Abstract
BACKGROUND/OBJECTIVES
Rubus Coreanus Miquel (RCM), used as a traditional Korean medicine, reduces chronic inflammatory diseases such as cancer and rheumatoid arthritis. However, its mechanism has not been elucidated. In this study, we examine the anti-inflammatory effects of RCM and their possible mechanisms using RAW 264.7 cells.
MATERIALS/METHODS
Unripe RCM ethanol extract (UE), unripe RCM water extract (UH), ripe RCM ethanol extract (RE), and ripe RCM water extract (RH) were prepared. Inflammatory response was induced with LPS treatment, and expression of pro-inflammatory mediators (iNOS, COX-2, TNF-α, IL-1β, and IL-6) and NO and PGE2 productions were assessed. To determine the anti-inflammatory mechanism of RCM, we measured NF-κB and MAPK activities.
RESULTS
UE and UH treatment significantly reduced NF-κB activation and JNK and p38 phosphorylation and reduced transcriptional activities decreased iNOS, COX-2, and pro-inflammatory cytokines expressions, and NO and PGE2 productions. RE and RH treatments reduced IL-1β and IL-6 expressions through suppressions of JNK and p38 phosphorylation.
CONCLUSIONS
In this study, we showed that RCM had anti-inflammatory effects by suppression of pro-inflammatory mediator expressions. Especially, unripe RCM showed strong anti-inflammatory effects through suppression of NF-κB and MAPK activation. These findings suggest that unripe RCM might be used as a potential functional material to reduce chronic inflammatory responses.
Inflammation is a protective response to pathogens such as bacteria, viruses, and parasites [1]. In inflammatory responses, macrophages are known to be one of the major immune cells involved [1,2], which are activated by several stimuli such as cytokines and viruses, and secrete pro-inflammatory mediators including nitric oxide (NO), prostaglandin E2 (PGE2), tumor necrosis factor-α (TNF-α), interleukin-1β (IL-1β), and IL-6 [1,2]. These inflammatory mediators are critically associated with pain, fever, edema, and recruiting additional immune cells to the site of inflammation [1]. However, overproduction of inflammatory mediators is associated with tissue damage [3]. Moreover, several studies have been reported that chronic inflammatory response could increase risk of chronic inflammatory diseases (CIDs) such as cancer, diabetes, and cardiovascular disease [4,5,6]. Thus, inhibition of pro-inflammatory mediator release could be beneficial in attenuating the inflammatory response in chronic diseases.
Expression of pro-inflammatory mediators, including inducible NO synthase (iNOS), cyclooxygenase-2 (COX-2), and pro-inflammatory cytokines, is regulated by activation of nuclear factor-κ B (NF-κB) and mitogen-activated protein kinases (MAPK) [2]. NF-κB exists as a heterodimer consisting of p65 and p50 subunits which are associated with inhibitors of κB (IκB) in the cytoplasm as an inactive form [2,7,8]. When macrophages are exposed to stimuli such as lipopolysaccaride (LPS), IκB is phosphorylated by IκB kinase (IKK) and NF-κB is separated from the phosphorylated IκB [2,3,7]. Separated NF-κB translocates to the nucleus and induces transcription of a variety of target genes such as iNOS, COX-2, IL-1β, and IL-6 [3,9,10]. Therefore, activation of NF-κB leads to inflammatory responses. LPS also stimulates MAPK pathways which increase pro-inflammatory cytokines expression through activator protein-1 (AP-1) activation [11,12]. MAPK activates AP-1 by phosphorylation then activated AP-1 binds to DNA to induce transcription of pro-inflammatory cytokines [12].
Rubus coreanus Miquel (RCM) has been used as one of the traditional Korean medicine for treatment of prostatism, diabetes mellitus, impotence, spermatorrhea, enuresis, and asthma [13]. Recent studies have shown that RCM reduces prostate tumor growth, kidney and stomach diseases, and rheumatoid arthritis because of high amounts of polyphenols [14,15,16,17]. Several studies reported that RCM contains high amounts of phenolic compounds including ellagic acid, gallic acid, cinnamic acid, protocatechuic acid, sangiin H-4, sanguiin H-6, 23-hydroxytormentic acid, and nigaichgoside F1 [18,19,20,21]. Other studies have shown that dietary polyphenols reduce inflammatory responses by reducing oxidative stress [22,23,24]. High polyphenol containing food such as strawberries, cherries, grapes, wine, or tea, decreased PGE2 and NO production by suppression of COX-2 and iNOS expressions through reduction of oxidative stress [22,23,24]. Ripe and unripe RCM also has been shown to reduce inflammatory cytokines production such as TNF-α, and IL-6 in mast cells and macrophages [16,25]. In addition, ellagitannins in ripe Rubus berries decreased gastric inflammation through suppression of NF-κB pathway [26]. RCM has been used as a medicine or food depending on its maturation. Unripe RCM has been used as a traditional remedy for some chronic diseases but ripe RCM has been taken as a fruit. Both RCM has been shown to have anti-carcinogenic, anti-diabetic, and anti-inflammatory effects. However, few studies have compared their anti-inflammatory effects depending on their maturation and none of them elucidated its mechanism. Therefore, in this study, we determine the effect of RCM on inflammatory responses and its possible mechanism depending on its maturation.
