Abstract
BACKGROUND/OBJECTIVES
Non-alcoholic fatty liver disease (NAFLD) is becoming an important public health problem as metabolic syndrome and type 2 diabetes have become epidemic. In this study we investigated the protective effect of Cordyceps militaris (C. militaris) against NAFLD in an obese mouse model.
MATERIALS/METHODS
Four-week-old male ob/ob mice were fed an AIN-93G diet or a diet containing 1% C. militaris water extract for 10 weeks after 1 week of adaptation. Serum glucose, insulin, free fatty acid (FFA), alanine transaminase (ALT), and proinflammatory cytokines were measured. Hepatic levels of lipids, glutathione (GSH), and lipid peroxide were determined.
RESULTS
Consumption of C. militaris significantly decreased serum glucose, as well as homeostasis model assessment for insulin resistance (HOMA-IR), in ob/ob mice. In addition to lowering serum FFA levels, C. militaris also significantly decreased hepatic total lipids and triglyceride contents. Serum ALT activities and tumor necrosis factor-α (TNF-α) and interleukin-6 (IL-6) levels were reduced by C. militaris. Consumption of C. militaris increased hepatic GSH and reduced lipid peroxide levels.
Non-alcoholic fatty liver disease (NAFLD) refers to a spectrum of liver diseases, which include hepatic steatosis (fatty liver), non-alcoholic steatohepatitis (NASH), and advanced fibrosis and cirrhosis in the absence of chronic alcohol use [1]. NAFLD is the most common cause of liver disease [2]. Although relatively benign, steatosis can progress to NASH, an extreme form of NAFLD, and NASH can eventually develop into liver cirrhosis [3]. NAFLD has also been suggested to potentiate liver damage induced by other factors, including alcohol, toxins, and viruses [4]. NAFLD prevalence is estimated at 15-40% in Western countries and 9-40% in Asia [5].
Although the underlying mechanism of NAFLD is not clear, the '2-hit hypothesis' was proposed to explain the pathogenesis [3,6]. The 'first hit' is accumulation of triglycerides in the liver (steatosis), which is strongly associated with insulin resistance. The fatty liver is susceptible to injury mediated by the 'second hit', which includes inflammatory adipokines/cytokines, oxidative stress, and mitochondrial dysfunction, leading to steatohepatitis and fibrosis. Insulin resistance is a major pathology underlying the development and progression of NASH [7]. Proinflammatory cytokines such as tumor necrosis factor-α (TNF-a) and interleukin-6 (IL-6) have been suggested to play a crucial role in the development of insulin resistance [8].
Because metabolic syndrome and type 2 diabetes are important risk factors for NAFLD [9], NAFLD is becoming an important public health problem as these have become epidemic [10]. Although numerous therapeutic agents have been postulated to treat NAFLD [11], no pharmacological treatment is to date known [12].
The Cordyceps species are entomopathogenic fungi that are used as medicinal mushrooms in eastern Asia [13]. Among them, Cordyceps sinensis (C. sinensis) is the most valued mushroom, which has pharmacological effects that are used in traditional Chinese medicine. However, natural C. sinensis is scarce and highly expensive. Therefore, Cordyceps militaris (C. militaris) is a prominent substitute for C. sinensis due to its similar composition and pharmacological effects to C. sinensis and reasonable price [14].
C. militaris has shown antioxidant activity in vitro [15,16]. C. militaris extract has free radical scavenging activity and has been reported to have hepatoprotective activity in vitro [17]. C. militaris extract has alleviated oxidative injury in HepG2 cells induced by tert-butyl hydroperoxide (t-BHP) by reducing reactive oxygen species (ROS) generation and thiobarbituric acid reactive substances (TBARS) formation. In addition, C. militaris has demonstrated anti-inflammatory activity in vitro [18]. A hot water extract of C. militaris reduced production of nitric oxide (NO) and secretion of TNF-α and IL-6 induced by lipopolysaccharide (LPS) in macrophages. These findings suggest that C. militaris could play a beneficial role in alleviation of NAFLD by improving oxidative stress and reducing inflammation.
However, the beneficial effects of C. militaris on NAFLD have not been fully investigated. Therefore, in this study, the protective effect of C. militaris against NAFLD was investigated in leptin-deficient ob/ob mice, which show obesity, insulin resistance, and hyperglycemia and are used as an animal model of NAFLD [19,20,21,22].
