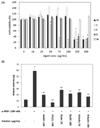
Fig. 5
Effect of VARM and its solvent fractions on cellular tyrosinase activity (A) and tyrosinase protein expression (B). M, VARM; C, CH2Cl2 fraction; E, EtOAc fraction; B, BuOH fraction; A, arbutin used as a positive control. *, **Significantly different from the vehicle treated control and α-MSH treated control, respectively (P < 0.05).
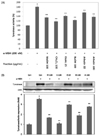
References
1. Yamaguchi Y, Brenner M, Hearing VJ. The regulation of skin pigmentation. J Biol Chem. 2007; 282:27557–27561.


2. Slominski A, Tobin DJ, Shibahara S, Wortsman J. Melanin pigmentation in mammalian skin and its hormonal regulation. Physiol Rev. 2004; 84:1155–1228.


3. Briganti S, Camera E, Picardo M. Chemical and instrumental approaches to treat hyperpigmentation. Pigment Cell Res. 2003; 16:101–110.


4. Costin GE, Hearing VJ. Human skin pigmentation: melanocytes modulate skin color in response to stress. FASEB J. 2007; 21:976–994.


5. Kondo T, Hearing VJ. Update on the regulation of mammalian melanocyte function and skin pigmentation. Expert Rev Dermatol. 2011; 6:97–108.


6. Cheli Y, Luciani F, Khaled M, Beuret L, Bille K, Gounon P, Ortonne JP, Bertolotto C, Ballotti R. αMSH and cyclic AMP elevating agents control melanosome pH through a protein kinase A-independent mechanism. J Biol Chem. 2009; 284:18699–18706.


7. Passeron T, Namiki T, Passeron HJ, Le Pape E, Hearing VJ. Forskolin protects keratinocytes from UVB-induced apoptosis and increases DNA repair independent of its effects on melanogenesis. J Invest Dermatol. 2009; 129:162–166.


8. Lee J, Jung E, Park J, Jung K, Park E, Kim J, Hong S, Park J, Park S, Lee S, Park D. Glycyrrhizin induces melanogenesis by elevating a cAMP level in b16 melanoma cells. J Invest Dermatol. 2005; 124:405–411.


9. Gibbs S, Murli S, De Boer G, Mulder A, Mommaas AM, Ponec M. Melanosome capping of keratinocytes in pigmented reconstructed epidermis--effect of ultraviolet radiation and 3-isobutyl-1-methylxanthine on melanogenesis. Pigment Cell Res. 2000; 13:458–466.


10. Agar N, Young AR. Melanogenesis: a photoprotective response to DNA damage? Mutat Res. 2005; 571:121–132.


11. Wen KC, Chang CS, Chien YC, Wang HW, Wu WC, Wu CS, Chiang HM. Tyrosol and its analogues inhibit alpha-melanocyte-stimulating hormone induced melanogenesis. Int J Mol Sci. 2013; 14:23420–23440.


12. Lee JY, Choi HJ, Chung TW, Kim CH, Jeong HS, Ha KT. Caffeic acid phenethyl ester inhibits alpha-melanocyte stimulating hormone-induced melanin synthesis through suppressing transactivation activity of microphthalmia-associated transcription factor. J Nat Prod. 2013; 76:1399–1405.


13. Eves PC, MacNeil S, Haycock JW. α-Melanocyte stimulating hormone, inflammation and human melanoma. Peptides. 2006; 27:444–452.


14. Mastore M, Kohler L, Nappi AJ. Production and utilization of hydrogen peroxide associated with melanogenesis and tyrosinase-mediated oxidations of DOPA and dopamine. FEBS J. 2005; 272:2407–2415.


15. Chaubal VA, Nair SS, Ito S, Wakamatsu K, Mojamdar MV. γ-Glutamyl transpeptidase and its role in melanogenesis: redox reactions and regulation of tyrosinase. Pigment Cell Res. 2002; 15:420–425.


16. Ha do T, Kim H, Thuong PT, Ngoc TM, Lee I, Hung ND, Bae K. Antioxidant and lipoxygenase inhibitory activity of oligostilbenes from the leaf and stem of Vitis amurensis. J Ethnopharmacol. 2009; 125:304–309.


17. Weidner S, Powałka A, Karamać M, Amarowicz R. Extracts of phenolic compounds from seeds of three wild grapevines-comparison of their antioxidant activities and the content of phenolic compounds. Int J Mol Sci. 2012; 13:3444–3457.


18. Wang JN, Chen YJ, Hano Y, Nomura T, Tan RX. Antioxidant activity of polyphenols from seeds of Vitis amurensis in vitro. Acta Pharmacol Sin. 2000; 21:633–636.
19. Jang MH, Piao XL, Kim JM, Kwon SW, Park JH. Inhibition of cholinesterase and amyloid-beta aggregation by resveratrol oligomers from Vitis amurensis. Phytother Res. 2008; 22:544–549.


20. Kim JY, Jeong HY, Lee HK, Kim S, Hwang BY, Bae K, Seong YH. Neuroprotection of the leaf and stem of Vitis amurensis and their active compounds against ischemic brain damage in rats and excitotoxicity in cultured neurons. Phytomedicine. 2012; 19:150–159.


21. Jeong HY, Kim JY, Lee HK, Ha do T, Song KS, Bae K, Seong YH. Leaf and stem of Vitis amurensis and its active components protect against amyloid beta protein (25-35)-induced neurotoxicity. Arch Pharm Res. 2010; 33:1655–1664.


