Abstract
The rapid increase in the prevalence of metabolic syndrome, which is associated with a state of elevated systemic oxidative stress and inflammation, is expected to cause future increases in the prevalence of diabetes and cardiovascular diseases. Oxidation of polyunsaturated fatty acids and sugars produces reactive carbonyl species, which, due to their electrophilic nature, react with the nucleophilic sites of certain amino acids. This leads to formation of protein adducts such as advanced glycoxidation/lipoxidation end products (AGEs/ALEs), resulting in cellular dysfunction. Therefore, an effective reactive carbonyl species and AGEs/ALEs sequestering agent may be able to prevent such cellular dysfunction. There is accumulating evidence that histidine containing dipeptides such as carnosine (β-alanyl-L-histidine) and anserine (β-alanyl-methyl-L-histidine) detoxify cytotoxic reactive carbonyls by forming unreactive adducts and are able to reverse glycated protein. In this review, 1) reaction mechanism of oxidative stress and certain chronic diseases, 2) interrelation between oxidative stress and inflammation, 3) effective reactive carbonyl species and AGEs/ALEs sequestering actions of histidine-dipeptides and their metabolism, 4) effects of carnosinase encoding gene on the effectiveness of histidine-dipeptides, and 5) protective effects of histidine-dipeptides against progression of metabolic syndrome are discussed. Overall, this review highlights the potential beneficial effects of histidine-dipeptides against metabolic syndrome. Randomized controlled human studies may provide essential information regarding whether histidine-dipeptides attenuate metabolic syndrome in humans.
Metabolic syndrome is characterized by a group of metabolic risk factors (abdominal obesity, atherogenic dyslipidemia, elevated blood pressure, and insulin resistance/glucose intolerance) [1]. The joint interim statement of the International Diabetes Federation Task Force on Epidemiology and Prevention; National Heart, Lung, and Blood Institute; American Heart Association; World Heart Federation; International Atherosclerosis Society; and International Association for the Study of Obesity identifies metabolic syndrome as having three out of the five metabolic risk factors (Table 1) [1].
Metabolic syndrome is reported to be common globally, with increasing prevalence in the United States [2] and Korea [3]. In the United States, metabolic syndrome rates measured by the National Health and Nutrition Examination Survey 1999-2000 [2] and National Health Statistics Reports (2003-2006) [4] in the elderly (aged ≥ 60) were 46.4 and 52% in men and 56.0 and 54% in women, respectively. This increasing prevalence has also been observed in Korea, where it is mainly due to increases of abdominal obesity and dyslipidemia [3]. According to the Korea National Health and Nutrition Examination survey for 1998-2007, the age-adjusted prevalence of metabolic syndrome increased significantly from 24.9% in 1998 to 31.3% in 2007.
Although increased oxidative stress [5-7] and inflammatory cytokines [8] are general phenomena in metabolic syndrome, the mechanism of the interrelation between oxidative stress and inflammation is not well established. Nevertheless, the increase of metabolic syndrome is projected to lead to further increases in diabetes [9], as well as cardiovascular and renal diseases [10].
Elevated cytotoxic reactive carbonyl species, which are produced by the oxidation of polyunsaturated fatty acids and sugars [11], play a crucial role in complications associated with metabolic syndrome such as diabetes and cardiovascular and renal diseases [12]. Fig. 1 presents the common reactive carbonyl species derived from lipid peroxidation and oxidation of sugars. The electrophilic nature of reactive carbonyl species (Fig. 2) allows for reaction with the nucleophilic sites of proteins as well as with DNA bases [13,14]. As shown in Fig. 3, these types of glycoxidation and lipoxidation processes can cause irreversible cellular dysfunction [15,16].
Advanced glycoxidation/lipoxidation end products (AGEs/ALES) are a group of molecules generated by nonenzymatic covalent bonding of free amino groups of proteins and nucleic acids with glucose residues and lipids [17]. The concentrations of AGEs and ALEs in tissue and circulation are reportedly related to various oxidative stress associated diseases. The serum concentrations of N-ɛ-(carboxymethyl) lysine (CML), the most well studied AGEs, are associated with complications of diabetes such as retinopathy [18], microangiopathy [19], and chronic kidney diseases [20]. The damaging effect of AGEs/ALEs can be mediated by various mechanisms such as dysfunction of proteins undergoing oxidative modification, protein aggregation, alteration of signal transduction, and immunoresponses. Although substantial knowledge gaps still exist regarding how AGEs/ALEs lead to cellular dysfunction in vivo, they have been recognized as important pathogenic factors of certain oxidative stress associated diseases.
The receptor for advanced glycoxidation end products (RAGE) is a multiligand transmembrane receptor [21]. Binding of AGEs to RAGE activates NFkB, which induces production of various inflammatory cytokines such as IL-1, IL-6 and TNF-α and further generation of mitochondrial reactive oxygen species (ROS). Therefore, it has been suggested that RAGE is a master switch that converts a transient proinflammatory response into cellular dysfunction [22]. The AGEs-RAGE interaction also reportedly alters cellular signaling and promotes gene expression [23]. The excess ROS produced by RAGE activation can also lead to increased mitochondrial superoxide [24]. It is not surprising that pharmacologic inhibition of cytosolic ROS production has been suggested as an appropriate strategy to reduce excessive mitochondrial superoxide production in diabetic complications [24]. Binding of AGEs to the V region of RAGE is initially facilitated by the ionic attraction between positive charges of RAGE and negative charges of AGE. The binding complex is then stabilized by hydrophobic interaction after conformational changes [25]. However, it is still not known whether ALEs can also bind to RAGE.
Histidine-dipeptides such as carnosine (β-alanyl-L-histidine) and anserine (N-β-alanyl-3-methyl-L-histidine) (Fig. 4) are widely distributed in skeletal muscles, the heart and the central nervous system at very high concentrations (up to 20 mM) [26]. Therefore, substantial amounts of carnosine and anserine can be easily obtained from animal protein such as beef [27], chicken, pork and fish [28,29].