Ripe and unripe fruits of RCM were collected from May to June of 2010 in the Gokseong area of South Korea and freeze-dried. Four different extracts were prepared from the fruits: i) 50% ethanol extracts of unripe fruit (UE), ii) aqueous extracts of unripe fruit (UH), iii) 50% ethanol extracts of ripe fruits (RE), and iv) aqueous extracts of ripe fruit (RH). Freeze-dried unripe or ripe fruits were pulverized and extracted in solutions of 30 grams of berries/L in distilled water or 50% ethanol by heating at 45℃ for 1.5 h. Supernatants were collected and and frozen dried with a lyophilizer. The obtained RCM extracts were dissolved in dimethyl sulfoxide (DMSO) and kept at -20℃ until it was used.
The murine macrophage cell line RAW 264.7 was purchased from the Korea Cell Line Bank (Seoul, Korea). Cells were cultured in DMEM containing 10% fetal bovine serum (v/v), 5% penicillin (100 U/ml), and streptomycin (100 µg/ml; Gibco TM Invitrogen, Grand Island, NY, USA) at 37℃ in a humidified atmosphere of 5% CO2. RCM (400 µg/ml) was added on plated cells for 24 h, and then stimulated with 1 µg/ml of LPS (Sigma-Aldrich, St. Louis, MO, USA) for the indicated time.
Cells were treated with increasing doses of RCM extracts for 24 h then stimulated with 1 µg/ml of LPS for 18h. Cell survival was determined by adding 500µg/ml of 3-(4,5-Dimethylthiazol-2-yl)-2,5-diphenyltetrazolium bromide (MTT) to each well and incubated for another 2 h at 37℃. After removal of medium, cells were lysed with isopropanol. The absorbance of the colored solution was quantified by measuring at 450nm with a microplate reader (Molecular Devices, Spectra MAX 340).
RAW 264.7 cells were pretreated with 400 µg/ml of RCM extract for 24h then stimulated with LPS (1 µg/ml). After 18h, NO and PGE2 levels in the culture media were determined using Nitrate/Nitrite Colorimetric Assay kits and PGE2 EIA kits (Cayman Chemical, Ann Arbor, MI, USA), respectively.
RAW 264.7 cells were incubated with RCM extracts for 24 h then stimulated with LPS for 18 h. Cell-free supernatants were subsequently employed in pro-inflammatory cytokine assays using mouse enzyme-linked immunosorbent assay (ELISA) kits (R&D systems, Minneapolis, MN, USA), following protocol of the manufacturer.
RAW 264.7 cells were incubated with RCM extracts for 24 h then induced by LPS (1 µg/ml) for 4 h. Total RNA from LPS-induced RAW 264.7 cells was prepared using RNeasy mini kits (QIAGEN, Valencia, CA, USA) according to manufacturer's protocol and stored at -80℃ before use. Total RNA was reverse-transcribed with Superscript First strand synthesis systems kits (Invigrogen, Carlsbad, CA, USA) to obtain cDNA. All PCR analyses using a thermal cycler (Bioer Technology Co., LTD, Hangzhou, China) were subsequently carried out in a 20 µl volume containing 10 µl of SYBR Green, 100 pmol of 5' and 3' primers, and cDNA. PCR primers used in this study are the following: iNOS: 5'-AGTGGTGTTCTTTGCTTC-3' (forward) and 5'-GCTTGCCTT ATACTGGTC-3' (reverse); COX-2: 5'-GGTCTGGTGCCTGGTCTG-3' (forward) and 5'-CTCTCCTATGAGTATGAGTCTGC-3' (reverse); TNF-α: 5'-ACGGCATGGATCTCAAAGAC-3' (forward) and 5'-AGATAG CAAATCGGCTGACG-3' (reverse); IL-1β : 5'-AATCTATACCTGTCCT GTGTAATG-3' (forward) and 5'-GCTTGTGCTCTGCTTGTG-3' (reverse); IL-6: 5'-CTTCCATCCAGTTGCCTTCTT-3' (forward) and 5'-ACG ATTTCCCAGAGAACATGT-3' (reverse); GAPDH : 5'-CCATCACCATC TTCCAGGAGCG-3' (forward) and 5'-AGAGATGATGACCCTTTTG GC-3' (reverse). After amplification, a portion of the PCR products were electrophoresed on 1% agarose gel and visualized under UV after ethidium bromide staining.
After RCM treatments, cells were stimulated with LPS and lysated in RIPA lysis buffer containing cocktail protease inhibitors and phosphatase inhibitors (Sigma-Aldrich, St. Louis, USA). Immunoblot assay was carried out as described previously [27] with antibodies against iNOS, COX-2, p-IκB, ERK 1/2, p-ERK 1/2, p38, p-p38, JNK, p-JNK, and β-actin from Santa Cruz Biotechnology (Santa Cruz, CA, USA).
RAW 264.7 cells were harvested after 24h RCM treatment and subsequent LPS stimulation for 20 min. Nuclear proteins were extracted using a Nuclear Extraction kit (Cayman, Ann Arbor, MI, USA), and NF-κB transcriptional activity was measured using an NF-κB transcriptional factor assay kit (Cayman, Ann Arbor, MI, USA). Two micrograms of extracted nuclear proteins were incubated overnight at 4℃ and p65 primary antibody was incubated. After wash, secondary antibodies were incubated and developed with transcription factor developing solution. The absorbance of the colored solution was quantified by measuring at 450nm with a microplate reader (Molecular Devices, Spectra MAX 340).
All data are presented as means ± standard error (SE) from at least three independent experiments. Data were statistically analyzed using one-way analysis of variance (ANOVA), followed by Duncan's multiple range test using SPSS Statistics V.17.0. A value of P < 0.05 was considered statistically significant.
To avoid any cytotoxic effect caused by RCM, we investigated the effects of RCM extracts on cell viability in RAW 264.7 using MTT assays (Fig. 1). RCM extract had no effect on cell viability except for UE, which slightly increased cell viability at 200 µg/ml. RAW 264.7 were treated with 400 µg/ml of RCM for subsequent analyses.
To investigate the effects of RCM on LPS-induced NO production and iNOS expression, cells were treated with 400 µg/ml of RCM extract for 24 h then stimulated with 1 µg/ml of LPS for 18 h. As shown in Fig. 2A, RCM treatment significantly reduced NO production compared to LPS-stimulated control. In addition, RCM pretreatments significantly reduced mRNA as well as protein expression of iNOS compared to LPS-stimulated control (Fig. 2B and 2C).