All animal experiments were approved by the Animal Resource Center at our university (approval no. 2011-44). Four-week-old male C57BL/6-Lepob/ob mice (n=16) were obtained from Korea Research Institute of Bioscience and Biotechnology, Ochang, Korea. The mice were housed individually under temperature (24 ± 5℃), humidity (55 ± 5%), and light (12 h light/dark cycle) controlled conditions. After acclimating for 1 week, the animals were randomly divided into two groups. The control group was offered an AIN-93G diet [25], while the C. militaris group was fed a diet containing 1% C. militaris water extract in place of the Alphacel ad libitum for 10 weeks.
At the end of the experiment, the mice were sacrificed by cardiac puncture following an overnight fast. Blood and liver samples were collected and serum was separated by centrifugation of blood samples at 1,500 g for 15 min. Serum and liver samples were stored at -70℃ for further analysis.
Serum glucose levels were measured by an enzymatic method using a commercial kit (Asan Pharmaceutical Co., Seoul, Korea). Insulin levels were determined using radioimmunoassay kits (Linco Co., St. Charles, MO, USA). The homeostasis model assessment of insulin resistance (HOMA-IR) was estimated by dividing the product of fasting glucose (mg/dL) and insulin levels (ng/mL) by 405 [26].
Serum FFA was measured using an assay kit as described by the manufacturer (Bioassay System, Hayward, CA, USA). To determine hepatic lipids, a portion of the liver tissue was homogenized in saline using a Teflon homogenizer and total lipid was extracted by the method developed by Folch et al. [27]. Total lipids of the liver were determined by a gravimetric method. The hepatic triglyceride contents were measured by an enzymatic method using a commercial serum triglyceride assay kit (Asan Pharmaceutical Co. Korea).
Serum alanine transaminase (ALT) activities were measured spectrophotometrically using a commercially available kit (Youngdong Pharmaceutical Co., Yongin, Korea) in accordance with the manufacturer's instructions. Serum levels of TNF-α, IL-6, and monocyte chemotactic protein-1 (MCP-1) were determined using enzyme-linked immunosorbent assay (ELISA) kits specific for mice (eBioscience, Vienna, Austria).
Hepatic TBARS were determined using the method of Ohkawa et al. [28]. A portion of the liver tissue was homogenized in 5 volumes of 10 mM sodium phosphate buffer (pH 7.4). To 0.5 mL of the homogenate, a solution composed of 15% trichloroacetic acid (TCA), 0.4% thiobarbituric acid (TBA), and 2.5% HCl (1 mL) was added. The reaction mixture was incubated at 100℃ for 45 min, and then cooled on ice. After centrifugation (1,500g for 15 min), the absorbance of the supernatant was measured at 532 nm. TBARS were expressed as nmol malondialdehyde (MDA)/g liver. The glutathione (GSH) level in the liver was quantified by the method of Ellman [29]. A portion of the liver sample was homogenized in 9 volumes of 0.1 mM phosphate buffer (pH 7.4). After centrifugation (10,000g at 4℃ for 30 min), the supernatant (0.5 mL) was mixed with 4.5 mL of 5,5-dithiobis-2-nitrobenzonic acid (DTNB) working solution containing 10 mM DTNB and 0.1 M phosphate buffer (pH 8.0; 1:90, v/v). After incubation at room temperature for 15 min, the absorbance was measured at 534 nm. The protein content was measured using the Bradford method [30]. The level of GSH was expressed as nmol/mg protein.
Body weight, food intake, and feed efficiency ratio (FER) of the ob/ob control mice and the mice supplemented with 1% C. militaris water extract are shown in Table 1. The body weight, weight gain, food intake, and FER of the C. militaris group were not significantly different from those of the control group.
The effects of C. militaris on glycemic control and insulin resistance are shown in Table 2. Serum glucose levels were significantly lower in the C. militaris group than in the control group (P < 0.05). Although insulin levels were not significantly different between the two groups, C. militaris supplementation significantly reduced the HOMA-IR value in comparison with the control group (P < 0.01).
The hepatic total lipid and triglyceride contents of the C. militaris group were reduced by 19.8% and 25.3%, respectively, compared with the control group (P < 0.05; Table 3). Serum FFA levels were lower in the C. militaris group than in the control group (P < 0.05; Table 3). Serum ALT activities were significantly reduced by consumption of C. militaris in comparison with the control group (P < 0.05).
Serum TNF-α levels in the C. militaris group were significantly decreased by 17.1% in comparison with the control group (P < 0.05; Fig. 1). C. militaris supplementation reduced the serum IL-6 by 19.9% in comparison with the control group. Serum MCP-1 levels of the C. militaris group tended to be low in comparison with the control group, although the difference was not significant.