In humans, carnosine is synthesized from two constitutive amino acids by carnosine synthase (EC 6.3.2.11) [30], and is degraded by carnosinase. Two different carnosinase iso-forms have been identified to date: a cytosolic form (tissue carnosinase, CN2, EC 3.4.13.18) that acts as a non-specific dipeptidase, and a highly specific metal-ion dependent homodimeric dipeptidase, serum carnosinase (CN1, EC 3.4.13.20), which is found in both serum and brain [31].
Although carnosine was detected in the plasma of healthy young men and women (18-25 yr) after ingesting 200 g of beef [27], another study did not detect any carnosine after ingesting the same amount of beef or chicken [32]. On the other hand, anserine was detected in circulation after ingesting chicken in this study [32]. The Cmax of anserine in plasma was reported to be 2.72 ± 1.08 µM at 100 min after ingesting 150 g of chicken breast. Although the biochemical function of histidine-dipeptides is still not clear, there is evidence that histidine-dipeptides could act as both a direct antioxidant by scavenging peroxyl radicals and singlet oxygen, and as an indirect antioxidant by deactivating the pro-oxidant effect of transition metal ions through a chelating mechanism [33-35].
In addition, hisitidine-dipeptides could act as an efficient scavenger of reactive carbonyl species generated by glucose and lipid oxidation such as methylglyoxal [36], acrolein [37] and hydroxynonenal [38]. Therefore, dietary histidine-dipeptides could be considered as a promising bioactive agent. Unlike conventional antioxidants that act as prooxidants [39-42] and/or produce toxic metabolites [43,44], carnosine metabolite is not toxic [45] and is safe at high doses [46].
To understand the function of a certain bioactive material in vivo, it is essential to understand its bioavailability. Even though the above reported observations have potentially large relevance for human health, knowledge regarding absorption and bioavailability of histidine-dipeptides in humans is scant. Indeed, only a few controversial studies regarding the absorption, distribution, metabolism and excretion profiles of carnosine and other histidine-dipeptides have been conducted [27,47]. Rodent studies have shown that carnosine is actively transported across the brush border membrane via the peptide transporter PEPT1 [48]. However, studies conducted in rodents cannot be extrapolated to humans because they lack serum carnosinases, which are assumed to be primarily involved in the metabolic fate of carnosine in humans. The absorption kinetics of histidine-dipeptides in humans has been reported in healthy adults after ingesting chicken. As described earlier, the blood and urine concentrations of histidine-dipeptides and β-alanine were measured in this study [32]. Anserine (β-alanyl 3-methyl L-histidine) concentration was significantly increased within 90 min. β-Alanine, a hydrolysis product of carnosine and anserine, also showed similar kinetics. Considering the amounts of carnosine and anserine contained in chicken and the preferential affinity of carnosinase to carnosine compared to that of anserine, it is not surprising that carnosine was not detected in circulation in these subjects. It should be noted that both carnosine and anserine were significantly up-regulated in urine after ingesting chicken. The higher urinary concentration of anserine compared to carnosine also reflects the higher content ratio of anserine/carnosine in chicken breast. It is plausible that the carnosinase efficiently hydrolyzes the carnosine, but not anserine.
It is important to understand gene-nutrient interactions because such interactions cause dietary interventions to be less successful in some individuals than in others, as shown in vitamin C-glutathione S-transferase [49] and vitamin E-haptoglobin [50] interactions. A link between low serum concentrations of carnosine and the risk for the complication of diabetes has been proposed. Two independent studies have revealed an association between a trinucleotide repeat in exon 2 of the CNDP1 gene, which encodes carnosinase, and diabetic nephropathy [51,52]. Subjects who have the 5-6, 5-7, 6-6, and 6-7 alleles of the CNDP1 gene showed elevated serum carnosinase activity. It is not surprising that diabetic patients with the 5-5 allele (~1/3 of study population) are less susceptible to nephropathy [51,52], nor that increased carnosinase activity is shown in subjects with nephropathy. It is evident that over-expression of carnosinase would cause enhanced carnosine degradation, resulting in a lower renal protective effect by carnosine, since serum and urine carnosine concentrations depend on carnosinase activity [51,52]. This finding has been supported by additional in vitro studies, showing that carnosine protects renal cells against the deleterious effect of high glucose levels [51].
The carbonyl groups on amino acid residues are generated by peroxidation of polyunsaturated fatty acids [53]. The electrophilic nature of the carbonyl compounds, mainly α-β-unsaturated aldehydes, leads to reaction with the nucleophilic sites of proteins, as well as with DNA bases [13,14]. In particular, 4-hydroxytrans-2-nonenal (HNE) is one of the most abundant and toxic lipid-derived α-β-unsaturated aldehydes generated through the β-cleavage of hydroperoxides from ω-6 polyunsaturated fatty acids. As discussed, the α-β-unsaturated aldehydes have a wide range of biological activities including inhibition of protein and DNA synthesis, inactivation of enzymes, reduction of gap-junction communication, stimulation of neutrophil chemotaxis and modulation of platelet aggregation [13,14]. It has also been reported that the elevation of 4-hydroxy-trans-2-noneal is related to the degree of cognitive impairment [54]. Protein carbonylation induced by reactive carbonyl compounds may play a significant role in the etiology and/or progression of several human diseases, such as cardiovascular and neurodegenerative diseases.
Unlike conventional antioxidants, which have been shown to be ineffective [41,55] and even harmful [39, 40] against chronic diseases, histidine-dipeptides have the potential to exert targeted action. As shown in Fig. 5, histidine-dipeptides can directly counteract cytotoxic reactive carbonyl species, resulting in an unreactive adduct [38]. Although the biological functions of histidine-dipeptides are still not clear, our in vitro studies have shown that carnosine and anserine effectively detoxify one of the most abundant and toxic lipid-derived reactive carbonyl species, 4-hydroxy-trans-2-nonenal (HNE). Notably, histidine is a primary reaction site of HNE adduction because it represents one of the most reactive nucleophilic residues in protein [56]. Therefore, new approaches using the histidine-dipeptides rich dietary intervention can be a more targeted strategy against oxidative damage than standard approaches using conventional antioxidants. As shown in Fig. 6, histidine-dipeptides can remove reactive oxygen species before they produce cytotoxic reactive carbonyl species and by direct sequestering of reactive carbonyl species.