We attempted to determine whether RCM extracts modulated LPS-induced productions of PGE2 and COX-2 expression. Unripe RCM treatment significantly reduced PGE2 production by about 50% compared to LPS-stimulated control, but ripe RCM treatment did not (Fig. 3A). Moreover, unripe RCM significantly reduced COX-2 mRNA and protein expressions (Fig. 3B and 3C). However, ripe RCM did not modulate COX-2 expressions (Fig. 3B and 3C).
Next, we determine the effect of RCM on pro-inflammatory cytokine expression (Fig. 4). In TNF-α production, only UE treatment significantly decreased mRNA (Fig. 4A) and protein expression compared to LPS-stimulated control (Fig. 4B). However, UH, RE, and RH treatment had no effects on TNF-α production. RCM extract significantly decreased IL-1β and IL-6 expressions compared to LPS-stimulated control (Fig. 4C, 4D, 4E, and 4F). These results suggest that the inhibitory effects of RCM on the LPS-induced production of pro-inflammatory cytokines are regulated at the transcriptional level.
Since inflammatory mediator expressions are activated by NF-κB and MAP kinase activities, we tested whether these activities are modulated by RCM extract (Fig. 5). RAW 264.7 cells were treated with RCM for 24h before 20min of LPS stimulation. LPS-induced NF-κB activities were significantly decreased when cells were pretreated with unripe RCM (Fig. 5A). Especially, UE treatment reduced NF-κB activity by about 50% compared to LPS-stimulated control. However, ripe RCM treatment had no such effect. Furthermore, considering LPS-induced NF-κB activation requires degradation of IκB, we investigated cytosolic phosphorylated IκB protein expression in RAW 264.7 cells (Fig. 5B). Cytosolic p-IκB proteins were less detected in UE- and UH-treated macrophage cells, but RE and RH treatment had no effect on IκB phosphorylation. Since pro-inflammatory cytokines are transcriptionally regulated by MAP kinases activities, we also measured 3 different MAP kinases, extracellular signal-regulated kinase 1/2 (ERK 1/2), MAPK p38, and c-Jun N-terminal kinase (JNK), phosphorylation levels (Fig. 5C). Unripe and ripe RCM significantly reduced JNK and p38 phosphorylation but had no effects on ERK1/2 activation. Unripe and ripe RCM treatment showed about 20~25% reduction of JNK phosphorylation and 60% reduction of p38 phosphorylation. These data indicated that unripe RCM reduced pro-inflammatory mediator expression through suppression of NF-κB and MAP kinase activities but ripen RCM only decreased two MAP kinases, JNK and p38, phosphorylation.
Since RCM has been shown to have high amounts of polyphenols and has been used as a traditional remedy for some CIDs, we determined the anti-inflammatory effects of RCM and their possible mechanisms using LPS-induced RAW 264.7 cells. In this study, we showed that unripe RCM (UE and UH) significantly reduced pro-inflammatory mediator expressions through suppression of NF-κB activity and MAP kinase activity.
We demonstrated that unripe RCM (UE and UH) showed stronger reduction of pro-inflammatory mediator production compared to ripe RCM (RE and RH). Several studies have reported that during maturation of RCM, amounts of polyphenols decreased while sugar increased [21,28,29]. We previously have reported that unripe RCM contained more of cinnamic acid (16 times), ferulic acid (6 times), epicatechine (6 times), protocatechuic acid (4 times), gallic acid (3 times), and vanillic acid (2.5 times) compared to ripe RCM [21]. Moreover, active compounds of unripe RCM, nigaichigoside F1 was present at approximately 2.5% (w/dried w). However, ripe RCM contained more of anthocyanins (4.1g/100g of dried weight) and cyaniding compared to unripe RCM [21]. Polyphenols are known as excellent anti-oxidants and have been shown to have anti-inflammatory effects [19,28,30]. Therefore, unripe RCM, containing higher amounts of polyphenolic compounds, showed stronger anti-inflammatory response than ripe RCM.
In this study, we also showed that unripe RCM reduced pro-inflammatory mediator expressions through suppression of NF-κB activity, and MAP kinases, JNK and p38, activities. NF-κB, a transcriptional factor, regulates expression of pro-inflammatory mediators including iNOS, COX-2, and proinflammatory cytokines, and NF-κB activity is regulated by phosphorylation of IκB [2]. Unripe RCM significantly reduced phosphorylation of IκB, which blocked NF-κB translocation into nucleus and reduced pro-inflammatory mediators and cytokines expressions. In addition, unripe RCM significantly reduced MAP kinase phosphorylation. Several studies have shown that pro-inflammatory mediator expressions are regulated by phosphorylation of MAP kinase families such as ERK 1/2, p38, and JNK [31]. Phosphorylated MAP kinase activates AP-1 consisting of c-Jun and c-Fos, and then activates AP-1, a transcriptional factor that regulates inflammatory responses via modulation of expression of inflammatory mediator [31,32,33]. Other studies also showed that RCM inhibited inflammatory response through reduction of NF-κB and MAPK activity in mast cells and gastric cells [25,26]. Shin et al. reported that ripe RCM attenuated allergic inflammation such as histamine release, passive cutaneous anaphylaxis, and cytokine productions through inhibition of NF-κB and MAPK activities [25]. Moreover, Agli et al. showed that ellagitannins in Rubus berries was the anti-inflammatory compound and ellagitannins suppressed gastric inflammation through decrease of NF-κB translocation [26]. In this study, we showed that unripe RCM reduced pro-inflammatory mediator expression through suppression of NF-κB activity as well as MAP kinases, JNK and p38, activities. However, even though ripe RCM (RE and RH) reduced IL-1β and IL-6 production, it only modulated JNK and p38 phosphorylation but not NF-κB activities in macrophages. These results suggest that effects of anti-inflammatory response of RCM are differently modulated depending on cell lines or tissues.