The effects of C. militaris on lipid peroxide and GSH concentrations in the liver are shown in Fig. 2. Consumption of C. militaris decreased hepatic TBARS by 23.4% and increased GSH levels by 18.7% in comparison with the control group (P < 0.05).
We determined the effect of C. militaris supplementation on development of fatty liver, oxidative stress, and inflammatory cytokine levels to evaluate its benefit for NAFLD in ob/ob mice. These mice have a mutation in the ob gene, which encodes leptin, resulting in hyperphagia and obesity [31]. The hepatocytes of these insulin-resistant mice spontaneously become steatotic, making them a valuable tool for studying NAFLD [32].
Supplementation with 1% C. militaris reduced serum glucose and the HOMA-IR, a surrogate parameter of insulin resistance [33] in the ob/ob mice. This finding is in agreement with previous reports. C. militaris extract offered at 1% of the diet improved insulin resistance and hyperglycemia without influencing insulin secretion capacity in 90% pancreatectomized rats [34] and in db/db mice [24].
C. militaris extract reduced serum FFA levels in this study. In addition, it decreased hepatic total lipids and triglyceride contents and serum ALT, suggesting alleviation of fatty liver and improvement of liver function. Insulin resistance increases secretion of FFAs from peripheral adipose tissue due to enhanced lipolysis [35], resulting in elevated FFA uptake by the liver, which in turn is converted into triglycerides [36]. Reportedly, FFAs in the blood are elevated in animal models of NAFLD [37] and in NAFLD patients [36].
The improvement in insulin resistance by C. militaris may be partially related to its effect on proinflammatory cytokines. C. militaris decreased serum TNF-α and IL-6 levels. C. militaris also showed a tendency to decrease serum MCP-1, although the difference was not statistically significant. These findings are in agreement with a previous report that C. militaris reduced TNF-α and IL-6 secretion by LPS-treated murine macrophages [18]. TNF-α mediates insulin resistance by impairing insulin signal transduction [38]. IL-6 affects insulin signaling, leading to development of insulin resistance [39]. MCP-1 contributes to development of the insulin resistance and hepatic steatosis associated with obesity [40]. Thus, the reduction in TNF-α and IL-6 levels induced by C. militaris could contribute to improvement of insulin sensitivity and attenuation of hepatic steatosis.
ob/ob mice with fatty liver have been reported to show increased production of ROS, suggesting increased oxidative stress [41]. Steatotic liver is vulnerable to the 'second hit' mediated by oxidative stress, which leads to inflammation [3,6]. In this study, C. militaris reduced hepatic TBARS, suggesting alleviation of the oxidative stress in ob/ob mice. C. militaris extract was reported to exert a potent antioxidant activity by directly scavenging free radicals [15,16]. In addition, we demonstrated that C. militaris elevated hepatic GSH levels in ob/ob mice. C. militaris extract has been shown to increase GSH levels in t-BHP-treated HepG2 cells [17]. Ramesh et al. reported that cordycepin (3'-deoxyadenosin), one of the major bioactive components of C. militaris, elevated hepatic GSH and reduced TBARS in aged rats, suggesting that cordycepin could be the active component in terms of antioxidant activities [42]. Since nuclear erythroid-related factor 2 (Nrf2) plays a key role in synthesis of GSH [43], further study to investigate the effect of C. militaris and cordycepin on Nrf2-signaling pathway could be needed to elucidate the mechanism underlying the antioxidant activity. GSH is an antioxidant involved in scavenging ROS and removing lipid peroxides [44]. Thus, C. militaris could contribute to amelioration of the progression of NAFLD by decreasing oxidative stress.
In conclusion, C. militaris effectively reduced serum glucose and FFA levels and improved insulin sensitivity in ob/ob mice. C. militaris also reduced hepatic triglyceride accumulation and serum ALT. In addition, C. militaris ameliorated hepatic oxidative stress and reduced serum proinflammatory cytokine levels. Therefore, C. militaris may be beneficial in preventing NAFLD.
Figures and Tables
Fig. 1
Serum levels of TNF-α (A), IL-6 (B), and MCP-1 (C) in ob/ob mice. Values represent mean ± SEM (n = 8). *P < 0.05. nsNot significant.
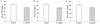
Fig. 2
Hepatic levels of TBARS (A) and GSH (B) in ob/ob mice. Values represent mean ± SEM (n = 8). *P < 0.05.
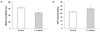
References
1. Sanyal AJ. AGA technical review on nonalcoholic fatty liver disease. Gastroenterology. 2002; 123:1705–1725.