The covalent modifications of AGEs and ALEs can induce functional derangement (e.g., structural or enzymatic) of the protein itself due to protein conformational changes or as a consequence of catalytic site distortion or impairment caused by the covalent modification. AGEs and ALEs protein modifications can also induce signal transduction, resulting in a damaging response [17]. It has been reported that carnosine can inhibit protein glycation as well as reverse glycated proteins through a transglycation mechanism [58,59]. In vivo studies using different animal models have clearly demonstrated the ability of histidine-dipeptides to inhibit AGEs formation and prevent RAGE activation. However the molecular mechanism/s through which these effects occur have not yet been elucidated.
Our previous study with Zucker obese rats indicated that histidine-dipeptides such as carnosine supplementation significantly reduce the development of dyslipidemia, hypertension and renal injury through an anticarbonylation and antiglycation mechanism [59]. In particular, urinary AGEs levels were 182.9 ± 24.4 FU/mg creatinine/day in lean rats, 610.1 ± 36.6 in Zucker obese rats, and 337.2 ± 28.7 in Zucker obese rats treated with histidine-dipeptides, carnosine (30 mg/Kg daily for 24 weeks). The effects of histidine-dipeptides on AGEs has also been confirmed in other experimental models such as ApoE null mice feeding on a western diet. In these animals, elevated AGEs were found to be associated with RAGE over-expression leading to inflammatory response in both the aorta and kidney and with fibrosis leading to atherosclerosis and kidney disease. It is highly plausible that the beneficial effects of histidine-dipeptides presented in these studies are mediated by disruption of the ALEs/AGEs-RAGE- pro-inflammatory axis [25].
Peptidomic and albuminomic approaches that use a highly sensitive and accurate (< 3 ppm) ORBITRAP LC-ESI-MS/MS technique have enabled determination of the cytotoxic reactive carbonyl species-amino acid adducts in circulation and urine. Human serum albumin has recently been shown to act as an efficient detoxifying agent of cytotoxic reactive carbonyl species [60,61]. In particular, Cys-34 in albumin efficiently removes cytotoxic carbonyls due to the nucleophilicity of its Cys-34 residue. The reactive carbonyl species quenching ability of human serum albumin is due to the nucleophilicity of the Cys-34 residue, which is able to react with the electrophilic carbon of the reactive carbonyl species through a michael adduction, leading to unreactive and stable covalent adducts. In addition to allowing measurement of Cys-34, the LC-ESI-MS/MS approach enables identification of reactive carbonyl species induced covalent modifications of various labile sites of serum albumin. Reactive carbonyl species can also directly react with nucleophilic sites of DNA, as shown in in vitro studies [62-64].
The oxidizing species of albumin forms the corresponding sulfenic acid, which then reacts with extracellular cysteine, leading to the corresponding cysteinylated form. The sulfenic acid residue can also be further oxidized to sulfinic or sulfonic acid residues, which are the irreversible oxidized forms of cysteine. Our preliminary study indicated that the metabolic syndrome subject (n = 8) showed a significantly higher (P < 0.05) cysteinylated form (52 ± 4%) relative to the age-matched healthy subjects (40 ± 6%). Therefore, the covalent oxidative modification of albumin (e.g. Cys-34 modification) can be used as a new and novel biomarker of oxidative damage in vivo and utilized to determine the effects of reactive carbonyl species quenching agents such as histidine-dipeptides on its modification.
Histidine-dipeptides have been shown to be beneficial in a variety of disease models in which chronic oxidative or glycative stress is a characteristic feature [51,65-68]. There is also compelling evidence that histidine-dipeptides mediate their health-promoting effects in these models by decreasing the levels of advanced glycoxidattion (AGEs) and lipoxidation end-products (ALEs), thereby blocking the damaging axis of AGEs/ALEs-RAGE. In addition, a possible role of carnosine in regulating blood glucose through controlling autonomic nerves has been reported [69]. The reduced muscle carnosine in type 2 diabetic patients is noteworthy [70]. A significant correlation was observed between serum carnosine concentrations and β-cell mass in the pancreas [71]. Although it was shown in an animal model, oral administration of carnosine prevented stress-induced decline in glucose tolerance and glycogen content in liver and muscle, and reduced plasma corticosterone levels [72]. The optimal concentration of histidine-dipeptides that elicit beneficial effects is not yet known. To the best of our knowledge, no intervention studies have evaluated the efficacy toward histidine-dipeptide on metabolic syndrome to date. However, it has been reported that L-carnosine supplementation (800 mg/d for 8 wks) enhanced neurologic function in children with autistic spectrum disorders [73]. Further evidence of the biological functions of hisitidine-dipeptides would lead to a personalized dietary defense strategy and/or discovery of drugs for treatment of oxidative stress-associated chronic diseases that are effective, non-toxic and not affected by genetics.
Metabolic syndrome, which is associated with a state of elevated oxidative stress and inflammation, often leads to chronic diseases such as diabetes and cardiovascular and kidney diseases. Dietary histidine-dipeptides such as carnosine and anserine may prevent progression of metabolic syndrome by quenching cytotoxic reactive carbonyl species as well as AGEs/ALEs and preventing the over-expression of RAGE triggering inflammation. The effectiveness of dietary histidine-dipeptides against metabolic disorders may depend on the genotype of the carnosinase encoding gene, CNDP1. Further intervention trials utilizing dietary histidine-dipeptides are warranted to provide effective personalized defense strategies against progression of metabolic syndrome in the form of a new prevention strategy.
Figures and Tables
Fig. 1
Structures of reactive carbonyl species derived from (A) lipid peroxidation and (B) sugar oxidation
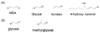
Fig. 2
Mesomeric equilibrium of the reactivity carbonyl species, 4-hydroxy-trans-2-nonenal, producing a strong electrophilic center