Unripe RCM significantly reduced NO and PGE2 production, and iNOS and COX-2 mRNA and protein expressions. NO is a high reactive molecule that is synthesized from L-arginine in a reaction catalyzed by an NOS. Three different NOS isoforms have been identified [34], among which iNOS is known to be the most important in regulation of inflammatory responses [34,35]. Activated macrophages generate large amounts of NO by iNOS induction until the enzyme is degraded [35,36,37]. NO generated in the body has cytotoxicity activity and is involved in the induction biological response by activation of NO-sensitive enzymes such as guanylate cyclase [35,36,37]. However, overproduction of NO could be one of the causes of inflammatory disorder by suppressing growth of lymphocytes and damaging other normal cells and tissues [34,35,37]. PGE2 is also an inflammatory mediator and is produced by COXs. COX exists in two forms; COX-1 is expressed in almost all tissues and associated with blood flow, whereas COX-2 is dramatically up-regulated during inflammation and induces inflammatory responses such as fever and vasodilatation by generating PGE2 [38,39]. COX-2 converts arachidonic acid to prostanoids such as prostaglandins, prostacyclins and thromboxanes [40], and over production of PGE2 has been implicated in the pathology of several CIDs [41]. Since iNOS and COX-2 are key mediators of inflammation, unripe RCM could be used as an anti-inflammatory reagent.
Ethanol extract of unripe RCM significantly reduced proinflammatory cytokine expressions. Other RCM extracts significantly decreased IL-1β and IL-6 expressions but not TNF-α expression. TNF-α, IL-1β and IL-6 are key cytokines for inflammation induction in macrophages [42]. These pro-inflammatory cytokines are involved in inflammatory responses such as generation of fever, induction of COX-2 expression, increase of PGE2 production, and promoting of immune cell differentiation [43]. However, sustained production of pro-inflammatory cytokines recruit immune cells to the site of inflammation and amplify the inflammatory state, thereby increasing risk of CIDs [3]. In this study, we found that LPS highly stimulated pro-inflammatory cytokines but RCM extracts selectively inhibited cytokine expression. These results suggest that RCM, especially unripe RCM, possesses useful anti-inflammatory activity through inhibition of pro-inflammatory cytokine production.
In this study, we showed that RCM can reduce expression and production of several inflammatory mediators, and unripe RCM (UE and UH) more effectively inhibits inflammatory responses compared to ripe RCM (RE and RH) via suppression of NF-κB activation and MAP kinases phosphorylation. Therefore, these results suggest that unripe RCM might be used for treatment of CIDs. Since sulfasalazine or glucocorticoids are widely used for treatment of CIDs, but are known to have side-effects such as fatigue, stomach pain, diarrhea, and osteoporosis [44,45,46]. Thus, many studies have been focused on natural products to safely suppress inflammation via suppression of NF-κB activation and/or MAP kinases activities [47]. Therefore, RCM which has been used as a traditional remedy, might be used as a potential functional material for prevention and treatment of CIDs because of its anti-inflammatory effects.
Figures and Tables
Fig. 1
Effects of Rubus coreanus Miquel (RCM) extracts on cell viability in RAW 264.7. Cells were treated with UE, UH, RE and RH (0, 12.5, 25, 50, 100, 200 and 400 µg/ml) for 24 h then percentage of cell viability was measured by MTT assay. Values not sharing the same letter were significantly different (P < 0.05). (UE: unripe Rubus coreanus Miquel ethanol extract, UH: unripe Rubus coreanus Miquel water extract, RE: ripe Rubus coreanus Miquel ethanol extract, and RH: ripe Rubus coreanus Miquel water extract)
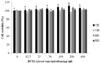
Fig. 2
Effects of RCM extracts on NO production and iNOS expression in LPS induced RAW 264.7. RAW 264.7 cells were treated with UE, UH, RE and RH (400 µg/ml) for 24 h then stimulated with LPS (1 µg/ml) for 4 h (B) or 18 h (A, C). Relative NO production, iNOS mRNA expression and iNOS protein expression were measured by nitrite/nitrate kit (A), PCR (B), and western blot (C). Values not sharing the same letter were significantly different (P < 0.05). (UE: unripe Rubus coreanus Miquel ethanol extract, UH: unripe Rubus coreanus Miquel water extract, RE: ripe Rubus coreanus Miquel ethanol extract, and RH: ripe Rubus coreanus Miquel water extract)
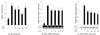
Fig. 3
Effects of RCM extracts on PGE2 production and COX-2 expression in LPS induced RAW 264.7. RAW 264.7 cells were treated with UE, UH, RE and RH (400 µg/ml) for 24 h then stimulated with LPS (1 µg/ml) for 4 h (B) or 18 h (A, C). Relative PGE2 production, COX-2 mRNA expression and COX-2 protein expression were measured by PGE2 EIA kit (A), PCR (B), and western blot (C). Values not sharing the same letter were significantly different (P < 0.05). (UE: unripe Rubus coreanus Miquel ethanol extract, UH: unripe Rubus coreanus Miquel water extract, RE: ripe Rubus coreanus Miquel ethanol extract, and RH: ripe Rubus coreanus Miquel water extract)