2. Petta S, Muratore C, Craxì A. Non-alcoholic fatty liver disease pathogenesis: the present and the future. Dig Liver Dis. 2009; 41:615–625.


4. Salt WB 2nd. Nonalcoholic fatty liver disease (NAFLD): a comprehensive review. J Insur Med. 2004; 36:27–41.
5. Ahmed MH, Abu EO, Byrne CD. Non-Alcoholic Fatty Liver Disease (NAFLD): new challenge for general practitioners and important burden for health authorities? Prim Care Diabetes. 2010; 4:129–137.


7. Marchesini G, Forlani G. NASH: from liver diseases to metabolic disorders and back to clinical hepatology. Hepatology. 2002; 35:497–499.


8. Tilg H, Moschen AR. Insulin resistance, inflammation, and non-alcoholic fatty liver disease. Trends Endocrinol Metab. 2008; 19:371–379.


9. Lebovics E, Rubin J. Non-alcoholic fatty liver disease (NAFLD): why you should care, when you should worry, what you should do. Diabetes Metab Res Rev. 2011; 27:419–424.


11. Ibrahim MA, Kelleni M, Geddawy A. Nonalcoholic fatty liver disease: current and potential therapies. Life Sci. 2013; 92:114–118.


13. Paterson RR. Cordyceps: a traditional Chinese medicine and another fungal therapeutic biofactory? Phytochemistry. 2008; 69:1469–1495.


14. Ng TB, Wang HX. Pharmacological actions of Cordyceps, a prized folk medicine. J Pharm Pharmacol. 2005; 57:1509–1519.


15. Yu HM, Wang BS, Huang SC, Duh PD. Comparison of protective effects between cultured Cordyceps militaris and natural Cordyceps sinensis against oxidative damage. J Agric Food Chem. 2006; 54:3132–3138.


16. Zhan Y, Dong CH, Yao YJ. Antioxidant activities of aqueous extract from cultivated fruit-bodies of Cordyceps militaris (L.) Link in vitro. J Integr Plant Biol. 2006; 48:1365–1370.


17. Wang BS, Lee CP, Chen ZT, Yu HM, Duh PD. Comparison of the hepatoprotective activity between cultured Cordyceps militaris and natural Cordyceps sinensis. J Funct Foods. 2012; 4:489–495.


18. Jo WS, Choi YJ, Kim HJ, Lee JY, Nam BH, Lee JD, Lee SW, Seo SY, Jeong MH. The anti-inflammatory effects of water extract from Cordyceps militaris in murine macrophage. Mycobiology. 2010; 38:46–51.


19. Anstee QM, Goldin RD. Mouse models in non-alcoholic fatty liver disease and steatohepatitis research. Int J Exp Pathol. 2006; 87:1–16.


20. Weisberg SP, Leibel R, Tortoriello DV. Dietary curcumin significantly improves obesity-associated inflammation and diabetes in mouse models of diabesity. Endocrinology. 2008; 149:3549–3558.


21. Bruno RS, Dugan CE, Smyth JA, DiNatale DA, Koo SI. Green tea extract protects leptin-deficient, spontaneously obese mice from hepatic steatosis and injury. J Nutr. 2008; 138:323–331.


22. Massart J, Robin MA, Noury F, Fautrel A, Lettéron P, Bado A, Eliat PA, Fromenty B. Pentoxifylline aggravates fatty liver in obese and diabetic ob/ob mice by increasing intestinal glucose absorption and activating hepatic lipogenesis. Br J Pharmacol. 2012; 165:1361–1374.


23. Zhang G, Huang Y, Bian Y, Wong JH, Ng TB, Wang H. Hypoglycemic activity of the fungi Cordyceps militaris, Cordyceps sinensis, Tricholoma mongolicum, and Omphalia lapidescens in streptozotocin-induced diabetic rats. Appl Microbiol Biotechnol. 2006; 72:1152–1156.