Fig. 3
Reactive carbonyl species produced by the oxidation of polyunsaturated fatty acid and sugars react with protein, producing advanced glycoxidation and lipoxidation end products (AGEs/ALEs), which can cause irreversible cellular dysfunction. PUFA, polyunsaturated fatty acids; AGEs, advanced glycoxidation end products; ALEs, advanced lipoxidation end products
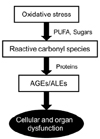
Fig. 5
Cytotoxic reactive carbonyl species sequestering action of the histidine-dipeptides (i.e. carnosine). HNE, 4-hydroxy-trans-2-nonenal
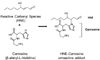
Fig. 6
Histidine-dipeptides can act at two key points of the oxidative stress cascade: by removing reactive oxygen species before producing cytotoxic reactive carbonyl species, and by directly sequestering reactive carbonyl species. HD, histidine-dipeptides; RCS, reactive carbonyl species; AGEs, advanced glycoxidation products, ALEs, advanced lipoxidation end products
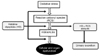
References
1. Alberti KG, Eckel RH, Grundy SM, Zimmet PZ, Cleeman JI, Donato KA, Fruchart JC, James WP, Loria CM, Smith SC Jr. International Diabetes Federation Task Force on Epidemiology and Prevention. Hational Heart, Lung, and Blood Institute. American Heart Association. World Heart Federation. International Atherosclerosis Society. International Association for the Study of Obesity. Harmonizing the metabolic syndrome: a joint interim statement of the International Diabetes Federation Task Force on Epidemiology and Prevention; National Heart, Lung, and Blood Institute; American Heart Association; World Heart Federation; International Atherosclerosis Society; and International Association for the Study of Obesity. Circulation. 2009; 120:1640–1645.
2. Ford ES, Giles WH, Mokdad AH. Increasing prevalence of the metabolic syndrome among U.S. adults. Diabetes Care. 2004; 27:2444–2449.