Fig. 4
Effect of RCM on TNF-α (A and B), IL-1β (C and D), and IL-6 (E and F) mRNA and protein expression. Cells were pretreated with 400 µg/ml concentration of RCM for 24 h then stimulated with LPS (1 µg/ml) for 4 or 16 h. Total RNA and protein lysates were subjected to semi-quantitative PCR or Western blot analysis. The values are expressed as the mean ± S.D. of three individual experiments. Values not sharing the same letter were significantly different (P < 0.05). (UE: unripe Rubus coreanus Miquel ethanol extract, UH: unripe Rubus coreanus Miquel water extract, RE: ripe Rubus coreanus Miquel ethanol extract, and RH: ripe Rubus coreanus Miquel water extract)
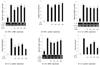
Fig. 5
Effects of RCM extracts on NF-κB activity (A), IκB phosphorylation (B) and MAP kinase activity (C) in LPS induced RAW 264.7. Cells were treated with UE, UH, RE and RH (400 µg/ml) for 24 h then stimulated with LPS (1 µg/ml) for 20 min (A, C) or 15 min (B). NF-κB activity and relative IκB phospholylation were measured by NF-κB (p65) transcription factor assay kit (A) and western blot (B) and MAP kinase activity was measured by western blot (C). Values not sharing the same letter were significantly different (P < 0.05). (UE: unripe Rubus coreanus Miquel ethanol extract, UH: unripe Rubus coreanus Miquel water extract, RE: ripe Rubus coreanus Miquel ethanol extract, and RH: ripe Rubus coreanus Miquel water extract)
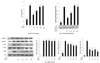
Notes
This study was carried out with the support of the Research Program for Agricultural Science & Technology Development (Project No. PJ006387012011), the National Academy of Agricultural Science, and the Rural Development Administration, Republic of Korea and supported by the Chung-Ang University Excellent Student Scholarship.
References
1. Laroux FS. Mechanisms of inflammation: the good, the bad and the ugly. Front Biosci. 2004; 9:3156–3162.