22. Lee EO, Lee HJ, Hwang HS, Ahn KS, Chae C, Kang KS, Lu J, Kim SH. Potent inhibition of Lewis lung cancer growth by heyneanol A from the roots of Vitis amurensis through apoptotic and anti-angiogenic activities. Carcinogenesis. 2006; 27:2059–2069.


23. Kim JA, Kim MR, Kim O, Phuong NT, Yun J, Oh WK, Bae K, Kang KW. Amurensin G inhibits angiogenesis and tumor growth of tamoxifen-resistant breast cancer via Pin1 inhibition. Food Chem Toxicol. 2012; 50:3625–3634.


24. Yim N, Ha do T, Trung TN, Kim JP, Lee S, Na M, Jung H, Kim HS, Kim YH, Bae K. The antimicrobial activity of compounds from the leaf and stem of Vitis amurensis against two oral pathogens. Bioorg Med Chem Lett. 2010; 20:1165–1168.


25. Shin TY, Kim SH. Meoruh wine suppresses mast cell-mediated allergic inflammation. Immunopharmacol Immunotoxicol. 2011; 33:271–278.


26. Kim SH, Kwon TK, Shin TY. Antiallergic effects of Vitis amurensis on mast cell-mediated allergy model. Exp Biol Med (Maywood). 2008; 233:192–199.


27. Zhu L, Zhang Y, Lu J. Phenolic contents and compositions in skins of red wine grape cultivars among various genetic backgrounds and originations. Int J Mol Sci. 2012; 13:3492–3510.


28. Bak MJ, Jun M, Jeong WS. Procyanidins from wild grape (Vitis amurensis) seeds regulate ARE-mediated enzyme expression via Nrf2 coupled with p38 and PI3K/Akt pathway in HepG2 cells. Int J Mol Sci. 2012; 13:801–818.


29. Huang KS, Lin M. Oligostilbenes from the roots of Vitis amurensis. J Asian Nat Prod Res. 1999; 2:21–28.
30. Oh WK, Cho KB, Hien TT, Kim TH, Kim HS, Dao TT, Han HK, Kwon SM, Ahn SG, Yoon JH, Kim TH, Kim YG, Kang KW. Amurensin G, a potent natural SIRT1 inhibitor, rescues doxorubicin responsiveness via down-regulation of multidrug resistance 1. Mol Pharmacol. 2010; 78:855–864.


31. Lee EO, Kwon BM, Song GY, Chae CH, Kim HM, Shim IS, Ahn KS, Kim SH. Heyneanol A induces apoptosis via cytochrome c release and caspase activation in human leukemic U937 cells. Life Sci. 2004; 74:2313–2326.


32. Huang KS, Lin M, Cheng GF. Anti-inflammatory tetramers of resveratrol from the roots of Vitis amurensis and the conformations of the seven-membered ring in some oligostilbenes. Phytochemistry. 2001; 58:357–362.


33. Kedare SB, Singh RP. Genesis and development of DPPH method of antioxidant assay. J Food Sci Technol. 2011; 48:412–422.


34. Piao LZ, Park HR, Park YK, Lee SK, Park JH, Park MK. Mushroom tyrosinase inhibition activity of some chromones. Chem Pharm Bull (Tokyo). 2002; 50:309–311.


35. Ngamwongsatit P, Banada PP, Panbangred W, Bhunia AK. WST-1-based cell cytotoxicity assay as a substitute for MTT-based assay for rapid detection of toxigenic Bacillus species using CHO cell line. J Microbiol Methods. 2008; 73:211–215.


36. Chung KW, Park YJ, Choi YJ, Park MH, Ha YM, Uehara Y, Yoon JH, Chun P, Moon HR, Chung HY. Evaluation of in vitro and in vivo anti-melanogenic activity of a newly synthesized strong tyrosinase inhibitor (E)-3-(2,4 dihydroxybenzylidene)pyrrolidine-2,5-dione (3-DBP). Biochim Biophys Acta. 2012; 1820:962–969.


37. Schwahn DJ, Xu W, Herrin AB, Bales ES, Medrano EE. Tyrosine levels regulate the melanogenic response to alpha-melanocyte-stimulating hormone in human melanocytes: implications for pigmentation and proliferation. Pigment Cell Res. 2001; 14:32–39.


38. Park HY, Wu C, Yaar M, Stachur CM, Kosmadaki M, Gilchrest BA. Role of BMP-4 and its signaling pathways in cultured human melanocytes. Int J Cell Biol. 2009; 2009:750482.


39. Ding HY, Lin HC, Chang TS. Tyrosinase inhibitors isolated from the roots of Paeonia suffruticosa. J Cosmet Sci. 2009; 60:347–352.
40. Zi SX, Ma HJ, Li Y, Liu W, Yang QQ, Zhao G, Lian S. Oligomeric proanthocyanidins from grape seeds effectively inhibit ultraviolet-induced melanogenesis of human melanocytes in vitro. Int J Mol Med. 2009; 23:197–204.


41. Tan Y, Yu R, Pezzuto JM. Betulinic acid-induced programmed cell death in human melanoma cells involves mitogen-activated protein kinase activation. Clin Cancer Res. 2003; 9:2866–2875.
42. Chowdhury AR, Mandal S, Mittra B, Sharma S, Mukhopadhyay S, Majumder HK. Betulinic acid, a potent inhibitor of eukaryotic topoisomerase I: identification of the inhibitory step, the major functional group responsible and development of more potent derivatives. Med Sci Monit. 2002; 8:BR254–BR265.
43. Zuco V, Supino R, Righetti SC, Cleris L, Marchesi E, Gambacorti-Passerini C, Formelli F. Selective cytotoxicity of betulinic acid on tumor cell lines, but not on normal cells. Cancer Lett. 2002; 175:17–25.