3. Lim S, Shin H, Song JH, Kwak SH, Kang SM, Won Yoon J, Choi SH, Cho SI, Park KS, Lee HK, Jang HC, Koh KK. Increasing prevalence of metabolic syndrome in Korea: the Korean National Health and Nutrition Examination Survey for 1998-2007. Diabetes Care. 2011; 34:1323–1328.
4. Ervin RB. Prevalence of metabolic syndrome among adults 20 years of age and over, by sex, age, race and ethnicity, and body mass index: United States, 2003-2006. Natl Health Stat Report. 2009; 1–7.
5. Furukawa S, Fujita T, Shimabukuro M, Iwaki M, Yamada Y, Nakajima Y, Nakayama O, Makishima M, Matsuda M, Shimomura I. Increased oxidative stress in obesity and its impact on metabolic syndrome. J Clin Invest. 2004; 114:1752–1761.


6. Dandona P, Mohanty P, Ghanim H, Aljada A, Browne R, Hamouda W, Prabhala A, Afzal A, Garg R. The suppressive effect of dietary restriction and weight loss in the obese on the generation of reactive oxygen species by leukocytes, lipid peroxidation, and protein carbonylation. J Clin Endocrinol Metab. 2001; 86:355–362.


7. Caimi G, Hopps E, Noto D, Canino B, Montana M, Lucido D, Lo Presti R, Averna MR. Protein oxidation in a group of subjects with metabolic syndrome. Diabetes Metab Syndr. 2013; 7:38–41.


8. Festa A, D'Agostino R Jr, Williams K, Karter AJ, Mayer-Davis EJ, Tracy RP, Haffner SM. The relation of body fat mass and distribution to markers of chronic inflammation. Int J Obes Relat Metab Disord. 2001; 25:1407–1415.


9. Ford ES, Schulze MB, Pischon T, Bergmann MM, Joost HG, Boeing H. Metabolic syndrome and risk of incident diabetes: findings from the European Prospective Investigation into Cancer and Nutrition-Potsdam Study. Cardiovasc Diabetol. 2008; 7:35.


10. Grundy SM. A constellation of complications: the metabolic syndrome. Clin Cornerstone. 2005; 7:36–45.
11. Carini M, Aldini G, Facino RM. Mass spectrometry for detection of 4-hydroxy-trans-2-nonenal (HNE) adducts with peptides and proteins. Mass Spectrom Rev. 2004; 23:281–305.


12. Pedchenko VK, Chetyrkin SV, Chuang P, Ham AJ, Saleem MA, Mathieson PW, Hudson BG, Voziyan PA. Mechanism of perturbation of integrin-mediated cell-matrix interactions by reactive carbonyl compounds and its implication for pathogenesis of diabetic nephropathy. Diabetes. 2005; 54:2952–2960.


13. Uchida K. Role of reactive aldehyde in cardiovascular diseases. Free Radic Biol Med. 2000; 28:1685–1696.


14. Poli G, Schaur RJ. 4-Hydroxynonenal in the pathomechanisms of oxidative stress. IUBMB Life. 2000; 50:315–321.


15. Dalle-Donne I, Aldini G, Carini M, Colombo R, Rossi R, Milzani A. Protein carbonylation, cellular dysfunction, and disease progression. J Cell Mol Med. 2006; 10:389–406.


16. Harcourt BE, Sourris KC, Coughlan MT, Walker KZ, Dougherty SL, Andrikopoulos S, Morley AL, Thallas-Bonke V, Chand V, Penfold SA, de Courten MP, Thomas MC, Kingwell BA, Bierhaus A, Cooper ME, de Courten B, Forbes JM. Targeted reduction of advanced glycation improves renal function in obesity. Kidney Int. 2011; 80:190–198.


17. Singh DK, Winocour P, Farrington K. Oxidative stress in early diabetic nephropathy: fueling the fire. Nat Rev Endocrinol. 2011; 7:176–184.