2. Barnes PJ, Karin M. Nuclear factor-kappaB: a pivotal transcription factor in chronic inflammatory diseases. N Engl J Med. 1997; 336:1066–1071.


3. Blackwell TS, Christman JW. The role of nuclear factor-kappa B in cytokine gene regulation. Am J Respir Cell Mol Biol. 1997; 17:3–9.


4. Ben-Neriah Y, Karin M. Inflammation meets cancer, with NF-kappaB as the matchmaker. Nat Immunol. 2011; 12:715–723.


5. Schmidt MI, Duncan BB, Sharrett AR, Lindberg G, Savage PJ, Offenbacher S, Azambuja MI, Tracy RP, Heiss G. Markers of inflammation and prediction of diabetes mellitus in adults (Atherosclerosis Risk in Communities study): a cohort study. Lancet. 1999; 353:1649–1652.


6. Pearson TA, Mensah GA, Alexander RW, Anderson JL, Cannon RO 3rd, Criqui M, Fadl YY, Fortmann SP, Hong Y, Myers GL, Rifai N, Smith SC Jr, Taubert K, Tracy RP, Vinicor F. Centers for Disease Control and Prevention. American Heart Association. Markers of inflammation and cardiovascular disease: application to clinical and public health practice: A statement for healthcare professionals from the Centers for Disease Control and Prevention and the American Heart Association. Circulation. 2003; 107:499–511.
7. Tak PP, Firestein GS. NF-kappaB: a key role in inflammatory diseases. J Clin Invest. 2001; 107:7–11.
8. Lee JI, Burckart GJ. Nuclear factor kappa B: important transcription factor and therapeutic target. J Clin Pharmacol. 1998; 38:981–993.