24. Choi HN, Kang MJ, Jeong SM, Seo MJ, Kang BW, Jeong YK, Kim JI. Effect of Dongchunghacho (Cordyceps militaris) on hyperglycemia and dyslipidemia in type 2 diabetic db/db mice. Food Sci Biotechnol. 2012; 21:1157–1162.


25. Reeves PG, Nielsen FH, Fahey GC Jr. AIN-93 purified diets for laboratory rodents: final report of the American Institute of Nutrition ad hoc writing committee on the reformulation of the AIN-76A rodent diet. J Nutr. 1993; 123:1939–1951.


26. Matthews DR, Hosker JP, Rudenski AS, Naylor BA, Treacher DF, Turner RC. Homeostasis model assessment: insulin resistance and beta-cell function from fasting plasma glucose and insulin concentrations in man. Diabetologia. 1985; 28:412–419.


27. Folch J, Lees M, Sloane Stanley GH. A simple method for the isolation and purification of total lipides from animal tissues. J Biol Chem. 1957; 226:497–509.


28. Ohkawa H, Ohishi N, Yagi K. Assay for lipid peroxides in animal tissues by thiobarbituric acid reaction. Anal Biochem. 1979; 95:351–358.


30. Bradford MM. A rapid and sensitive method for the quantitation of microgram quantities of protein utilizing the principle of protein-dye binding. Anal Biochem. 1976; 72:248–254.


31. Halaas JL, Gajiwala KS, Maffei M, Cohen SL, Chait BT, Rabinowitz D, Lallone RL, Burley SK, Friedman JM. Weight-reducing effects of the plasma protein encoded by the obese gene. Science. 1995; 269:543–546.


32. Koteish A, Mae Diehl A. Animal models of steatohepatitis. Best Pract Res Clin Gastroenterol. 2002; 16:679–690.


33. Muniyappa R, Lee S, Chen H, Quon MJ. Current approaches for assessing insulin sensitivity and resistance in vivo: advantages, limitations, and appropriate usage. Am J Physiol Endocrinol Metab. 2008; 294:E15–E26.


34. Choi SB, Park CH, Choi MK, Jun DW, Park S. Improvement of insulin resistance and insulin secretion by water extracts of Cordyceps militaris, Phellinus linteus, and Paecilomyces tenuipes in 90% pancreatectomized rats. Biosci Biotechnol Biochem. 2004; 68:2257–2264.


35. Jensen MD, Haymond MW, Rizza RA, Cryer PE, Miles JM. Influence of body fat distribution on free fatty acid metabolism in obesity. J Clin Invest. 1989; 83:1168–1173.


36. Donnelly KL, Smith CI, Schwarzenberg SJ, Jessurun J, Boldt MD, Parks EJ. Sources of fatty acids stored in liver and secreted via lipoproteins in patients with nonalcoholic fatty liver disease. J Clin Invest. 2005; 115:1343–1351.


37. Gauthier MS, Couturier K, Latour JG, Lavoie JM. Concurrent exercise prevents high-fat-diet-induced macrovesicular hepatic steatosis. J Appl Physiol (1985). 2003; 94:2127–2134.


38. Hotamisligil GS, Spiegelman BM. Tumor necrosis factor alpha: a key component of the obesity-diabetes link. Diabetes. 1994; 43:1271–1278.


39. Senn JJ, Klover PJ, Nowak IA, Zimmers TA, Koniaris LG, Furlanetto RW, Mooney RA. Suppressor of cytokine signaling-3 (SOCS-3), a potential mediator of interleukin-6-dependent insulin resistance in hepatocytes. J Biol Chem. 2003; 278:13740–13746.


40. Kanda H, Tateya S, Tamori Y, Kotani K, Hiasa K, Kitazawa R, Kitazawa S, Miyachi H, Maeda S, Egashira K, Kasuga M. MCP-1 contributes to macrophage infiltration into adipose tissue, insulin resistance, and hepatic steatosis in obesity. J Clin Invest. 2006; 116:1494–1505.


41. Yang S, Zhu H, Li Y, Lin H, Gabrielson K, Trush MA, Diehl AM. Mitochondrial adaptations to obesity-related oxidant stress. Arch Biochem Biophys. 2000; 378:259–268.


42. Ramesh T, Yoo SK, Kim SW, Hwang SY, Sohn SH, Kim IW, Kim SK. Cordycepin (3'-deoxyadenosine) attenuates age-related oxidative stress and ameliorates antioxidant capacity in rats. Exp Gerontol. 2012; 47:979–987.