18. Ghanem AA, Elewa A, Arafa LF. Pentosidine and N-carboxymethyl-lysine: biomarkers for type 2 diabetic retinopathy. Eur J Ophthalmol. 2011; 21:48–54.


19. Hirata K, Kubo K. Relationship between blood levels of N-carboxymethyl-lysine and pentosidine and the severity of microangiopathy in type 2 diabetes. Endocr J. 2004; 51:537–544.


20. Dworacka M, Winiarska H, Szymańska M, Szczawińska K, Wierusz-Wysocka B. Serum N-epsilon-(carboxymethyl)lysine is elevated in nondiabetic coronary heart disease patients. J Basic Clin Physiol Pharmacol. 2002; 13:201–213.
21. Ramasamy R, Yan SF, Schmidt AM. The diverse ligand repertoire of the receptor for advanced glycation endproducts and pathways to the complications of diabetes. Vascul Pharmacol. 2012; 57:160–167.


22. Bierhaus A, Nawroth PP. Multiple levels of regulation determine the role of the receptor for AGE (RAGE) as common soil in inflammation, immune responses and diabetes mellitus and its complications. Diabetologia. 2009; 52:2251–2263.


23. Barlovic DP, Soro-Paavonen A, Jandeleit-Dahm KA. RAGE biology, atherosclerosis and diabetes. Clin Sci (Lond). 2011; 121:43–55.


24. Coughlan MT, Thorburn DR, Penfold SA, Laskowski A, Harcourt BE, Sourris KC, Tan AL, Fukami K, Thallas-Bonke V, Nawroth PP, Brownlee M, Bierhaus A, Cooper ME, Forbes JM. RAGE-induced cytosolic ROS promote mitochondrial superoxide generation in diabetes. J Am Soc Nephrol. 2009; 20:742–752.


25. Aldini G, Vistoli G, Stefek M, Chondrogianni N, Grune T, Sereikaite J, Sadowska-Bartosz I, Bartosz G. Molecular strategies to prevent, inhibit, and degrade advanced glycoxidation and advanced lipoxidation end products. Free Radic Res. 2013; 47:Suppl 1. 93–137.


26. Quinn PJ, Boldyrev AA, Formazuyk VE. Carnosine: its properties, functions and potential therapeutic applications. Mol Aspects Med. 1992; 13:379–444.


27. Park YJ, Volpe SL, Decker EA. Quantitation of carnosine in humans plasma after dietary consumption of beef. J Agric Food Chem. 2005; 53:4736–4739.


28. Chan KM, Decker EA. Endogenous skeletal muscle antioxidants. Crit Rev Food Sci Nutr. 1994; 34:403–426.


29. Gil-Agustí M, Esteve-Romero J, Carda-Broch S. Anserine and carnosine determination in meat samples by pure micellar liquid chromatography. J Chromatogr A. 2008; 1189:444–450.


30. Horinishi H, Grillo M, Margolis FL. Purification and characterization of carnosine synthetase from mouse olfactory bulbs. J Neurochem. 1978; 31:909–919.


31. Teufel M, Saudek V, Ledig JP, Bernhardt A, Boularand S, Carreau A, Cairns NJ, Carter C, Cowley DJ, Duverger D, Ganzhorn AJ, Guenet C, Heintzelmann B, Laucher V, Sauvage C, Smirnova T. Sequence identification and characterization of human carnosinase and a closely related non-specific dipeptidase. J Biol Chem. 2003; 278:6521–6531.


32. Yeum KJ, Orioli M, Regazzoni L, Carini M, Rasmussen H, Russell RM, Aldini G. Profiling histidine dipeptides in plasma and urine after ingesting beef, chicken or chicken broth in humans. Amino Acids. 2010; 38:847–858.


33. Kohen R, Yamamoto Y, Cundy KC, Ames BN. Antioxidant activity of carnosine, homocarnosine, and anserine present in muscle and brain. Proc Natl Acad Sci U S A. 1988; 85:3175–3179.


34. Kang JH, Kim KS, Choi SY, Kwon HY, Won MH, Kang TC. Carnosine and related dipeptides protect human ceruloplasmin against peroxyl radical-mediated modification. Mol Cells. 2002; 13:498–502.
35. Egorov SYu, Kurella EG, Boldyrev AA, Krasnovsky AA Jr. Quenching of singlet molecular oxygen by carnosine and related antioxidants. Monitoring 1270-nm phosphorescence in aqueous media. Biochem Mol Biol Int. 1997; 41:687–694.


36. Brownson C, Hipkiss AR. Carnosine reacts with a glycated protein. Free Radic Biol Med. 2000; 28:1564–1570.


37. Carini M, Aldini G, Beretta G, Arlandini E, Facino RM. Acrolein-sequestering ability of endogenous dipeptides: characterization of carnosine and homocarnosine/acrolein adducts by electrospray ionization tandem mass spectrometry. J Mass Spectrom. 2003; 38:996–1006.