9. Brockman JA, Scherer DC, McKinsey TA, Hall SM, Qi X, Lee WY, Ballard DW. Coupling of a signal response domain in I kappa B alpha to multiple pathways for NF-kappa B activation. Mol Cell Biol. 1995; 15:2809–2818.


10. Siebenlist U, Franzoso G, Brown K. Structure, regulation and function of NF-kappa B. Annu Rev Cell Biol. 1994; 10:405–455.
11. Chen C, Chen YH, Lin WW. Involvement of p38 mitogen-activated protein kinase in lipopolysaccharide-induced iNOS and COX-2 expression in J774 macrophages. Immunology. 1999; 97:124–129.


12. Guha M, Mackman N. LPS induction of gene expression in human monocytes. Cell Signal. 2001; 13:85–94.


13. Kim EJ, Lee YJ, Shin HK, Park JH. Induction of apoptosis by the aqueous extract of Rubus coreanum in HT-29 human colon cancer cells. Nutrition. 2005; 21:1141–1148.


14. Ra JC, Lee HY, Choi MK, Park HG, Kang KS. Effect of decreasing body weight with plant extracts containing Rubi fructus. J Toxicol Public Health. 2004; 20:167–172.
15. Yang HM, Oh SM, Lim SS, Shin HK, Oh YS, Kim JK. Antiinflammatory activities of Rubus coreanus depend on the degree of fruit ripening. Phytother Res. 2008; 22:102–107.


16. Yang HM, Lim SS, Lee YS, Shin HK, Oh YS, Kim JK. Comparison of the anti-inflammatory effects of the extracts from Rubus coreanus and Rubus occidentalis. Korean J Food Sci Technol. 2007; 39:342–347.
17. Kim Y, Kim J, Lee SM, Lee HA, Park S, Kim Y, Kim JH. Chemopreventive effects of Rubus coreanus Miquel on prostate cancer. Biosci Biotechnol Biochem. 2012; 76:737–744.
18. Kim YH, Choi JH, Rim HK, Kang HJ, Chang SG, Park JH, Park HJ, Choi JW, Kim SD, Lee KT. 23-Hydroxytormentic acid and nigaichgoside f(1) isolated from Rubus coreanus attenuate cisplatin-induced cytotoxicity by reducing oxidative stress in renal epithelial LLC-PK(1) cells. Biol Pharm Bull. 2011; 34:906–911.


19. Lee J, Dossett M, Finn CE. Rubus fruit phenolic research: the good, the bad, and the confusing. Food Chem. 2012; 130:785–796.


20. Pang KC, Kim MS, Lee MW. Hydrolyzable tannins from the fruits of Rubus coreanum. Korean J Pharmacogn. 1996; 27:366–370.
21. Kim HS, Park SJ, Hyun SH, Yang SO, Lee J, Auh JH, Kim JH, Cho SM, Marriott PJ, Choi HK. Biochemical monitoring of black raspberry (Rubus coreanus Miquel) fruits according to maturation stage by 1H-NMR using multiple solvent systems. Food Res Int. 2011; 44:1977–1988.
22. Ellis CL, Edirisinghe I, Kappagoda T, Burton-Freeman B. Attenuation of meal-induced inflammatory and thrombotic responses in overweight men and women after 6-week daily strawberry (Fragaria) intake. A randomized placebo-controlled trial. J Atheroscler Thromb. 2011; 18:318–327.


23. Terra X, Montagut G, Bustos M, Llopiz N, Ardèvol A, Bladé C, Fernández-Larrea J, Pujadas G, Salvadó J, Arola L, Blay M. Grape-seed procyanidins prevent low-grade inflammation by modulating cytokine expression in rats fed a high-fat diet. J Nutr Biochem. 2009; 20:210–218.


24. Seymour EM, Lewis SK, Urcuyo-Llanes DE, Tanone II, Kirakosyan A, Kaufman PB, Bolling SF. Regular tart cherry intake alters abdominal adiposity, adipose gene transcription, and inflammation in obesity-prone rats fed a high fat diet. J Med Food. 2009; 12:935–942.