38. Aldini G, Carini M, Beretta G, Bradamante S, Facino RM. Carnosine is a quencher of 4-hydroxy-nonenal: through what mechanism of reaction? Biochem Biophys Res Commun. 2002; 298:699–706.


39. Miller ER 3rd, Pastor-Barriuso R, Dalal D, Riemersma RA, Appel LJ, Guallar E. Meta-analysis: high-dosage vitamin E supplementation may increase all-cause mortality. Ann Intern Med. 2005; 142:37–46.


40. Lawson KA, Wright ME, Subar A, Mouw T, Hollenbeck A, Schatzkin A, Leitzmann MF. Multivitamin use and risk of prostate cancer in the National Institutes of Health-AARP Diet and Health Study. J Natl Cancer Inst. 2007; 99:754–764.


41. Bjelakovic G, Nikolova D, Gluud LL, Simonetti RG, Gluud C. Antioxidant supplements for prevention of mortality in healthy participants and patients with various diseases. Cochrane Database Syst Rev. 2012; 3:CD007176.


42. Rietjens IM, Boersma MG, Haan Ld, Spenkelink B, Awad HM, Cnubben NH, van Zanden JJ, Woude Hv, Alink GM, Koeman JH. The pro-oxidant chemistry of the natural antioxidants vitamin C, vitamin E, carotenoids and flavonoids. Environ Toxicol Pharmacol. 2002; 11:321–333.


43. Veeramachaneni S, Ausman LM, Choi SW, Russell RM, Wang XD. High dose lycopene supplementation increases hepatic cytochrome P4502E1 protein and inflammation in alcohol-fed rats. J Nutr. 2008; 138:1329–1335.


44. van Helden YG, Keijer J, Knaapen AM, Heil SG, Briedé JJ, van Schooten FJ, Godschalk RW. Beta-carotene metabolites enhance inflammation-induced oxidative DNA damage in lung epithelial cells. Free Radic Biol Med. 2009; 46:299–304.


45. Hipkiss AR, Michaelis J, Syrris P. Non-enzymatic glycosylation of the dipeptide L-carnosine, a potential anti-protein-cross-linking agent. FEBS Lett. 1995; 371:81–85.


46. Sato M, Karasawa N, Shimizu M, Morimatsu F, Yamada R. Safety evaluation of chicken breast extract containing carnosine and anserine. Food Chem Toxicol. 2008; 46:480–489.


47. Gardner ML, Illingworth KM, Kelleher J, Wood D. Intestinal absorption of the intact peptide carnosine in man, and comparison with intestinal permeability to lactulose. J Physiol. 1991; 439:411–422.


48. Son DO, Satsu H, Kiso Y, Shimizu M. Characterization of carnosine uptake and its physiological function in human intestinal epithelial Caco-2 cells. Biofactors. 2004; 21:395–398.


49. Cahill LE, Fontaine-Bisson B, El-Sohemy A. Functional genetic variants of glutathione S-transferase protect against serum ascorbic acid deficiency. Am J Clin Nutr. 2009; 90:1411–1417.


50. Milman U, Blum S, Shapira C, Aronson D, Miller-Lotan R, Anbinder Y, Alshiek J, Bennett L, Kostenko M, Landau M, Keidar S, Levy Y, Khemlin A, Radan A, Levy AP. Vitamin E supplementation reduces cardiovascular events in a subgroup of middle-aged individuals with both type 2 diabetes mellitus and the haptoglobin 2-2 genotype: a prospective double-blinded clinical trial. Arterioscler Thromb Vasc Biol. 2008; 28:341–347.


51. Janssen B, Hohenadel D, Brinkkoetter P, Peters V, Rind N, Fischer C, Rychlik I, Cerna M, Romzova M, de Heer E, Baelde H, Bakker SJ, Zirie M, Rondeau E, Mathieson P, Saleem MA, Meyer J, Köppel H, Sauerhoefer S, Bartram CR, Nawroth P, Hammes HP, Yard BA, Zschocke J, van der Woude FJ. Carnosine as a protective factor in diabetic nephropathy: association with a leucine repeat of the carnosinase gene CNDP1. Diabetes. 2005; 54:2320–2327.
52. Freedman BI, Hicks PJ, Sale MM, Pierson ED, Langefeld CD, Rich SS, Xu J, McDonough C, Janssen B, Yard BA, van der Woude FJ, Bowden DW. A leucine repeat in the carnosinase gene CNDP1 is associated with diabetic end-stage renal disease in European Americans. Nephrol Dial Transplant. 2007; 22:1131–1135.


53. Stadtman ER. Metal ion-catalyzed oxidation of proteins: biochemical mechanism and biological consequences. Free Radic Biol Med. 1990; 9:315–325.


54. McGrath LT, McGleenon BM, Brennan S, McColl D, McILroy S, Passmore AP. Increased oxidative stress in Alzheimer's disease as assessed with 4-hydroxynonenal but not malondialdehyde. QJM. 2001; 94:485–490.


55. Neuhouser ML, Wassertheil-Smoller S, Thomson C, Aragaki A, Anderson GL, Manson JE, Patterson RE, Rohan TE, van Horn L, Shikany JM, Thomas A, LaCroix A, Prentice RL. Multivitamin use and risk of cancer and cardiovascular disease in the Women's Health Initiative cohorts. Arch Intern Med. 2009; 169:294–304.


56. Aldini G, Granata P, Orioli M, Santaniello E, Carini M. Detoxification of 4-hydroxynonenal (HNE) in keratinocytes: characterization of conjugated metabolites by liquid chromatography/electrospray ionization tandem mass spectrometry. J Mass Spectrom. 2003; 38:1160–1168.


57. Seidler NW, Yeargans GS, Morgan TG. Carnosine disaggregates glycated alpha-crystallin: an in vitro study. Arch Biochem Biophys. 2004; 427:110–115.


58. Seidler NW. Carnosine prevents the glycation-induced changes in electrophoretic mobility of aspartate aminotransferase. J Biochem Mol Toxicol. 2000; 14:215–220.


59. Aldini G, Orioli M, Rossoni G, Savi F, Braidotti P, Vistoli G, Yeum KJ, Negrisoli G, Carini M. The carbonyl scavenger carnosine ameliorates dyslipidaemia and renal function in Zucker obese rats. J Cell Mol Med. 2011; 15:1339–1354.


60. Aldini G, Regazzoni L, Orioli M, Rimoldi I, Facino RM, Carini M. A tandem MS precursor-ion scan approach to identify variable covalent modification of albumin Cys34: a new tool for studying vascular carbonylation. J Mass Spectrom. 2008; 43:1470–1481.


61. Aldini G, Vistoli G, Regazzoni L, Gamberoni L, Facino RM, Yamaguchi S, Uchida K, Carini M. Albumin is the main nucleophilic target of human plasma: a protective role against pro-atherogenic electrophilic reactive carbonyl species? Chem Res Toxicol. 2008; 21:824–835.


62. Okada K, Wangpoengtrakul C, Osawa T, Toyokuni S, Tanaka K, Uchida K. 4-Hydroxy-2-nonenal-mediated impairment of intracellular proteolysis during oxidative stress. Identification of proteasomes as target molecules. J Biol Chem. 1999; 274:23787–23793.


63. Mistry N, Bevan RJ, Cooke MS, Evans MD, Halligan EP, Lowes DA, Nichol K, Lunec J. Antiserum detection of reactive carbonyl species-modified DNA in human colonocytes. Free Radic Res. 2008; 42:344–353.


64. Roberts MJ, Wondrak GT, Laurean DC, Jacobson MK, Jacobson EL. DNA damage by carbonyl stress in human skin cells. Mutat Res. 2003; 522:45–56.


65. Gallant S, Semyonova M, Yuneva M. Carnosine as a potential anti-senescence drug. Biochemistry (Mosc). 2000; 65:866–868.
66. Hipkiss AR. Glycation, ageing and carnosine: are carnivorous diets beneficial? Mech Ageing Dev. 2005; 126:1034–1039.


67. Lee YT, Hsu CC, Lin MH, Liu KS, Yin MC. Histidine and carnosine delay diabetic deterioration in mice and protect human low density lipoprotein against oxidation and glycation. Eur J Pharmacol. 2005; 513:145–150.


68. Kurata H, Fujii T, Tsutsui H, Katayama T, Ohkita M, Takaoka M, Tsuruoka N, Kiso Y, Ohno Y, Fujisawa Y, Shokoji T, Nishiyama A, Abe Y, Matsumura Y. Renoprotective effects of l-carnosine on ischemia/reperfusion-induced renal injury in rats. J Pharmacol Exp Ther. 2006; 319:640–647.


69. Nagai K, Niijima A, Yamano T, Otani H, Okumra N, Tsuruoka N, Nakai M, Kiso Y. Possible role of L-carnosine in the regulation of blood glucose through controlling autonomic nerves. Exp Biol Med (Maywood). 2003; 228:1138–1145.


70. Gualano B, Everaert I, Stegen S, Artioli GG, Taes Y, Roschel H, Achten E, Otaduy MC, Junior AH, Harris R, Derave W. Reduced muscle carnosine content in type 2, but not in type 1 diabetic patients. Amino Acids. 2012; 43:21–24.


71. Sauerhöfer S, Yuan G, Braun GS, Deinzer M, Neumaier M, Gretz N, Floege J, Kriz W, van der Woude F, Moeller MJ. L-carnosine, a substrate of carnosinase-1, influences glucose metabolism. Diabetes. 2007; 56:2425–2432.