25. Kim HH, Choi PH, Yoo JS, Jeon H, Chae BS, Park JS, Kim SH, Shin TY. Ripe fruit of Rubus coreanus inhibits mast cell-mediated allergic inflammation. Int J Mol Med. 2012; 29:303–310.


26. Sangiovanni E, Vrhovsek U, Rossoni G, Colombo E, Brunelli C, Brembati L, Trivulzio S, Gasperotti M, Mattivi F, Bosisio E, Dell'Agli M. Ellagitannins from Rubus berries for the control of gastric inflammation: in vitro and in vivo studies. PLoS One. 2013; 8:e71762.


28. Kim JM, Shin M. Characteristics of Rubus coreanus Miq. Fruits at different ripening stages. Korean J Food Sci Technol. 2011; 43:341–347.


29. Wang SY, Lin HS. Antioxidant activity in fruits and leaves of blackberry, raspberry, and strawberry varies with cultivar and developmental stage. J Agric Food Chem. 2000; 48:140–146.


30. González R, Ballester I, López-Posadas R, Suárez MD, Zarzuelo A, Martínez-Augustin O, Sánchez de Medina F. Effects of flavonoids and other polyphenols on inflammation. Crit Rev Food Sci Nutr. 2011; 51:331–362.


31. Herlaar E, Brown Z. p38 MAPK signalling cascades in inflammatory disease. Mol Med Today. 1999; 5:439–447.


32. Fujioka S, Niu J, Schmidt C, Sclabas GM, Peng B, Uwagawa T, Li Z, Evans DB, Abbruzzese JL, Chiao PJ. NF-kappaB and AP-1 connection: mechanism of NF-kappaB-dependent regulation of AP-1 activity. Mol Cell Biol. 2004; 24:7806–7819.


33. Whitmarsh AJ, Davis RJ. Transcription factor AP-1 regulation by mitogen-activated protein kinase signal transduction pathways. J Mol Med (Berl). 1996; 74:589–607.


34. Korhonen R, Lahti A, Kankaanranta H, Moilanen E. Nitric oxide production and signaling in inflammation. Curr Drug Targets Inflamm Allergy. 2005; 4:471–479.


35. Nussler AK, Billiar TR. Inflammation, immunoregulation, and inducible nitric oxide synthase. J Leukoc Biol. 1993; 54:171–178.


36. Kleinert H, Pautz A, Linker K, Schwarz PM. Regulation of the expression of inducible nitric oxide synthase. Eur J Pharmacol. 2004; 500:255–266.


37. MacMicking J, Xie QW, Nathan C. Nitric oxide and macrophage function. Annu Rev Immunol. 1997; 15:323–350.


38. Murakami A, Ohigashi H. Targeting NOX, INOS and COX-2 in inflammatory cells: chemoprevention using food phytochemicals. Int J Cancer. 2007; 121:2357–2363.


39. Dubois RN, Abramson SB, Crofford L, Gupta RA, Simon LS, Van De Putte LB, Lipsky PE. Cyclooxygenase in biology and disease. FASEB J. 1998; 12:1063–1073.


40. Lin CC, Lee IT, Yang YL, Lee CW, Kou YR, Yang CM. Induction of COX-2/PGE(2)/IL-6 is crucial for cigarette smoke extract-induced airway inflammation: role of TLR4-dependent NADPH oxidase activation. Free Radic Biol Med. 2010; 48:240–254.


41. Willoughby DA, Moore AR, Colville-Nash PR. COX-1, COX-2, and COX-3 and the future treatment of chronic inflammatory disease. Lancet. 2000; 355:646–648.


42. Tao JY, Zheng GH, Zhao L, Wu JG, Zhang XY, Zhang SL, Huang ZJ, Xiong FL, Li CM. Anti-inflammatory effects of ethyl acetate fraction from Melilotus suaveolens Ledeb on LPS-stimulated RAW 264.7 cells. J Ethnopharmacol. 2009; 123:97–105.


43. Feghali CA, Wright TM. Cytokines in acute and chronic inflammation. Front Biosci. 1997; 2:d12–d26.


44. Weber CK, Liptay S, Wirth T, Adler G, Schmid RM. Suppression of NF-kappaB activity by sulfasalazine is mediated by direct inhibition of IkappaB kinases alpha and beta. Gastroenterology. 2000; 119:1209–1218.


45. Schäcke H, Döcke WD, Asadullah K. Mechanisms involved in the side effects of glucocorticoids. Pharmacol Ther. 2002; 96:23–43.

